(1)
Department of Clinical Radiology, Amiri Hospital – Kuwait City, Kuwait City, Kuwait
2.2.3 CADASIL (Cerebral Autosomal Dominant Arteriopathy with Subcortical Infarcts and Leukodystrophy)
2.2.7 Locked-In Syndrome
2.3.1 Epidural Hematoma
2.3.2 Subdural Hematoma
2.3.3 Subarachnoid Hemorrhage
2.4 Meningitis
2.5 Encephalitis
2.5.1 Limbic Encephalitis
2.5.3 Hashimoto’s Encephalitis
2.5.5 Measles Encephalitis
2.5.7 Japanese Encephalitis
2.5.8 West Nile Encephalitis
2.5.10 Murray Valley Encephalitis
2.5.11 St. Louis Encephalitis
2.5.12 Encephalitis Lethargica
2.6 Epilepsy
2.7 Headache
2.7.1 Migraine
2.8.1 Multiple Sclerosis
2.8.3 Marburg’s Type MS
2.8.6 Susac’s Syndrome
2.8.7 Guillain–Barré Syndrome
2.9 Parkinsonism
2.10 Dementia
2.10.1 Alzheimer’s Disease
2.10.2 Vascular Dementia
2.10.4 Dementia with Lewy Bodies
2.10.8 Prion Disease
2.11 Huntington’s Disease
2.13 Aphasia
2.13.1 Neural Control of Speech
2.14 Squint (Strabismus)
2.14.2 Pathophysiology
2.14.3 Related Disorders
2.15 Nystagmus
2.15.2 Nystagmus Subtypes
2.16 Erectile Dysfunction
2.16.2 Pathophysiology
2.1 Stroke (Brain Infarction)
Stroke means the death of brain cells (infarction) due to ischemia or emboli.
The most common causes of stroke are atherosclerosis, embolic vascular occlusion, hypertension, and inflammatory vascular diseases (vasculitis). Patients present with sudden neurological deficits in the body according to the area of the brain affected. Up to 75 % of all cerebral infarctions occur due to middle cerebral artery occlusion. Occlusion of the posterior inferior cerebellar artery (PICA) causes infarction of the lateral medulla plus the inferior cerebellar peduncles (Wallenberg’s syndrome).
Imaging strokes involves the assessment of four Ps:
Parenchyma: assess the area of stroke and excludes hemorrhage (checked by unenhanced CT).
Pipes: assess the extra- and intracerebral blood vessels (carotid and vertebral arteries). Scanning for CTA should start from the head to the aortic arch.
Perfusion: assess cerebral blood volume (CBV), cerebral blood flow (CBF), and mean transit time (MTT).
Penumbra: the concept of penumbra in stroke refers to the salvageable brain tissue. When a vascular insult occurs, the infarcted tissue is surrounded by a region of stunned tissue due to reduction of the blood flow within the affected region. The identification of the penumbra helps the decision of using thrombolytics in acute stroke cases. On CT, the penumbra is assessed by showing parameters’ mismatch, while on MR, it is assessed by showing diffusion/perfusion mismatch. Penumbra = MTT minus CBV.
Thrombolytics are not given to stroke patients beyond 3 h from the start of the symptoms due to the risk of hemorrhage. Hemorrhage is an absolute contraindication for thrombolytic therapy. Stroke is evaluated on unenhanced CT, CT angiography, and CT perfusion study.
Hemorrhagic infarction is usually caused by hypertension or embolic occlusion. Hemorrhagic infarctions arise due to two mechanisms:
Venous thrombosis: the high flowing arterial blood is obstructed by a blocked vein, which raises the intracapillary pressure causing them to rupture and bleed.
Arterial embolism: the embolus blocks the artery, and in some times a small hole develops within the embolus making blood gush into the capillaries with high speed and pressure, causing them to rupture and bleed.
Lacunar infarctions (cerebral microangiopathy) are infarctions less than 1 cm in size and occur due to occlusion of the penetrating arterioles of the brain parenchyma. Usually, they are seen in the basal ganglia, the thalamus, and the internal capsule. Lacunar infarctions are commonly seen in diabetic patients.
Differential Diagnoses and Related Diseases
Pusher syndrome is a very specific disease of postural orientation, commonly affecting poststroke hemiparetic patients. In the pusher syndrome, patients use their nonparetic arm and/or leg to actively push from the nonparalyzed side toward the paralyzed, which results in loss of balance and falling toward the paralyzed side (. Fig. 2.1.1). These patients also resist any attempt to correct their tilted body posture toward the vertical upright position. Pusher syndrome can be seen in up to 10 % of patients with hemiparesis due to strokes. Pusher syndrome may be also arising due to brain trauma or tumors.
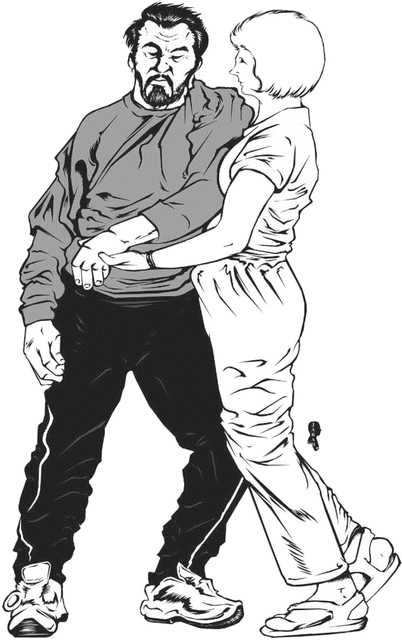
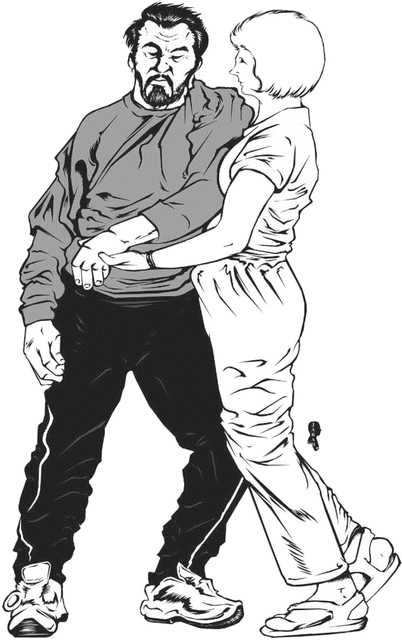
Fig. 2.1.1
An illustration demonstrates pusher syndrome; the patient is actively pushing and extending his right side (nonparalytic side) toward the left side (paralytic side), which is assisted by the nurse
Signs on CT
Hyperacute stage (the first 3–6 h): unenhanced CT usually is normal. It must be repeated after this period within 24–48 h.
Acute stage (from 6 to 24 h): nonenhanced CT shows a wedge-shaped hypodense area surrounded by edema that may cause mass effect on the ventricles with effacement of the cerebral sulci (. Fig. 2.1.2a). The hypodense lesion follows a vascular territory (. Fig. 2.1.3). Cytotoxic edema starts after 30 min from the stroke attack, and vasogenic edema starts from 4 to 6 h postattack. Each increase in 1 % of parenchymal edema reduces the Hounsfield unit (HU) by 2.5 HU.
Fig. 2.1.2
Multiple axial CT of the brain with different stages of infarction: (a) acute infarction, (b) subacute infarction, (c) chronic infarction with gliosis, and (d) chronic infarction with formation of porencephalic cyst (arrowhead)
Fig. 2.1.3
Sequential axial brain MR illustrations demonstrate different vascular territories of the brain parenchyma
Subacute stage (days to weeks): there is a hypodense lesion without edema (. Fig. 2.1.2b). Edema resolves and the mass effect decreases at 7–10 days postattack.
Chronic stage (more than 3 months postattack): the tissues around the lesion will lose their volume (gliosis), which will cause negative pressure upon the adjacent ventricles, causing their dilatation (evacuee dilatation) (. Fig. 2.1.2c). When the evacuee dilatation is massive, the negative pressure causes the ventricle to open into the infarction, creating a porencephalic cyst (. Fig. 2.1.2d). Porencephalic cyst is a cerebrospinal fluid cyst that is communicating with the ventricles.
