Fig. 12.1
Representative images of various stages of newly formed atherosclerotic changes within neointima after stent implantation. (a) Foamy macrophage clusters in the peristrut region of sirolimus-eluting stents (SES) implanted for 13 months antemortem. (b) Fibroatheroma with a foamy macrophage-rich lesion and early necrotic core formation in SES of 13 months’ duration. (c) Fibroatheroma with peristrut early necrotic core, cholesterol clefts, surface foamy macrophages, and early calcification (arrows) in SES at 13 months. (d) Peristrut late necrotic core in the neointima characterized by a large aggregate of cholesterol clefts in SES at 17 months. (e) Fibroatheroma with calcification in the necrotic core in SES of 10 months’ duration. (f) Peristrut calcification (arrows) with fibrin in SES of 7 months’ duration. (g, h) A low-power magnification image (h) of a severely narrowed bare-metal stent (BMS) implanted for 61 months with a thin-cap fibroatheroma. Note macrophage infiltration and a discontinuous thin fibrous cap in a high-power magnification image (g). (i) A low-power magnification image shows a plaque rupture with an acute thrombus totally occluding the lumen in a BMS implanted for 61 months antemortem. (j) A high-power magnification image shows a discontinuous thin-cap fibroatheroma with occlusive luminal thrombus (Reprinted with permission from Dr. Nakazawa with permission from the publisher (From Nakazawa et al. [20]))
More aggressive development of neoatherosclerotic changes in DES compared with BMS might be explained by the delayed and incomplete recovery of endothelial cells covering the neointimal surface due to cytotoxic or cytostatic drugs. It is possible that dysfunctioning endothelial cells or a denudated endothelial cell layer over the neointima stimulates the interaction between circulating monocytes and the vascular wall, which then leads to earlier development of unstable atherosclerotic changes in the neointima through cell adhesion and cell migration mechanisms.
One pathological study examined neoatherosclerosis that developed in second-generation DES. Otsuka et al. [21] studied 204 lesions that included 46 everolimus-eluting stents (EES) from 149 autopsy cases. As expected, EES demonstrated greater strut coverage with less inflammation, less fibrin deposition, and less late and very late stent thrombosis compared with the first-generation DES. Nevertheless, the observed frequency of neoatherosclerosis was comparable between these devices, indicating that careful long-term follow-up is still mandatory even after second-generation DES implantation.
Up to this point, there have been no reports on the pathohistological characteristics of neoatherosclerosis in bioabsorbable vascular scaffolds (BVS).
12.4 IVUS and Angioscopic Findings of Neoatherosclerosis
It has been difficult to precisely identify the characteristics of neointimal tissue using intravascular ultrasound (IVUS) because of this technique’s relatively low spatial resolution. However, several studies have used gray-scale IVUS to evaluate the development of atherosclerotic changes within the neointima [22, 23]. In particular, Hakim et al. [23] studied 47 patients (54 lesions) who received serial IVUS examinations for more than 1.5 years after coronary stenting. Calcified neointima was observed in 20 % (12 lesions) of patients during follow-up, but the possibility of lipid accumulation was not assessed in this study. Researchers have begun to evaluate changes in neointimal tissue characteristics using new-generation IVUS with spectral analysis of ultrasound intensity and frequency [24–26]. Kang et al. [24] examined tissue characteristics of restenotic in-stent neointima after DES and BMS using virtual histology intravascular ultrasound (VH-IVUS) in 117 lesions (70 DES and 47 BMS). During mean follow-up durations of 43.5 months for BMS and 11.1 months for DES, they observed time-dependent development and progression of both necrotic cores and dense calcium deposits within the neointima in both stent types.
