Musculoskeletal Ultrasound Artifacts
Technological Aspects
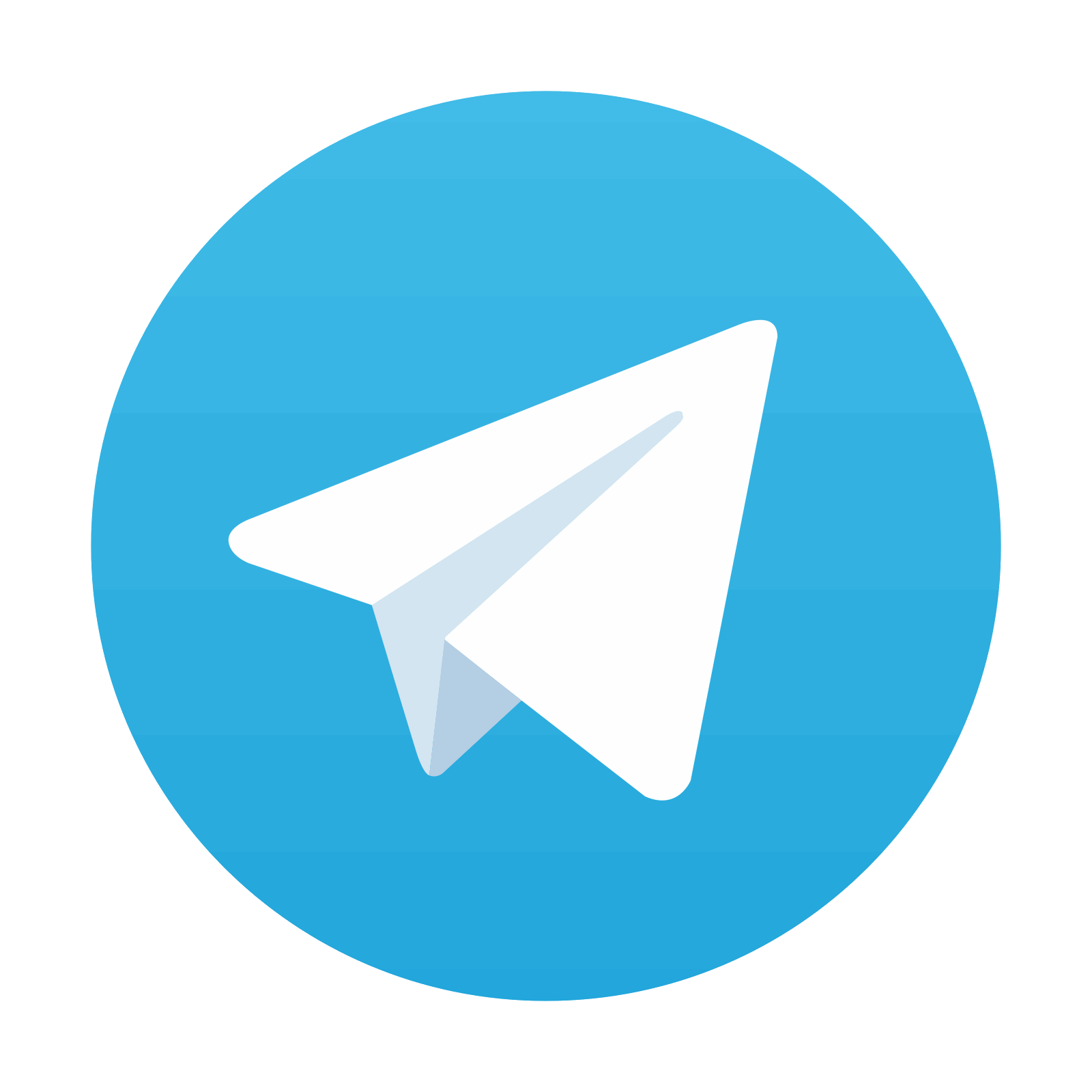
Stay updated, free articles. Join our Telegram channel

Full access? Get Clinical Tree
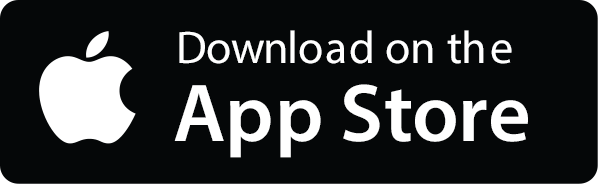
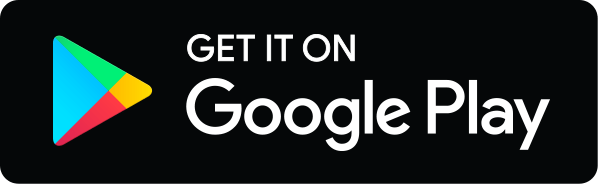
Musculoskeletal Ultrasound Artifacts
Technological Aspects