Hyperdense vessel sign: on nonenhanced CT, a thrombosed vessel may appear as a hyperdense structure due to the thrombus within it. Normal blood measures 40–60 HU and is normally not seen on nonenhanced CT, while thrombosed blood measures 77–80 HU and can appear on nonenhanced CT. The thrombosed blood vessels are usually asymmetric, and bilateral symmetrical hyperdense vessel is unlikely to be thrombosis.
Obscuration of the lentiform nucleus: hypoattenuation and obscuration of the lentiform nucleus due to cytotoxic edema are another signs of acute infarction.
Insular ribbon sign: it refers to hypoattenuation of the insular region with loss of the gray–white matter definition.
HU window alteration: the standard HU window setting is 80 HU widths and 20 HU center. If no abnormality in attenuation is seen in the image, lower the window to 8 HU widths and 32 HU center. The last settings increase the sensitivity for detection of hypodense areas.
Luxury perfusion: When you inject contrast into an acute infarction, you’ll get contrast diffusion as multiple lines into the gyri (. Fig. 2.1.4). This sign appears within the first 3 days of the attack. It is best recalled by Elster’s rule of 3 (as early as 3 days, maximum at 3 days to 3 weeks and gone by 3 months).
Fig. 2.1.4
Axial brain CT illustration demonstrates infarction within the vascular region of the middle cerebral artery with multiple enhanced lines inside it representing luxury perfusion
Hemorrhagic infarction is seen as an area of hyperdense blood within the brain parenchyma surrounded by hypodense area of cytotoxic edema.
Lacunar infarction is seen as a small (<2 cm), hypodense area within the brain parenchyma with no mass effect (. Fig. 2.1.5).
Fig. 2.1.5
Axial brain CT image shows acute lacunar infarction in the right centrum semiovale with small ring of cytotoxic edema around it (arrowhead)
Disruption of the normal circle of Willis branches is classically detected in stroke (. Fig. 2.1.6).
Fig. 2.1.6
Axial brain CTA image of a patient with left brain infarction shows blockage of the left middle cerebral artery (M1) segment (arrowhead)
Signs on MRI and DWI
Hyperattenuated vessel sign: it is seen on T2* images as hyperintense vessel (like on unenhanced CT images).
T2* images: they are very sensitive to detect hemorrhage and microhemorrhages. Hemorrhage is seen as areas of abnormal blooming, while hemosiderin is seen as areas of low signal intensities.
DWI: areas of infarction are seen as areas of high signal intensity due to water motion impedance (cytotoxic edema), usually 30 min from the start of the attack (. Fig. 2.1.7). On the ADC map, the areas of high signal intensity on DWI show low signal intensity. Chronic infarction shows low signal intensity on DWI and high signal intensity on ACD maps. The two must be assessed together to diagnose chronic infarction. DWI is accurate in detecting brain stem and lacunar infarctions.
Fig. 2.1.7
Axial brain DWI shows acute lacunar infarction in the left paraventricular area (arrowhead)
MR spectroscopy in infarction shows high lactate and low N-acetyl aspartate, choline, and creatine (present up to 5 weeks postattack).
Further Reading
de Lucas EM, et al. CT protocol for acute stroke: tips and tricks for general radiologists. Radiographics. 2008;28:1673–87.
Johannsen L, et al. “Pusher syndrome” following cortical lesions that spare the thalamus. J Neurol. 2006;253:455–63.
Karnath H-O. Pusher syndrome – a frequent but little-known disturbance of body orientation reception. J Neurol. 2007;254:414–24.
Mullins ME. The hyperdense cerebral artery sign on CT scan. Semin Ultrasound CT MR. 2005;26:394–403.
Shetty SK, et al. CT perfusion in acute stroke. Neuroimaging Clin N Am. 2005;15:481–501.
Srinivasan A, et al. State-of-the-art imaging of acute stroke. RadioGraph. 2006;26:S75–95.
Vu D, et al. Non-contrast CT in acute stroke. Semin Ultrasound CT MR. 2005;26:380–6.
2.2 Stroke Diseases and Syndromes
Stroke can be a symptom rather than an actual disease. Some systemic diseases present in the form of stroke. Other diseases or syndromes are associated with stroke as one of their diagnostic criteria. Some syndromes or diseases arise due to stroke in a certain area of the brain. This topic discusses some of the known and uncommon causes, syndromes, and diseases of stroke.
Moyamoya Disease (Progressive Occlusive Arteritis)
Moyamoya disease is characterized by a progressive occlusion of arteries of the circle of Willis due to intimal wall thickening of the distal internal carotid artery and its proximal anterior cerebral artery branch bilaterally, with the formation of abnormal collateral networks that develop adjacent to the stenotic vessel. These collaterals give the shape of a puff of smoke, which is called “moyamoya” in Japanese.
Moyamoya disease is a rare disease worldwide but with high incidence in Japanese. Occlusion usually occurs in both hemispheres, but unilateral occlusion can occur. The disease can be seen in association with sickle cell disease and neurofibromatosis.
The disease peaks in the first decade and dips in the fourth decade. Patients present in young age with recurrent strokes, headaches, and behavioral disturbance. The disease can be suspected in a young adult presenting with stroke, with no predisposing factors. Diagnosis is essentially established by angiography or MR angiography.
Signs on CT, MRI, and MR Angiography
Large network of collaterals in the basal ganglia and brain stem fed by the internal carotid artery, the basilar artery, and the anterior cerebral artery giving the appearance of a puff of smoke (pathognomonic) (. Fig. 2.2.1).
Fig. 2.2.1
MR angiography of a patient with moyamoya disease (a) and MR angiography in a normal healthy patient (b) for comparison. In (a), there is irregular arterial collateral formation in the region of the anterior cerebral arteries bilaterally in figure (a) (arrowheads), with complete disappearance of the middle cerebral artery (MCA) (M1 segment). Compare the image in (a) with the normal MRI appearance of the circle of Willis in (b)
On T1W images, there are multiple hypointense, flow void lesions located in the basal ganglia representing the abnormal collateral networks (. Fig. 2.2.2).
Fig. 2.2.2
Axial T1W brain MR illustration (a) with axial FLAIR MRI (b) of a patient with moyamoya disease shows multiple flow void signal intensities in the region of the basal ganglia bilaterally representing the abnormal collateral formation (arrowheads). Leukoencephalopathy at the region of the posterior horns of the lateral ventricles (b) can be seen (arrow) affecting the right side more than the left side
Cerebral Amyloid Angiopathy
Cerebral amyloid angiopathy (CAA) is a disease that occurs due to deposition of a protein (AB peptide) or amyloid substance in the arteriolar wall of the cerebral vessels, causing weakening and fragility of blood vessel walls. Later, intracerebral bleeding develops due to spontaneous blood vessel rupture.
In CAA, amyloid proteins replace the contractile element of the arteriolar muscle layer, leading to increased fragility of the walls. CAA is not a part of systemic amyloidosis, and it is the most common cause of spontaneous, nontraumatic intracranial bleeding in nonhypertensive elderly patients.
CAA should be suspected when an elderly patient (60 years) presents with unexplained spontaneous intracranial bleeding that is lobar and located very superficial in the cortex. It is responsible for up to 20 % of nontraumatic brain hemorrhage and hemorrhagic infarction and up to 30 % of lobar bleeding.
CAA can be associated with Alzheimer’s disease, with dementia occurring in up to 40 % of cases. Dementia in CAA patients occurs and progresses much faster than dementia in patients with Alzheimer’s disease. Diagnosis of CAA is usually done by clinical history and the radiological features of the CT or the MRI.
Signs on CT and MRI
Acute intracranial bleeding area (lobar hemorrhage) is seen as high-density area on CT images or with high T1/T2 signal intensities on MRI that is located in the superficial cortex and the subcortical white matter (. Figs. 2.2.3 and 2.2.4), with a distribution different from those resulting from hypertensive bleeding (usually deep within the brain). Bleeding due to CAA is characteristically multiple, spares the basal ganglia and brain stem, and is located at the corticomedullary junction.