Coronary angioscopy is able to differentiate neointimal characteristics by semi-quantitatively grading yellow color tones. A recent study involving serial angioscopic examinations of 26 patients [27] showed that the incidence of yellow plaque and thrombus in BMS increased from the first (6–12 months) to second (>4 years) follow-up visits (58 and 31 %, P < 0.05). In addition, all of the yellow plaques at the second follow-up visit protruded from the vessel wall into the lumen. Higo et al. [28] used angioscopy to compare BMS with 57 cases of SES in terms of changes in neointimal characteristics. SES promoted more frequent formation of atherosclerotic yellow neointima in stent-implanted lesions at 10-month follow-up compared with BMS. In addition, intraluminal thrombus was detected more often on yellow areas than on white ones, and was never detected where a stent was buried under white neointima. It is possible that the increased risk of late stent thrombosis in DES lesions may be due to newly formed yellow neointima, which is indicative of cholesterol accumulation.
12.5 OCT Findings of Neoatherosclerosis
Intravascular OCT is a newly developed intravascular imaging modality with a maximum spatial resolution of 10 μm, which is approximately ten times higher than that of conventional IVUS imaging [29–31]. In addition to plaque characterization of native coronary arteries [32, 33], OCT provides detailed morphological information about neointimal tissue within coronary stents [34–36]. Indeed, innovations in the application of OCT to coronary imaging have promoted our understanding of neoatherosclerosis after coronary stent implantation.
Morphological characteristics of the neointima within 1 year after stent implantation can be categorized into three patterns based on OCT appearance [37]. Figure 12.2 shows representative pictures. The homogeneous pattern is characterized by a uniformly signal-rich and low-attenuation appearance. This OCT feature is predominantly observed in early-stage BMS neointima, and represents stable fibrous tissue. The layered pattern has a signal-poor appearance with a high-signal band adjacent to the luminal surface. The heterogeneous pattern is mainly signal-poor with islands of various signal intensities. In some cases there were mixed neointimal patterns. The latter two patterns are frequently observed in DES neointima, and represent the deposition of fibrin, platelets, or extracellular matrix proteoglycans during the acute phase of implantation. Accordingly, such OCT pattern observed within early phase of implantation should be distinguished from neoatherosclerosis developing in the chronic phase. Figure 12.3 depicts representative findings of a variety of neoatherosclerotic changes within the neointima of either BMS or DES.