Fig. 2.2.3
Axial T1W brain MR illustration demonstrates cerebral amyloid angiopathy (CAA). There is corticomedullary bleeding in the left parieto-occipital area surrounded by cytotoxic edema (arrowhead). Multiple areas of hypointense signal intensities scattered within the white matter representing chronic microhemorrhages. An area of low signal intensity is seen in the right parieto-occipital area representing gliosis (arrow)
Fig. 2.2.4
Axial brain nonenhanced CT in a patient with intracranial bleeding due to CAA shows an area of bleeding in the right temporo-occipital region involving the cortices and the corticomedullary junction with a rim of cytotoxic edema (arrowhead)
On MRI, multiple chronic microhemorrhages are seen as signal void, a few millimeters in size hypointense lesions in the deep white and the subcortical white matter on T2* images (. Fig. 2.2.3).
CAA affects commonly the frontal and the parietal lobes. Up to 10–50 % of cases bleed in more than one lobe.
CADASIL (Cerebral Autosomal Dominant Arteriopathy with Subcortical Infarcts and Leukodystrophy)
CADASIL is the most common form of familial strokes with progressive dementia due to vasculopathy of the deep perforating arteries of the cerebral white matter. Patient with CADASIL presents with multiple attacks of strokes beginning usually between 40 and 60 years of life. Patients typically have no risk factors for stroke. Psychiatric symptoms occur in 30 % of cases (e.g., depression).
Diagnostic Criteria for CADASIL
Young age (<50 years)
Two of the following clinical findings: stroke-like episodes with permanent neurological defects, migrainous headache, major mood disturbance, or subcortical dementia
No risk factors for stroke
Positive family history for such stroke attacks
On MRI, white matter changes without cortical infarcts
Signs on MRI
Extensive white matter lesions and basal ganglia abnormalities in the absence of cortical infarcts typically seen in the temporal lobes and the extreme and external capsule (. Fig. 2.2.5). The lesions do not enhance after contrast injection.
Fig. 2.2.5
Axial nonenhanced brain CT (a) and axial FLAIR MRI (b) of a patient with cerebral autosomal dominant arteriopathy with subcortical infarcts and leukodystrophy (CADASIL) show bilateral diffuse white matter lesions with affection of the right external capsule (arrowheads). Pineal calcification can be seen as a secondary finding (arrow)
Widening of the periventricular spaces (Virchow–Robin spaces) (. Fig. 2.2.6).
Fig. 2.2.6
Axial T1W (a) and FLAIR (b) brain MRI of another patient with CADASIL shows bilateral diffuse white matter lesions (leukoencephalopathy) with dilated right Virchow–Robin space (arrowhead)
MR angiography is typically normal (no vascular lesion).
MELAS (Mitochondrial Myopathy, Encephalopathy, Lactic Acidosis, and Stroke-Like Episodes)
MELAS is a mitochondrial disease characterized by episodes of infarction-like cerebral injuries causing hemiparesis or hemiplegia, hearing loss, or cortical blindness. The disease is characterized by elevated serum levels of lactic acid (lactic acidosis).
MELAS starts during early infancy or between the ages of 2 and 5 years. The child presents with vomiting, seizures, failure to thrive, and infarction-like injuries that can occur anywhere in the brain. Interestingly, angiography reveals no vascular occlusion or vascular disease. The infarction-like episodes are due to abnormal physiology rather than abnormal anatomy or vascular occlusion.
Signs on CT and MRI
There are hypodense, asymmetric areas on CT located mainly in the occipitotemporal areas representing infarction that does not follow a vascular territory (. Fig. 2.2.7). The same area shows low T1/high T2 signal intensities. Diffuse brain atrophy can be seen.
Fig. 2.2.7
Axial nonenhanced brain CT illustration (a) and image (b) of a patient with mitochondrial myopathy, encephalopathy, lactic acidosis, and stroke-like episodes (MELAS) demonstrate the radiological features of MELAS. In (a), there is a hypodense lesion in the right temporoparietal region that does not follow a vascular territory (arrows) with bilateral basal ganglia calcifications, as typical signs of MELAS. In (b), a young patient diagnosed with MELAS shows hypodense lesions in the right temporal area representing infarction (arrowhead)
On CT, bilateral basal ganglia calcification may be seen due to old infarctions (. Fig. 2.2.7).
MR angiography is typically normal (excluding vasculitis or moyamoya).
High lactic acidosis peak in MR spectroscopy.
Cortical Laminar Necrosis
Cortical laminar necrosis (CLN) is a disease characterized by destruction of different layers of the cerebral cortex, most prominently the third cortical layer.
On histopathological examination, CLN shows brain infarction of the cortical neuronal elements and the blood vessels (pan-necrosis) without hemorrhage or calcification. CLN has been reported in patients with Reye’s syndrome.
Reye’s syndrome is a rare condition characterized by acute noninflammatory encephalopathy and fatty degeneration of the viscera, commonly the liver. It commonly affects children between 2 months and 15 years of age. The classical clinical presentation is a child presenting with vomiting, headache, convulsions, and signs of encephalopathy, associated with hepatic dysfunction and elevated liver enzymes. Liver biopsy classically shows fatty infiltration. A liver biopsy can help to rule out other conditions that may be affecting the liver and causing liver dysfunction.
The explanation of Reye’s syndrome pathology is linked to hepatic and neuronal mitochondrial dysfunction triggered by certain toxins or infections. The most common causes of Reye’s syndrome are reaction to salicylate (aspirin) or infections like influenza B and chicken pox (varicella-zoster virus infection). Chicken pox encephalitis can lead to the development of Reye’s syndrome among other cerebral complications.
Signs on MRI
Typically, CLN lesions show hyperintense curvilinear lines along the cortical gyri and convolutions on both T1W and FLAIR images (. Fig. 2.2.8), usually seen from 1 month to 1 year after the ischemic event.
Fig. 2.2.8
Axial T1W (a) and T2W (b) brain MRI in a 7-year-old child with chicken pox encephalitis shows cortical laminar necrosis (CLN). Notice the curvilinear hyperintense signal intensity lines that follow the convolutions of the gyri on both hemispheres (arrowheads), with diffuse white matter lesions seen on T2W images
CLN lesions show contrast enhancement after contrast injection, classically 2 weeks after the initial attack.
In Reye’s syndrome, the brain shows diffuse or focal ischemia in a laminar pattern similar to CLN. Multiple abnormal white matter lesions with heterogeneous signal intensities may be found reflecting different stages of Wallerian degeneration. The latter finding is commonly seen in chronic stage of the disease. Wallerian degeneration is a type of neurological degeneration that is seen after an axonal injury. The distal and the proximal segments of the damaged axon with its myelin are fragmented until the first node of Ranvier.
Man-in-the-Barrel Syndrome
Man-in-the-barrel syndrome (MIBS) is a rare disease characterized by paralysis of both arms while the cranial nerves and motor functions of the legs are functioning, giving the patient appearance of a man being confined to a barrel.
MIBS is caused by bilateral ischemia or infarction involving the border-zone areas between the anterior cerebral artery and the middle cerebral artery (MCA) (watershed zones) at the level of the motor cortex of the arm. Spinal cord disease paralyzing both brachial plexus can cause this disease, but it is extremely rare.
The most common cause of MIBS is systemic hypotension following cardiac surgeries or cardiac arrest. MIBS association with pontine myelinolysis, head trauma, and cerebral metastasis has been reported.
Signs on CT and MRI
CT can be normal.
MRI typically shows bilateral low T1 and high T2 signal intensities in the parietotemporal, sensorimotor cortical areas of the arm and hand in the brain (. Fig. 2.2.9).
Fig. 2.2.9
Axial brain FLAIR MR illustration shows the radiological findings in patients with man-in-the-barrel syndrome (MIBS)
Locked-In Syndrome
Locked-in syndrome (LIS) is a rare disease characterized by normal wakefulness and cognition, anarthria, quadriplegia, and gaze paralysis, caused by ventral pontine infarction.
Patients with LIS are fully conscious, yet they experience almost complete motor paralysis. Typically, patients are able to communicate via vertical gaze movement and blinking. LIS is classically caused by infarction or hemorrhage involving the pontine perforating arteries arising from the basilar artery. Other causes of LIS include Guillain–Barré syndrome, West Nile encephalitis, and amyotrophic lateral sclerosis.
Signs on CT and MRI
Brain scan typically shows infarction or hemorrhage involving the ventral pontine region or the cerebral peduncles.