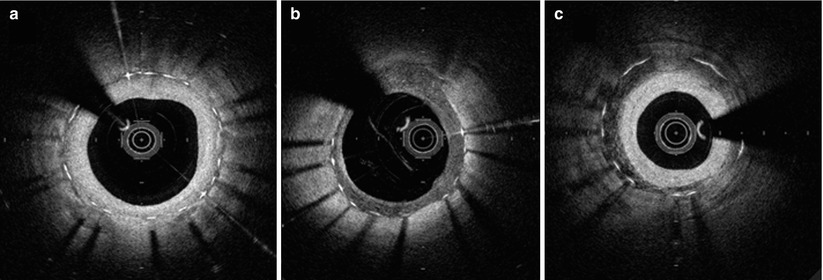
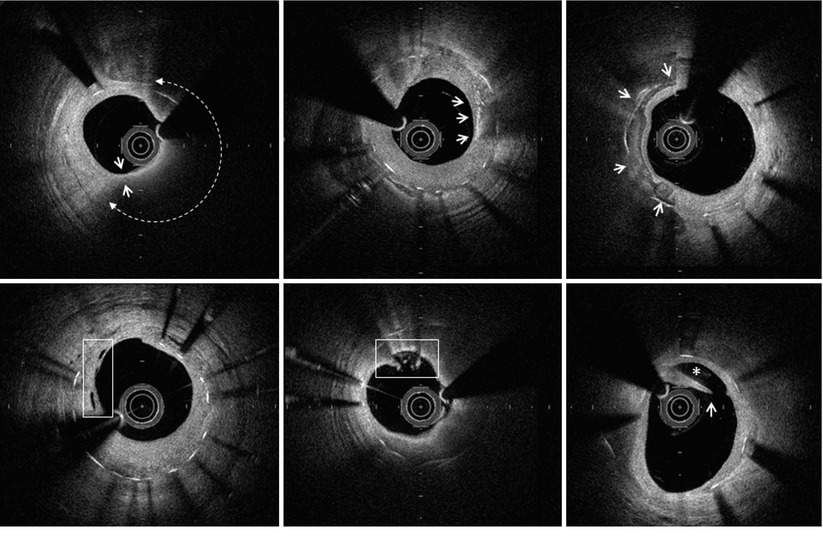
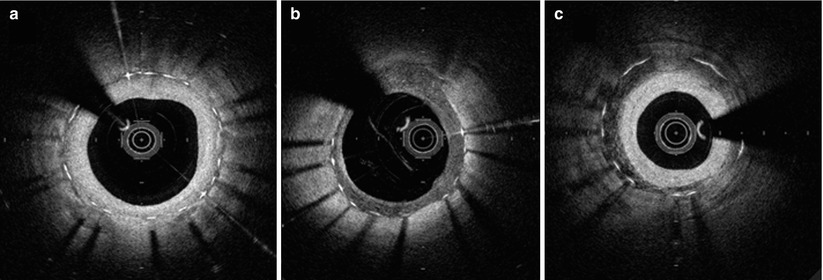
Fig. 12.2
OCT characteristics of neointimal tissue observed in the early post-implantation stage (<1 year). (a) Homogenous, (b) heterogenous, and (c) layered patterns. (b) and (c) are more frequently observed in DES than BMS
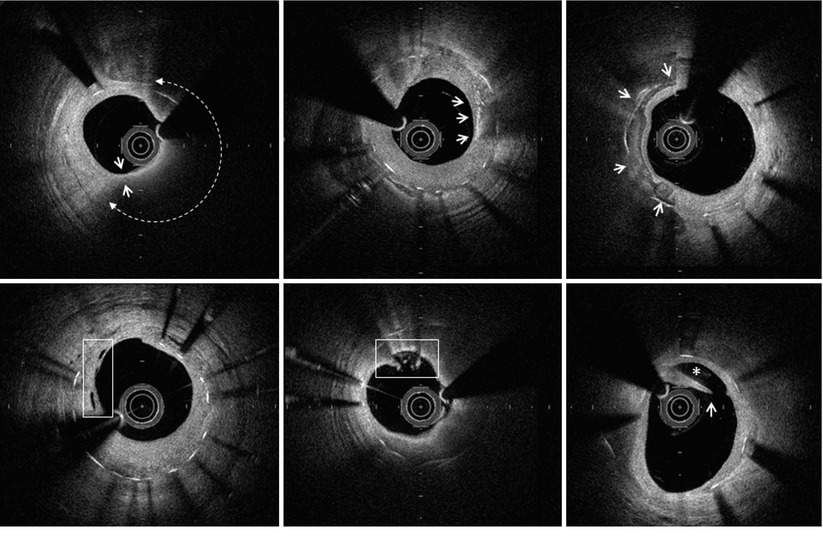
Fig. 12.3
OCT characteristics of neoatherosclerosis observed in the intermediate and late post-implantation phases. (a) Lipid-laden neointima showing a diffusely bordered, signal-poor region with overlying signal-rich homogeneous band (bidirectional arc). The minimal thickness of the fibrous cap is 60 m (between the two white arrows). (b) Macrophage infiltration with high signal accumulation accompanied by heterogeneous back shadows (white arrows). (c) Calcium deposition within the stented segment showing a sharply delineated region with a mixture of signal-poor and signal-rich areas (white arrows). (d) Neovascularization showing small vesicular or tubular structures of 50- to 300 µm diameters (rectangular box). (e) Neointimal laceration showing irregularities or disruption of the superficial intimal lining without fibrous cap rupture (rectangular box). (f) Intra-stent plaque rupture showing disrupted fibrous membrane (white arrow) with an underlying empty cavity
12.5.1 BMS
Using intravascular OCT, Takano et al. [11] for the first time demonstrated that fibrous neointima could transform into lipid-laden neointima in the chronic phase after BMS implantation. In their study, 67 % of BMS demonstrated conversion to lipid-laden neointima after 5 years of implantation, and plaque angiogenesis (neovascularization) was also observed significantly more frequently in the late phase (>5 years) than in the early phase (<1 year). Remarkably, neoatherosclerotic changes were observed in the BMS without angiographic restenosis. They also found disruption of the fibrous cap and thrombus formation associated with neoatherosclerotic changes, suggesting a potential role of neoatherosclerosis in late stent failure. In selected cases with restenosis, Habara et al. [38] compared the morphological characteristics of neointima either very late (>5 years) or early after BMS implantation. Almost 90 % of the BMS restenotic lesions that occurred in the very late phase were accompanied by neoatherosclerotic changes, and peri-stent and intra-neointimal microvessels were more frequently observed in in-stent restenosis occurring in the very late phase.
12.5.2 DES
Neoatherosclerosis occurs more evidently in DES compared with BMS. Kang et al. [39] showed that of 50 restenotic lesions in DES that were on average 32 months old, 90 % of neointima contained lipid accumulation, and 10 % demonstrated calcium deposits. In addition, 52 % showed in-stent thin-cap fibroatheroma (TCFA) and 29 (58 %) had at least one in-stent neointimal rupture. Patients presenting with unstable angina showed a significantly thinner fibrous cap (55 m vs 100 m, P < 0.006) and higher incidence of TCFA (75 % vs 37 %, P < 0.008), intimal rupture (75 % vs 47 %, P < 0.044), and intraluminal thrombus (80 % vs 43 %, P < 0.010) than stable patients. Interestingly, fibrous cap thickness negatively correlated with follow-up time (r = −0.318, P < 0.024) after stent implantation. Compared with DES implanted more recently than 20 months, those placed at least 20 months prior to the study had a higher incidence of TCFA (69 % vs 33 %, P < 0.012) and red thrombus (27 % vs 0 %, P < 0.007). Patients with unstable angina demonstrated an increasing number of unstable findings on OCT, including TCFA, neointima rupture, and thrombus. The authors showed that in-stent neoatherosclerosis possibly correlates with DES failure, especially late after implantation.
12.5.3 BMS vs DES
As previously mentioned, there are substantial differences between BMS and DES in terms of the incidence and time course of neoatherosclerosis development. Yonetsu et al. [40] recently used OCT to compare the characteristics of neoatherosclerosis in 56 BMS and 82 DES cases. They categorized 138 stents with neointima (>100 μm) based on time since implantation: early phase (<9 months), intermediate phase (9–48 months), and delayed phase (>48 months) [40]. In the early phase, a greater incidence of lipid-laden plaque (37 % vs 8 %, p < 0.02) was found in DES than in BMS. This relation was also evident in the intermediate phase. In the delayed phase, however, incidence of neoatherosclerosis was similar between the two stent types in total. A similar pattern was observed regarding the incidence of lipid-rich plaque (LRP), defined as a plaque with a lipid arc >90° (Fig.12.4). These findings support previous pathological data showing earlier development of neoatherosclerosis in DES than in BMS [20]. In addition, in both stent types the incidence of lipid-laden plaque, intra-intimal neovascularization, and TCFA were significantly greater in cases with symptomatic restenosis than in those with asymptomatic restenosis. Thus, lipid-rich neoatherosclerosis develops inside stents earlier in DES than in BMS. After 48 months, most restenotic stents will have developed lipid-laden neointima in both groups. The same group [41] demonstrated that cutoff durations for the development of neoatherosclerosis in DES and BMS were 14 and 55 months, respectively.