Brain Stem Infarction Syndromes
The brain stem holds nuclei of the cranial nerves. The midbrain contains the nuclei of the cranial nerves 3 and 4. The pons contains the nuclei of the cranial nerves 5–8, and the medulla contains the nuclei of the cranial nerves 9–12. Infarction within the brain stem can result in cranial nerve palsies among other complications. Different syndromes may arise according to the infarcted region within the brain stem. A summary of the most common disorders arising due to brain stem infarction is presented below:
Millard–Gubler syndrome: unilateral hemiplegia or hemiparesis with contralateral lower motor neuron facial (CN 7) paralysis due to hemorrhage, tumor, or infarction of the pons.
Claude syndrome: unilateral oculomotor (CN 3) nerve palsy with contralateral hemiataxia due to paramedial midbrain infarction (. Fig. 2.2.10).
Fig. 2.2.10
Axial T1W brain stem MR illustration at the level of the pone demonstrates the typical infarction region causing Claude syndrome (arrowhead)
Benedikt syndrome: unilateral oculomotor (CN 3) nerve palsy with contralateral limb tremor or paralysis. It can be caused by pontine infarction due to posterior cerebral artery occlusion.
Weber syndrome: unilateral oculomotor (CN 3) nerve palsy with contralateral hemiplegia due to midbrain infarction.
Avellis syndrome: hemiparalysis of the larynx and soft palate on the same side due to infarction of the nucleus ambiguous in the medulla oblongata (central) or a mass lesion around the jugular foramen (peripheral) involves the glossopharyngeal and the vagus nerves (CN 9 + 10) (. Fig. 2.2.11).
Fig. 2.2.11
Axial T1W brain stem MR illustration at the level of the medulla demonstrates different kinds of diseases according to the area of medullary infarction: (a) Avellis syndrome, (b) Cestan–Chenais syndrome, (c) Reinhold (hemimedullary) syndrome, (d) Babinski–Nageotte syndrome, (e) Wallenberg syndrome, and (f) Dejerine syndrome
Foix–Chavany–Marie syndrome: pseudobulbar palsy (CN 9–12) due to bilateral cortical infarction in the territory of the MCA.
Babinski–Nageotte syndrome: ipsilateral Horner’s syndrome; facial loss of pain and temperature; cerebellar hemiataxia, with paresis of the larynx and pharynx; and contralateral hemiparesis and loss of pain and temperature. The disease arises due to unilateral infarction of the medulla oblongata involving the spinal trigeminal tract and nucleus, nucleus ambiguous, lateral spinothalamic tract, sympathetic fibers, corticospinal tract, and afferent spinocerebellar tract (. Fig. 2.2.11).
Cestan–Chenais syndrome: has same clinical presentation as Babinski–Nageotte syndrome and arises due to unilateral infarction of the medulla oblongata involving the spinal trigeminal tract and nucleus, nucleus ambiguous, lateral spinothalamic tract, sympathetic fibers, and corticospinal tract (. Fig. 2.2.11).
Lateral medullary (Wallenberg) syndrome: ipsilateral Horner’s syndrome; facial loss of pain and temperature; cerebellar hemiataxia, with paresis of the larynx and pharynx; and contralateral loss of body pain and temperature. It arises due to unilateral infarction of the medulla oblongata involving the spinal trigeminal tract and nucleus, nucleus ambiguous, lateral spinothalamic tract, sympathetic fibers, afferent spinocerebellar tracts, and vestibular nuclei (. Figs. 2.2.11 and 2.2.12).
Fig. 2.2.12
Axial FLAIR image in the region of the medulla in a patient with Wallenberg syndrome (arrowhead) shows hyperintense signal intensity lesion in the lateral portion of the right medulla oblongata (arrowhead)
Dejerine syndrome: ipsilateral tongue weakness with contralateral hemiparesis and face sparing hemihypesthesia. It arises due to unilateral infarction of the medulla oblongata involving the hypoglossal nucleus or fibers, corticospinal tract, and spinal medial lemniscus (. Fig. 2.2.11).
Reinhold (hemimedullary) syndrome: ipsilateral Horner’s syndrome; facial loss of pain and temperature; cerebellar hemiataxia, with paresis of the larynx and pharynx; and contralateral hemiparesis and face sparing hemihypesthesia. It arises due to unilateral infarction of the medulla oblongata involving the spinal trigeminal tract and nucleus, nucleus ambiguous, afferent spinocerebellar tracts, sympathetic fibers, hypoglossal nucleus or fibers, corticospinal tract, and spinal medial lemniscus (. Fig. 2.2.11).
Subclavian Steal Syndrome
Subclavian steal syndrome (SSS) is a disease characterized by subclavian stenosis or occlusion at the segment between its origin from the aortic arch and the origin of the vertebral artery. This stenosis or occlusion causes reverse blood diversion (stealing) from the basivertebral arteries through the vertebral artery at the same side of subclavian occlusion to supply the ipsilateral arm (blood flow from the head and neck to supply the arm, rather than flow normally from the aortic arch toward the head via the vertebral artery).
Most patients with SSS are asymptomatic. However, symptomatic patients present with brain stem ischemia or stroke at rest or after exercise due to increased arm blood demand. Also, patients often complain from dizziness, cerebral dysfunction, and drop attacks when the disease is severe. Symptoms in the affected arm ranged between decrease pulses, coldness to claudications.
SSS doesn’t appear when the stenosed subclavian artery is accompanied by vertebral artery arising separately from the aortic arch (6 % of population). Angiography is the gold standard to establish the diagnosis of SSS.
Coronary-subclavian steal syndrome (CSS) is a disease seen in patients with previous history of coronary artery bypass graft surgery (CABG). The internal thoracic (mammary) artery, which is a branch of the subclavian artery, is commonly used as a graft for the left anterior descending artery (LAD). Severe stenosis of the subclavian artery that compromised the arm blood supply causes the blood flow to reverse in direction. The blood is withdrawn (stolen) from the coronary arteries via the internal thoracic artery graft to supply the arm. Patients typically present with exertional angina precipitated or exacerbated by arm exercise. Diagnostic keys of CSS include history of CABG (mandatory), difference in blood pressure between the two arms >20 mmHg, and angina produced by activity of the affected arm, while activity of the contralateral normal arm produces no symptoms.
Signs on Angiography
Stenosis of the subclavian artery is seen in arch aortography. After injecting the contrast within the normal vertebral artery, the contrast is seen flowing within the contralateral vertebral artery via the vertebrobasilar system in a retrograde pattern to supply the arm when the patient is asked to exercise his arm (. Fig. 2.2.13).
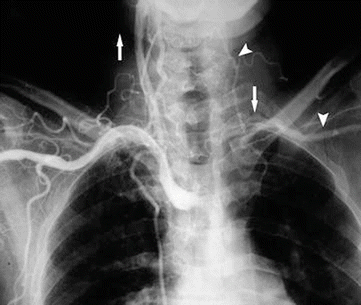
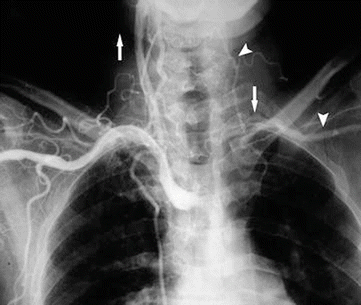
Fig. 2.2.13
Selective right subclavian artery angiogram shows retrograde flow in the left vertebral artery to supply the left arm via the brachial artery (arrowheads). The direction of the contrast flow is demonstrated by the arrows
Signs on Doppler Sonography
The earliest manifestation of stealing phenomenon is a transient sharp deceleration of blood flow after the first systolic peak. This deceleration is observed as a systolic peak with a median notch, creating two systolic peaks of the vertebral artery with stealing phenomenon. The nadir of the notch becomes progressively lower until it reaches and crosses the baseline.
On rest, the vertebral artery flow shows double peak systolic waveform with a median notch. The waveform is classified according to the velocity of the nadir into a nadir velocity greater than that of end diastole (type 1), a nadir velocity equal to the level of end diastole (type 2), a nadir velocity that reaches the baseline (type 3), and a nadir velocity that crosses the baseline (type 4).
After asking the patient to exercise his ipsilateral arm or applying brachial artery blood pressure cuff and then deflating it to induce the stealing phenomenon, the arterial flow waveform of the vertebral artery is reversed, and it is seen below the baseline, confirming the reversal blood flow.
Signs on MRI
In axial sections of 2D time-of-flight sequence, the vertebral artery with stealing phenomenon shows flow void signal compared to the contralateral vertebral artery and both internal carotid arteries (localizer sign), which indicates reversal of flow.
Further Reading
Benito-Leon J, et al. “Man-in-the-barrel” syndrome: MRI and SPECT imaging. Eur J Radiol. 1997;24:260–2.
Cuisset T, et al. Coronary-subclavian steal syndrome: an usual cause of refractory unstable angina. Int J Cardiol. 2008;127:e181–2.
Deleu D, et al. “Man-in-the-barrel” syndrome as delayed manifestation of extrapontine and central pontine myelinolysis: beneficial effect of intravenous immunoglobulin. J Neurol Sci. 2005a;237:103–6.
Elting JW, et al. Predicting outcome drome coma: man-in-the-barrel syndrome as potential pitfall. Clin Neurol Neurosurg. 2000;102:23–5.
Ferrari G, et al. Foix-Chavany-Marie syndrome: CT study and clinical report of three cases. Neuroradiology. 1979;18:41–2.
Girija AS, et al. Neurological complications of chickenpox. Ann Indian Acad Neurol. 2007;10:240–6.
Hoffmann HJ. Moyamoya disease and syndrome. Clin Neurol Neurosurg. 1997;99 Suppl 2:S39–44.
Holz A, et al. Moyamoya disease in a patient with hereditary spherocytosis. Pediatr Radiol. 1998;28:95–7.
Hsu C-Y, et al. Moyamoya disease: the clue from computer tomography. J Emerg Med. 2004;26:339–42.
Hurwitz ES, et al. A cluster of cases of Reye syndrome associated with chickenpox. Pediatrics. 1982;70:901–6.
Kaneko A, et al. Color-coded Doppler imaging of the subclavian steal syndrome. Intern Med. 1998;37:259–64.
Kim I-O, et al. Mitochondrial myopathy-encephalopathylactic acidosis and strokelike episodes (MELAS) syndrome: CT and MR findings in seven children. AJR Am J Roentgenol. 1996;166:641–5.
Kinoshita T, et al. Reye’s syndrome with cortical laminar necrosis: MRI. Neuroradiology. 1996;38:269–72.
Kliewer MA, et al. Vertebral artery Doppler waveform changes indicating subclavian steal physiology. AJR Am J Roentgenol. 2000;174:815–9.
Komiyama M, et al. Serial MR observation of cortical laminar necrosis caused by brain infarction. Neuroradiology. 1998;40:771–7.
Krasnianski M, et al. Babinski-Nageotte’s syndrome and Hemimedullary (Reinhold’s) syndrome are clinically and morphologically distinct conditions. J Neurol. 2003;250:938–42.
Krasnianski M, et al. Between Wallenberg syndrome and hemimedullary lesion. Cestan-Chenais and Babinski-Nageotte syndromes in medullary infarctions. J Neurol. 2006;253:1442–6.
Luxenberg EL, et al. Locked-in syndrome from rosto-caudal herniation. J Clin Neurosci. 2009;16:333–4.
Marquardt F, et al. The coronary-subclavian-vertebral steal syndrome (CSVSS). Clin Res Cardiol. 2006;95:48–53.
Masuzawa H, et al. Pontine gliomas causing locked-in syndrome. Childs Nerv Syst. 1993;9:256–9.
Prasad BKD, et al. Cerebral amyloid angiopathy. Ind J Radiol Imag. 2006;16:745–7.
Roldan-Valadez E, et al. Imaging diagnosis of subclavian steal syndrome secondary to Takayasu arteritis affecting a left-side subclavian artery. Arch Med Res. 2003;34:433–8.
Sheehy N, et al. Contrast-enhanced MR angiography of subclavian steal syndrome: value of the 2D time-to-flight “localizer” sign. AJR Am J Roentgenol. 2005;185:1069–73.
Trattnig S, et al. Colour Doppler imaging of partial subclavian steal syndrome. Neuroradiology. 1993;35:293–5.
Van Son JAM, et al. Diagnosis and management of the coronary-subclavian steal syndrome. Eur J Cardiothorac Surg. 1998;3:565–7.
Yamada I, et al. Moyamoya disease: diagnostic accuracy of MRI. Neuroradiology. 1995;37:356–61.
Zakaria T, et al. Locked-in syndrome resulting from bilateral cerebral peduncles infarctions. Neurology. 2006;67:1889.
2.3 Intracranial Hemorrhage
Intracranial hemorrhage is a condition characterized by the presence of free blood within the cranium. The free blood can be collected in the epidural space, subdural space, subarachnoid space, intrabrain parenchyma, or intraventricular spaces.
Intracranial hemorrhage can be caused by head trauma, anticoagulants use, ruptured aneurysms, vascular malformations, and hypertension. The most common areas of intracranial hemorrhage are the temporoparietal region and the cerebellum. Native, nonenhanced CT is the diagnostic modality of choice as an initial diagnostic modality to detect intracranial bleeding.
Blood exhibits different densities on CT or signal intensities on MRI according to the age of the hemorrhage (acute, subacute, or chronic) (. Fig. 2.3.1).
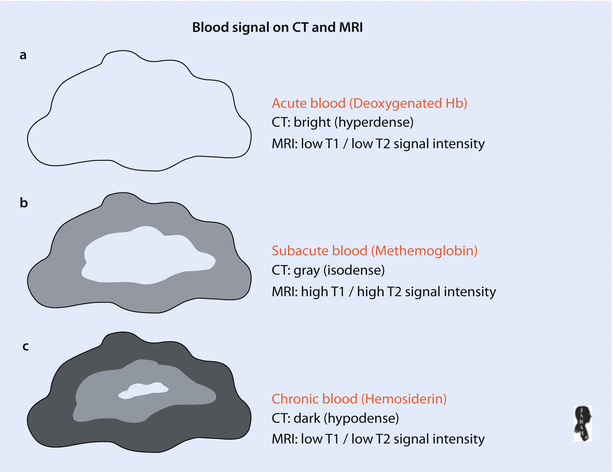
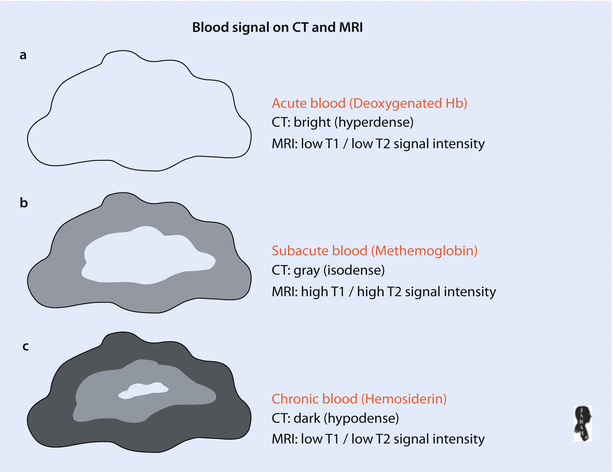
Fig. 2.3.1
Illustration demonstrates the different hematoma ages and manifestations on CT. (a) Acute blood (Deoxygenated Hb). CT: bright (hyperdense). MRI: low T1/low T2 signal intensity. (b) Subacute blood (Methemoglobin). CT: gray (isodense). MRI: high T1/high T2 signal intensity. (c) Chronic blood (Hemosiderin). CT: dark (hyperdense). MRI: low T1/low T2 signal intensity
Epidural Hematoma
Epidural hematoma is a free blood collection located between the inner skull table and the dura matter. It is a life-threatening emergency that usually results from trauma to the middle meningeal artery (85 % of cases).
Patients usually present with nausea, vomiting, and altered consciousness.
Signs on CT
The CT typically shows semi-convex-shaped, hyperdense blood collection usually located in the parietotemporal area (. Fig. 2.3.2).
Fig. 2.3.2
Axial CT images of two different patients show acute epidural hematoma (a, arrowhead) and acute on top of chronic hematoma (b, arrow). Notice the mass effect on the left lateral ventricle in (a) when the hematoma is acute and lack of the pressure effect on the lateral ventricles in (b) when the hematoma is chronic
The collected blood does not cross suture lines as the dura matter is firmly attached to the clavaria.