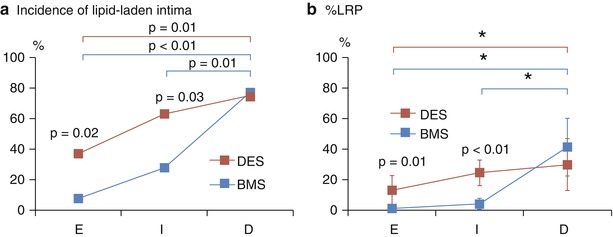
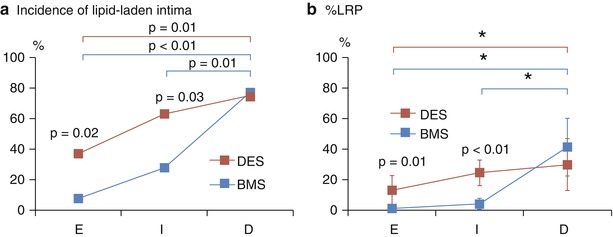
Fig. 12.4
Oct comparison of different neoatherosclerosis parameters between DES and BMS over time. (a) Incidence of lipid-laden plaque and (b) % lipid-rich plaque (LRP). LRP is defined as a plaque with a lipid arc >90°, and %LRP is calculated as the number of cross-sections with LRP divided by the total number of analyzed cross-sections of plaque × 100. *Significant difference using Duncan’s multiple range post hoc test at a 5 % significance level. E early phase (<9 months), I intermediate phase (>9 to <48 months), D delayed phase (>48 months) (Reprinted with permission from the publisher (FromYonetsu et al. [40]))
12.5.4 OCT-Derived Insight into the Pathogenesis of Neoatherosclerosis
The precise mechanism of neoatherosclerosis development remains unknown, but recent OCT studies have provided important clues in this regard.
Tian et al. [42] studied the spatial distribution of neoatherosclerotic changes and evaluated the relation between neoatherosclerosis and tissue characteristics around the stent edge reference segment. In their study, neoatherosclerosis was (1) more frequent in the proximal and distal sections than in the mid-sections of stents, (2) more frequently observed in stents with neointimal formation of microvessels, and (3) significantly more frequent when the adjacent plaque in the edge reference segment was rich in lipid content. These findings suggest that introduction of microvessels into neointima plays an important role in the formation of neoatherosclerosis, and that lipid-rich segments adjacent to stent edges are important in the formation of microvessels that supply blood during neointimal and neoatherosclerotic development.
Furthermore, a recent study by Vergallo et al. showed a positive correlation between the degree of neointimal hyperplasia and the presence of neointimal lipid accumulation [43]. They observed that stents of both types with greater neointimal thickness had a significantly greater prevalence of lipid-laden neointima, but the time course of neoatherosclerotic changes were different between BMS and DES (Fig. 12.5). Additionally, in DES, the magnitude of lipid accumulation was positively related to the degree of neointimal hypertrophy. This association was independent of stent type and time since implantation, and suggests a possible pathogenic link between the two processes. These results agree with data from a previous OCT study, in which neoatherosclerosis was more frequently identified in patients with restenosis than without restenosis [27]. Neointimal hypertrophy and neoatherosclerosis might share similar underlying mechanisms involving chronic inflammation and endothelial dysfunction, as suggested by the involvement of proinflammatory cytokines and the impairment of the nitric oxide pathway. In addition, the extensive neointimal hypertrophy that typically develops inside BMS likely maintains and amplifies flow disturbances inside the stent (restenosis-induced low endothelial shear stress: ESS), favoring further neointimal proliferation and ultimately resulting in neoatherosclerotic degeneration. This “ESS hypothesis” could explain the late occurrence of neoatherosclerosis and its preferential edge location observed in BMS. In contrast, incompetent and incomplete endothelialization after DES implantation induces an inflammatory response stimulating neointimal growth and lipid deposition into the subendothelial space, causing earlier development of neoatherosclerosis compared with BMS.