There is significant mass effect over the ventricles and the brain parenchyma in the acute phase.
It is almost always acute. However, acute on top of chronic epidural hematoma can occur uncommonly, and it is seen as a semi-convex blood collection with hypodense and hyperdense component (. Fig. 2.3.2).
Q: When can you find a black (hypodense) hematoma although the bleeding is acute?
This is a rare condition that is seen when the hemoglobin level in the blood is less than 4 mg/dL, because the hyperdense density that reflects the X-ray photon absorption by the iron in the blood is inadequate.
Subdural Hematoma
Subdural hematoma is a free blood collection located between the dura matter and the arachnoid. Subdural hematoma usually arises due to emissary vein tear from a minor trauma or due to uncontrolled anticoagulant therapy. Acute subdural hematoma is a clinical emergency, where patients present with signs similar to epidural hematoma. In contrast, chronic subdural hematomas present with less severe symptoms, such as headache, nausea, and vomiting.
Differential Diagnoses and Related Diseases
Subdural hygroma is a collection of cerebrospinal fluid or serum in the subdural space (. Fig. 2.3.4). It is believed to be caused by chronic subdural hematoma in the elderly or due to intracranial infections in children. Up to 30 % of cases arise after head trauma. The condition is self-limited and is thought to be caused by a tear in the arachnoid that functions as a one-way valve, allowing cerebrospinal fluid to enter the subdural space to be trapped in little or no absorption. The most common symptom is headache with or without nausea and vomiting; in the acute phase, subdural hygroma behaves like an enlarged intracranial hemorrhage, and in the chronic phase, it behaves like a space-occupying lesion. After traumatic head injury, development of subdural hygroma is noted 6–46 days after the initial trauma.
Signs on CT
Crescent-shaped, hyperdense blood collection usually located in the frontoparietal region (. Fig. 2.3.3). It can be bilateral in 15 % of cases.
Fig. 2.3.3
Axial CT images of two different patients show acute subdural hematoma (a, arrowhead) and chronic subdural hematoma (b, arrow). Again notice the pressure effect over the lateral ventricles in the acute subdural hematoma (a) compared to the chronic subdural hematoma (b)
The bleeding is not bounded by the sutures.
There is significant mass effect over the ventricles and the cisterns.
Subdural hematoma can be acute (hyperdense), subacute (isodense), and chronic (hypodense) (. Fig. 2.3.3). Acute on top of chronic subdural hematoma can occur, and it is seen as crescent-shaped blood collection with hypodense and hyperdense components (sedimentation subacute subdural hematoma).
Subdural hygroma is seen as a cerebrospinal fluid collection in the subdural space (. Fig. 2.3.4).
Fig. 2.3.4
Axial brain CT of a patient with a history of posttraumatic brain injury shows large left frontal subdural hygroma
Subarachnoid Hemorrhage
Subarachnoid hemorrhage is characterized by the presence of free blood within the subarachnoid space and the arachnoid cisterns. It most commonly occurs as a complication of ruptured arterial aneurysms and trauma to the head. Patients typically present with sudden severe headache, nausea, and vomiting with neck stiffness.
Signs on CT
The cerebrospinal fluid spaces and cistern will be seen hyperdense (white) due to blood mixed with cerebrospinal fluid (. Fig. 2.3.5).
Fig. 2.3.5
Sequential axial CT images (a) & (b) of a patient with subarachnoid hemorrhage show hyperdense suprasellar cistern (a) and Sylvian fissures (b) (arrowheads) due to subarachnoid bleeding
There is no midline displacement.
Intracerebral/Intraparenchymal Hemorrhage
Intracerebral hemorrhage is the presence of free blood within the gray or the white brain matter. It commonly arises due to stroke, embolic vascular occlusion, and tumors or after vascular rupture due to head trauma. Hypertension causes bleeding into the basal ganglia in 60 % of cases.
Signs on CT
There is hyperdense blood collection within the brain parenchyma that usually follows a vascular territory (. Fig. 2.3.6).
Fig. 2.3.6
Axial brain CT of a patient with intraparenchymal bleeding in the region of the right middle cerebral artery shows large area of intraparenchymal bleeding surrounded by cytotoxic edema exerting mass effect over the right anterior and posterior horns of the right lateral ventricle (arrowhead)
When the bleeding is due to stroke, it is surrounded by a halolike edema (cytotoxic edema), while when it is due to a tumor, a fingerlike edema is seen surrounding the blood collection (vasogenic edema).
Intraventricular Hemorrhage
Intraventricular hemorrhage is bleeding into the ventricles. Commonly, it occurs secondary to parenchymal or subarachnoid hemorrhage and associated with diffuse axonal injury of the corpus callosum. Arteriovenous malformation is the most common cause for spontaneous intraventricular hemorrhage in adults. There are two types of intraventricular hemorrhage:
Ependymal intraventricular bleeding: the blood is seen fixed to the ventricular walls.
Free intraventricular blood: the blood is seen located in the posterior horns (gravity dependent).
Signs on CT
There is hyperdense blood within the ventricles, either in a free form lying in the posterior horns or encapsulated within the ependymal ventricular wall (. Fig. 2.3.7).
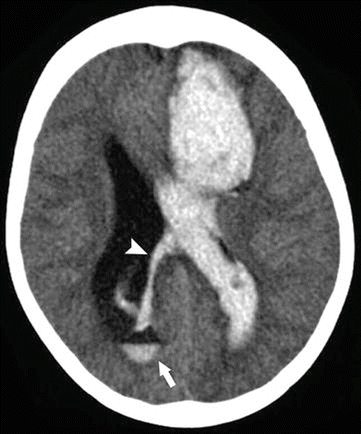
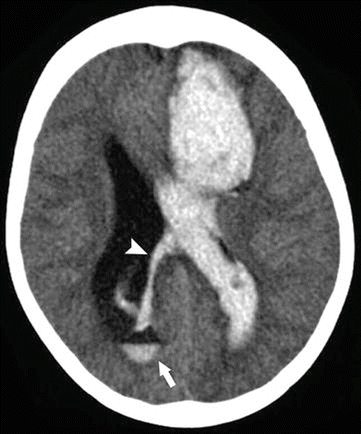
Fig. 2.3.7
Axial brain CT of a patient with severe intraventricular hemorrhage shows dilated both ventricles due to bleeding, with subependymal (arrowhead) and free (arrow) intraventricular bleedings also seen
Signs on MRI
Chronic bleeding can be detected on T2* images as hypointense intraparenchymal areas (. Fig. 2.3.8).
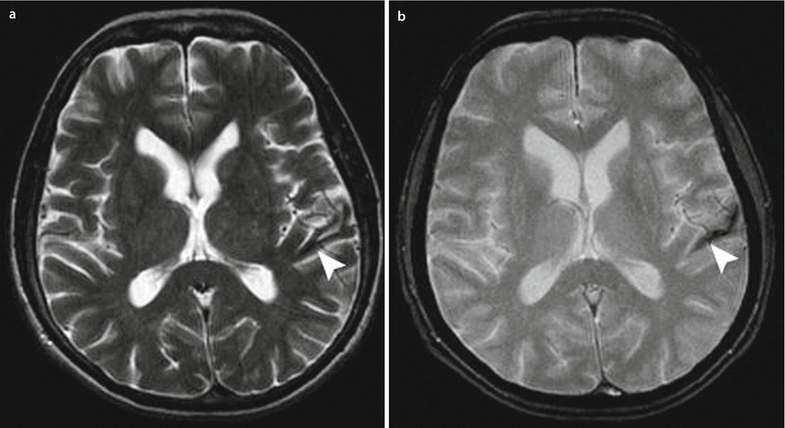
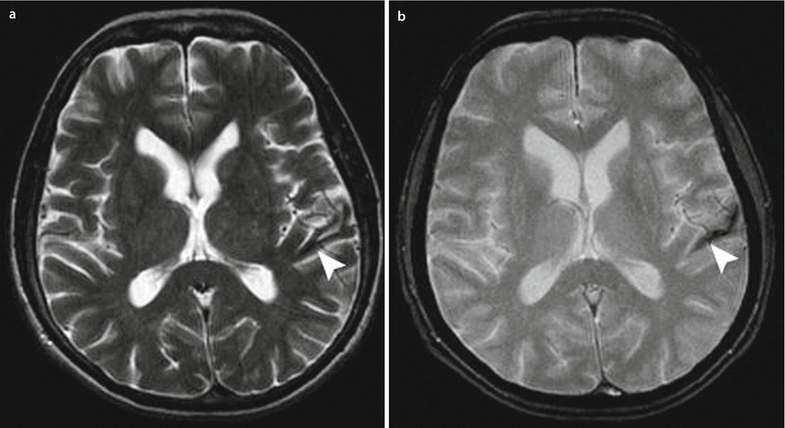
Fig. 2.3.8
Axial T2W (a) and T2* MRI of a patient with previous intraparenchymal bleeding in the left temporal lobe shows area of focal hypointense signal intensity on (b) due to hemosiderin. Notice the same area is visible on (a) but not as clearly seen as in the T2* image
Hemorrhage into Malignancy
Hemorrhage into neoplasms accounts for 10 % of spontaneous intracranial hemorrhage. It can be seen in 14 % of metastases from melanoma and bronchogenic carcinoma and in 5 % of cases of gliomas. Bleeding occurs because abnormal tumor vascularity usually occurs in higher-grade malignancies.