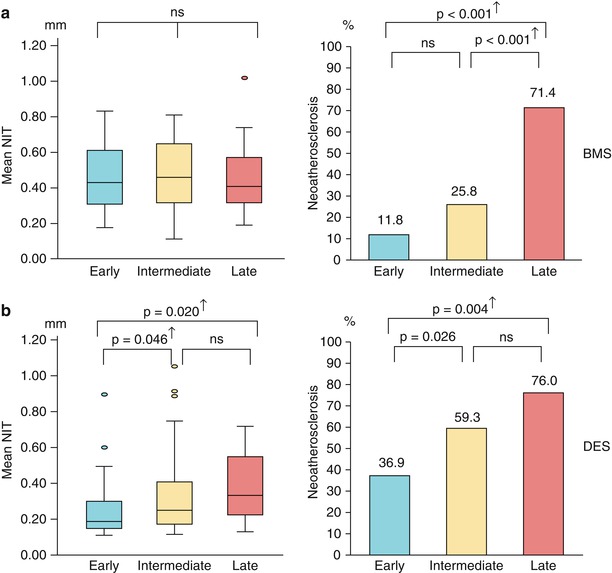
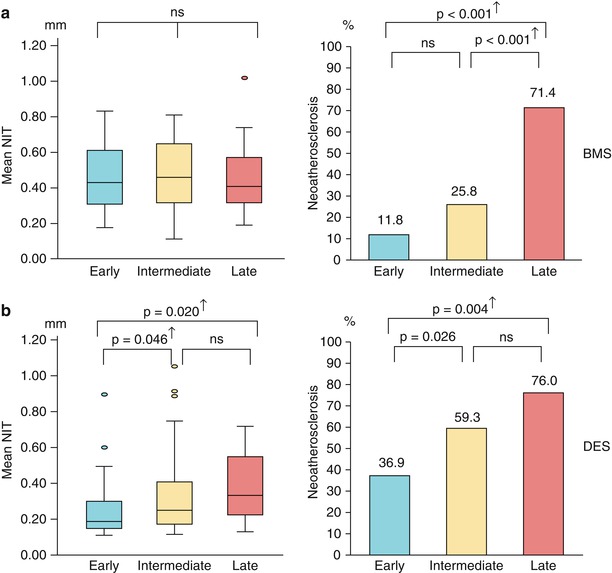
Fig. 12.5
Time course and relation between neointimal hyperplasia and neoatherosclerosis. (a) In the BMS group, there is no difference in mean NIT among stents in different phases. However, the prevalence of NA differs among stents in different phases, with significantly higher values in stents in the late phase compared with those in the early and intermediate phases. (b) In the DES group, mean NIT and prevalence of NA are progressively greater across the three groups. † Indicates significance after adjustment with Bonferroni’s correction (Reprinted with permission from the publisher (From Vergallo et al. [43]))
12.5.5 Risk Factors for Neoatherosclerosis
The predictive factors for the development of neoatherosclerosis have not been established. Yonetsu et al. [41] examined 179 stents in 151 patients and evaluated the influence of patient characteristics, stent type, and time since stent implantation (stent age) on the development of lipid-laden neointima or calcification inside the stents (Table 12.1). In multivariate analysis, stent age ≥48 months, all subtypes of DES, current smoking, chronic kidney disease, and use of angiotensin-converting enzyme inhibitors (ACE-I) or angiotensin II receptor blockade (ARB) remained independent predictors for neoatherosclerosis. Because current smoking and chronic kidney disease are established risk factors for native coronary atherosclerosis, a similar mechanism is likely to be involved in neoatherosclerosis formation. In addition, these results may support the importance of secondary prevention after stent implantation regardless of stent type. It is interesting that ACE-I and ARB were protectively correlated with neoatherosclerosis. These drugs are widely used to reduce cardiovascular events in the secondary prevention of coronary heart disease [44, 45]. Moreover, it has been reported that pharmacologic therapy with ACE-I or ARB reduces neointima proliferation and restenosis after stent implantation [46, 47]. Prospective studies to evaluate the effects of ACE-I and ARB on the prevention of neoatherosclerosis are warranted.
Table 12.1
Predictors for neoatherosclerosis
Final multivariate model (n = 179) | |||
---|---|---|---|
Adjusted odds ratio
![]() Stay updated, free articles. Join our Telegram channel![]() Full access? Get Clinical Tree![]() ![]() ![]() |