Signs on CT
Atypical location for bleeding in a patient with known primary or secondary brain malignancy.
The signal intensity of the blood is more heterogeneous than that of nonneoplastic hemorrhage. This heterogeneous texture is attributed to the multiple episodes of bleeding with different ages (mixed hypodense and hyperdense pattern).
Further Reading
Dincsoy MY, et al. Intracranial hemorrhage in hypothalamic low-birth-weight neonates. Child’s Nerv Syst. 1990;6:245–9.
Gross A, et al. Intraventricular hemorrhage originating from choroids plexus angioma in a road accident victim. Z Rechtsmed. 1989;102:409–13.
Heros RC, et al. Cerebral vasospasm after subarachnoid hemorrhage: an update. Ann Neurol. 1983;14:599–608.
Koc RK, et al. Acute subdural hematoma: outcome and outcome prediction. Neurosurg Rev. 1997;20:239–44.
Laguna P, et al. Intracranial hemorrhage in a boy with severe haemophilia A and factor VIII inhibitor. Child’s Nerv Syst. 2006;22:432–5.
Masuzawa T, et al. Computed tomographic evolution of post-traumatic subdural hygroma in young adults. Neuroradiology. 1948;26:245–8.
Masuzawa T, et al. Computed tomography evolution of posttraumatic subdural hygroma in young adults. Neuroradiology. 1984;26:245–8.
Moster ML, et al. Chronic subdural hematoma with transient neurological deficits: a review of 15 cases. Ann Neurol. 1983;14:539–42.
Park CK, et al. Spontaneous evolution of post-traumatic subdural hygroma into chronic subdural hematoma. Acta Neurochir (Wien). 1994;127:41–7.
Schellinger PD, et al. Intracranial hemorrhage, the role of magnetic resonance imaging. Neurocrit Care. 2004;1:31–45.
Schwartz DT. Sensitivity of computed tomography for subarachnoid hemorrhage. Ann Emerg Med. 2009;53(1):160–1.
Xi G, et al. Intracerebral hemorrhage, pathophysiology and therapy. Neurocrit Care. 2004;1:5–18.
2.4 Meningitis
Meningitis is a disease characterized by inflammation of the meninges due to infections or inflammatory disease (e.g., sarcoidosis). Infectious meningitis can be bacterial (e.g., pneumococcus) or viral (e.g., Haemophilus influenzae).
Patients with meningitis classically present with fever, neck stiffness, and neurological symptoms. Rarely, meningitis may lead to suprarenal gland suppression, causing patient death due to adrenal gland insufficiency. Infection of the meninges occurs due to hematogenous spread (e.g., bacteremia) or from direct extension from local infectious pathology (e.g., otitis media). Imaging in meningitis is mainly performed to evaluate complications.
Signs on CT and MRI
Meningitis is detected typically as thickened meninges with contrast enhancement. Meningeal enhancement is divided into pachymeningeal and leptomeningeal enhancement. The pachymeninges are the dura matter, with its thick inner meningeal component, and its inner table of the skull (periosteum) component. The leptomeninges are the pia and the arachnoid matters. Pachymeningeal enhancement is seen as enhancement of the inner skull table and meningeal reflections (e.g., falx cerebri) (. Fig. 2.4.1). In contrast, leptomeningeal enhancement is seen as thin linear enhancement that follows the pial surfaces, the cortical gyri, and fills the subarachnoid spaces (. Fig. 2.4.2).
Fig. 2.4.1
Axial T1W postcontrast brain MRI shows right frontal pachymeningeal enhancement with epidural abscess formation (arrow)
Fig. 2.4.2
Axial postcontrast brain CT shows enhancement of the leptomeninges around the ambient cisterns (arrowheads)
Ventriculitis is seen as an enhancement of the subependymal surface of the ventricles after contrast injection.
Subdural pus collection (empyema) is an extra-axial pus collection that usually results from untreated or chronic meningitis (crescent sign).
Abscess is seen as an area of low density on CT or low T1 and high T2 signal intensities on MRI with uniform rim enhancement after contrast injection (. Fig. 2.4.3). The abscess is commonly surrounded by vasogenic edema.
Fig. 2.4.3
Axial postcontrast brain CT shows right temporal abscess with thin rim enhancement and vasogenic edema (arrowhead) that exerts mass effect over the anterior horns of the lateral ventricles
Subdural hygroma is a sterile collection of fluid located in the subdural space usually as a sequela of meningitis in children.
Hydrocephalus may arise due to inflammation of the basal meninges blocking the fourth ventricle. It is seen in advanced stages of meningitis. Dilatation of the temporal horns is a definite sign of hydrocephalus.
Post meningioencephalic sequela is a severe advanced stage of meningitis characterized by loss of brain tissue (encephalomalacia) and parenchymal calcification with hydrocephalus (. Fig. 2.4.4).
Fig. 2.4.4
Axial nonenhanced brain CT shows postmeningoencephalic parenchymal and meningeal (falx) calcification (arrowheads)
Superior sagittal sinus thrombosis may be seen as a triangular filling defect on axial images (delta sign).
Differential Diagnoses and Related Diseases
Multiloculated hydrocephalus is a clinicopathological condition characterized by enlarged, loculated ventricles with paraventricular porencephalic cavities. The condition is seen in neonates, commonly as a sequel of ventriculitis complicating neonatal meningitis. Neonates present with hydrocephalus, neurological deterioration, and seizures. Mortality rate is high (>70 %). CT and MRI typically show multiloculated ventricles with irregular borders and internal septae (. Fig. 2.4.5).
Fig. 2.4.5
Axial nonenhanced brain CT of a neonate shows multiloculated hydrocephalus
Canalis basilaris medianus is a congenital anomaly characterized by a well-defined channel seen in the midline of the basiocciput, very close to the anterior rim of the foramen magnum. It is seen on CT or MRI as a linear defect in the midportion of the clivus (. Fig. 2.4.6). Although it is an asymptomatic anomaly, it can be the source of recurrent meningitis in children due to transmission of bacteria from the superior nasopharynx into the central nervous system through this basiocciput defect.
Fig. 2.4.6
Axial CT illustration of the base of the skull shows a linear median bony defect of the clivus (canalis basilaris medianus)
Vogt–Koyanagi–Harada syndrome is a rare, sporadic, and systemic disorder mostly seen in adults and characterized by acute panuveitis, meningitis, and cutaneous manifestations. The disease arises due to a widespread pathology affecting the melanin-forming cells in different organs, typically in dark-skinned people. Uveitis is inflammation of the uvea, which supplies nutrition to the globe and is composed of the iris, ciliary body, and choroid. Any part of the uvea can be involved in the inflammation (e.g., iritis), and patients typically present with a painful eye, with pain in the distribution of the trigeminal nerve (because the ciliary body is supplied by the ophthalmic division of the trigeminal nerve). The disease has three phases: a prodormal phase characterized by fever, severe headache, and tinnitus; an ophthalmic phase characterized by bilateral uveitis and optic disk hyperemia; and a convalescent phase seen weeks after the ophthalmic phase, characterized by premature graying of hair (poliosis), vitiligo, alopecia, painful hearing (dysacousia), tinnitus, and vertigo. Diagnostic criteria include the absence of ocular trauma with the following: (a) bilateral chronic iridocyclitis, (b) posterior uveitis including retinal detachment, (c) neurological signs with signs of meningitis (e.g., neck stiffness), and (d) cutaneous findings of alopecia, vitiligo, or poliosis. Signs on orbital CT or MRI may show choroidal and scleral thickening due to chronic inflammation on postcontrast images or retinal detachment with typical (V-shaped sign) on severe cases (. Fig. 2.4.7). Uncommonly, the disease can present with optic neuritis, seen as enhanced optic nerve on postcontrast images on both CT and MRI.
Fig. 2.4.7
Orbital CT postcontrast (a) and nonenhanced (b) illustrations of a patient show left eye choroidal/scleral thickening and enhancement due to uveitis in (a) (arrowhead), with V-shaped sign of retinal detachment in the left eye in (b)
Further Reading
Albanese V, et al. Neuroradiological findings in multiloculated hydrocephalus. Acta Neurochir. 1982;60:297–311.
Bilaniuk LT, et al. Computed tomography in meningitis. Neuroradiology. 1978;16:13–4.
Gilbert JA, et al. Vogt-Koyanagi-Harada syndrome: case report and review. J Emerg Med. 1994;12:615–9.
Jacquemin C, et al. Canalis basilaris medianus: MRI. Neuroradiology. 2000;42:121–3.
Kamra P, et al. Infectious meningitis: prospective evaluation with magnetization transfer MRI. Br J Radiol. 2004;77:387–94.
McGehee BE, et al. Bilateral retinal detachment in a patient with Vogt-Koyanagi-Harada syndrome. Emerg Radiol. 2005;11:366–71.
Rao NA, et al. Vogt-Koyanagi-Harada disease diagnostic criteria. Int Ophthalmol. 2007;27:195–9.
Smirniotopoulos JG, et al. Patterns of contrast enhancement in the brain and meninges. RadioGraph. 2007;27:525–51.
Splendiani A, et al. Contrast-enhanced FLAIR in the early diagnosis of infectious meningitis. Neuroradiology. 2005;47:591–8.
2.5 Encephalitis
Encephalitis means inflammation of the brain parenchyma. Brain inflammation can result from different etiologies, most commonly viruses and autoimmune inflammation.
Patients with encephalitis typically present in early stages with headache or flu-like illness, followed by alteration in consciousness, drowsiness, confusion, fever, and seizures. Coma may result in severe cases.
This topic discusses the different kinds of encephalitis with their characteristic radiological features.
Limbic Encephalitis
The brain can be divided into regions according to functions. The first part is the brain stem, which plays a role in the basic attention, consciousness, arousal, heart and respiration adjustment, temperature control, and sleep–wake cycle control. The second part is the limbic system, which controls the behavior related to food, hormones and sex, jealousy, sadness and love, pleasure, and fight-or-flight responses. The limbic system is composed of the hippocampus, thalamus, hypothalamus, and amygdala. The third part is the rational brain (neocortex), which controls logic, thoughts, speaking, planning, and writing.
Limbic encephalitis (LE) involves inflammation of one structure or more related to the limbic system. LE can be caused by infections (e.g., herpes simplex virus) or autoimmune response.
Herpes encephalitis (HSE) is caused by herpes simplex virus type 1 or type 2. HSE type 1 is often seen in children and young adults. It starts as an orofacial infection (gingivostomatitis), which lasts for 1–2 weeks, followed by flu-like symptoms. Patients present with fever, headache, and change in mental status. The virus spreads in retrograde fashion along the trigeminal nerve course or the olfactory bulb into the brain. In the brain, the virus has affinity to infect the meninges, temporal lobes, and the inferior frontal lobe. HSE type 1 is the most common cause of encephalitis (95 %). HSV type 2 is a genital form of HSE that affects neonates delivered by mothers, with herpes infection in the birth canal. It is an uncommon type of encephalitis (15 %), and clinical diagnosis is usually confirmed by cerebrospinal fluid (CSF) analysis that reveals high leukocytes and protein content and detection of the herpes virus DNA by serology.
Autoimmune LE has two forms. The first form is called paraneoplastic limbic encephalitis (PLE), which is seen in patients with particular cancers with paraneoplastic manifestations, such as thymus, lung, breast, and testes cancers. PLE is confirmed by detecting paraneoplastic antibodies in the patient blood like immunoglobulin G antibodies, ANNA 1, PCA 1, CV2, MA 2, and ANNA 2 antibodies.
The other form of autoimmune LE is nonPLE, which has the same picture as PLE in the absence of the serum paraneoplastic antibodies. The most common subsyndrome of the nonPLE is voltage-gated potassium channel (VGKC) antibody-associated encephalitis. This syndrome is commonly undiagnosed due to the lack of awareness about its existence. The diagnosis of autoimmune LE is important because it responds well to immunosuppressive drugs.
Signs on CT
Initially, the scan may be normal or shows hypodense lesions affecting the medial temporal lobes mainly in a bilateral asymmetrical pattern with mass effect and edema (. Fig. 2.5.1). Areas of necrosis and hemorrhage may be seen on nonenhanced contrast images. Postcontrast images show patchy enhancement. The explanation for the temporal lobe affection lies in the reactivation of the virus from the trigeminal ganglia within Meckel’s cave.
Fig. 2.5.1
Axial sequential T2W MR images show bilateral symmetrical hyperintense signal intensities affecting the region of the temporal lobes (both white and gray matters) in a patient with herpes encephalitis (HSE)
Postencephalitic sequel includes parenchymal calcification and dilatation of ventricles.
In HSE, the basal ganglia are often spared.
Signs on MRI
In HSE, hyperintense, ill-defined cortical and white matter areas on T2W sequences with edema, mass effect, and gyral enhancement (. Fig. 2.5.1) are seen. Later in the course of the disease, meningeal enhancement after contrast may be seen due to the spread of the virus to the meninges.
Autoimmune LE shows the same picture like HSE. Bilateral temporal and hippocampal lesions are typically seen. The main difference is based on the CSF analysis detecting the virus antibodies or the autoimmune antibodies. Also, the history of cancer favors the autoimmune encephalitis.
Acute Demyelinating Encephalomyelitis (ADEM)
ADEM is an autoimmune demyelinating encephalitis that arises typically as an immune reaction 2 weeks after viral infection with MMR (measles, mumps, rubella), whooping cough infection (pertussis), or after immunization with MMR vaccine.
In ADEM, there is a hypersensitivity reaction affecting myelin and brain vessels (vasculitis). Patients classically present with sudden onset of neurological symptoms reflecting a wide central nervous system disturbance 2 weeks after viral infection or immunization.
ADEM has the same MRI picture as multiple sclerosis (MS). Unlike MS, which has multiple relapsing episodes, ADEM occurs once in life (monophasic course).
Acute hemorrhagic leukoencephalitis (AHL) is a severe form of ADEM characterized by intraparenchymal hemorrhage. AHL often arises after an upper respiratory tract infection or allergic reaction. The patient will show features of encephalitis, fever, and impaired consciousness.
Signs on MRI
Large multifocal periventricular lesions with mild mass effect giving high signals in T2 (demyelinating areas) exactly like MS plaques, with asymmetric involvement of cerebral hemispheres (. Fig. 2.5.2). MS plaques are often found bilaterally. The demyelinating plaques show ring enhancement after gadolinium injection in a similar fashion like acute MS plaques.
Fig. 2.5.2
Axial T1W (a) and FLAIR (b) images in a patient with acute demyelinating encephalomyelitis (ADEM) after measles, mumps, and rubella (MMR) vaccination show multifocal hyperintense areas in (b) affecting the posterior lobe and the area around the temporal horns of the lateral ventricles (arrowheads). The areas are patchy and asymmetrically distributed
Involvement of spinal cord and the cortical gray matter is common.
Contrast enhancement is not always a feature.
Typically, ADEM does not involve the corpus callosum.
Bilateral optic neuritis may occur.
In AHL, signs of hemorrhagic plaques are found on nongadolinium-enhanced images.
How can you differentiate between MS and ADEM?
MS has an acute and chronic phase, while ADEM has only one acute stage.Stay updated, free articles. Join our Telegram channel
Full access? Get Clinical Tree
