Fig. 1
Overlay of functional activation maps on morphological images (contrast-enhanced T1-weighted 3D data set) visualizing the critical spatial relationship between the left precentral high-grade glioma and the primary motor foot (orange cluster) and hand (yellow cluster) representations. Transverse sections (Images: courtesy of C. Stippich, MD) (Color figures online)
Historically, neurosurgeons have mapped cortical functions invasively by direct cortical stimulation either intraoperatively or preoperatively using implanted subdural grids. However, these methods have severe limitations in terms of presurgical planning and direct intraoperative evaluation of function and are nowadays only used for the confirmation or validation of functional imaging data or intraoperatively when the anatomy has changed due to the procedure. The same applies nowadays for subcortical diffusion tensor imaging of white matter tracts compared to subcortical stimulation (see later).
fMRI imaging studies of motor, somatosensory, and language function as part of the presurgical evaluation process are considered very helpful and used to define the patient’s individual risk for a decline in neurological function. This enables most patients to decide between a more aggressive (gross total) and conservative (subtotal or extended biopsy) resection. Finally this information is used to choose the “cortical entry” of lowest risk and to plan the surgical approach avoiding functional “no entry” areas in all cases. fMRI is confirmed intraoperatively with surgical inspection, with intraoperative neuronavigation, and, in selected cases, with cortical stimulation or evoked potentials. Optimized preoperative protocols were developed by Stippich et al. (1999, 2000, 2003) and performed together with a 3D T1-weighted MR sequence used for neuronavigation. Limitations are a reduced patient’s compliance, motion artifacts, uncontrolled movements during the resting phase or co-movements of other parts of the body, or a paresis due to tumor growth. Minor inaccuracies can be eliminated by correction algorithms; in rare cases, however, the data acquisition has to be repeated.
Implementation of fMRI information in navigation systems is straightforward to the surgeon, when the functional information is already integrated and displayed in the data volume normally read into these systems. When the anatomical and functional information is acquired in the same session, no additional registration of fMRI data is necessary, hereby reducing the amount of registration errors.
A major drawback is the fact that fMRI is still very labor-intensive, requiring a group of MRI specialists for patient training, acquisition of MRI and fMRI data, and the post-processing of the data which requires several hours. New real-time fMRI packages of the manufactures (see later) will help to solve these problems (Moeller et al. 2004).
The importance and usefulness of navigation in neurosurgical cases has been evaluated in 200 neurosurgical cases (Wirtz et al. 1998, 2000) with regard to the planning of the surgical approach, the determination of functional areas, the detection of lesion and its boundaries, and finally the radicality of glioma resection.
For planning the operative approach, in 90 % the use of neuronavigation was considered beneficial by six neurosurgeons, for the definition of anatomy and functional areas in 80 %, for lesion detection in 90 % for definition of tumor boundaries in 75 % and for resection radicality in 65 %.
In a more recent study (Winkler et al. 2005), the additional use of functional MRI has been considered especially helpful for glioma surgery, enabling an extended amount of resection and a reduction of postoperative morbidity as compared to landmark-oriented resection alone. Less impressive were the results for metastases or meningiomas.
A study involving higher magnetic field strengths (3–7 T) has conclusively shown an increase in spatial resolution and signal-to-noise ratio (Chen and Ugurbil 1999; Thulborn 1999). The future use of nanoparticles as contrast media will possibly demonstrate larger lesions, which can be identified and encircled with the navigation tool. Both developments will have further implications for functionally presurgical evaluation with regard to lesion delineation and preservable neurological function and therapeutic options (Cheng et al. 2014; Wegscheid et al. 2014).
4 Applications of Functional Neuronavigation
4.1 Motor and Sensory Systems
Integration of functional data for sensorimotor function into the neuronavigational system is of substantial benefit for patients with perirolandic tumors, in terms of preoperative risk assessment, surgical access, and trajectory planning (Fig. 2) Wengenroth et al. 2011). For example, a patient admitted with a hemiparesis due to a focal central lesion with surrounding edema is first treated with corticosteroid (e.g., 16 mg dexamethasone) in order to evaluate whether the neurological symptoms ameliorate with reduction of mass effect. Persisting paresis strongly indicates tumor involvement in the precentral gyrus or subcortical fiber tracts, which can be confirmed by morphological MRI and diffusion tensor imaging (DTI; see below). In this case, a functional task (e.g., finger tapping) cannot typically be performed contralaterally to the lesion due to the impaired neurological function. However, some information can be obtained from an ipsilateral task eliciting bilateral brain responses (Stippich et al. 2003, 2007; Stoeckel and Binkofski 2010; Tozakidou et al. 2013). Thus, voluntary movements of the unimpaired hand can display the ipsilateral precentral gyrus and premotor area, which—together with postcentral activation induced by contralateral passive somatosensory stimulation—can serve as functional landmarks of the affected motor cortex (see section 4.6 in chapter “Task-based presurgical functional MRI in patients with brain tumors”). In patients with distinct motor deficiencies, a more robust approach is required with tasks like opening and closing a fist to establish signal changes with fMRI (Fig. 3). Alternatively, resting-state fMRI is performed in several centers, which bypasses specific problems (Mitchell et al. 2013).
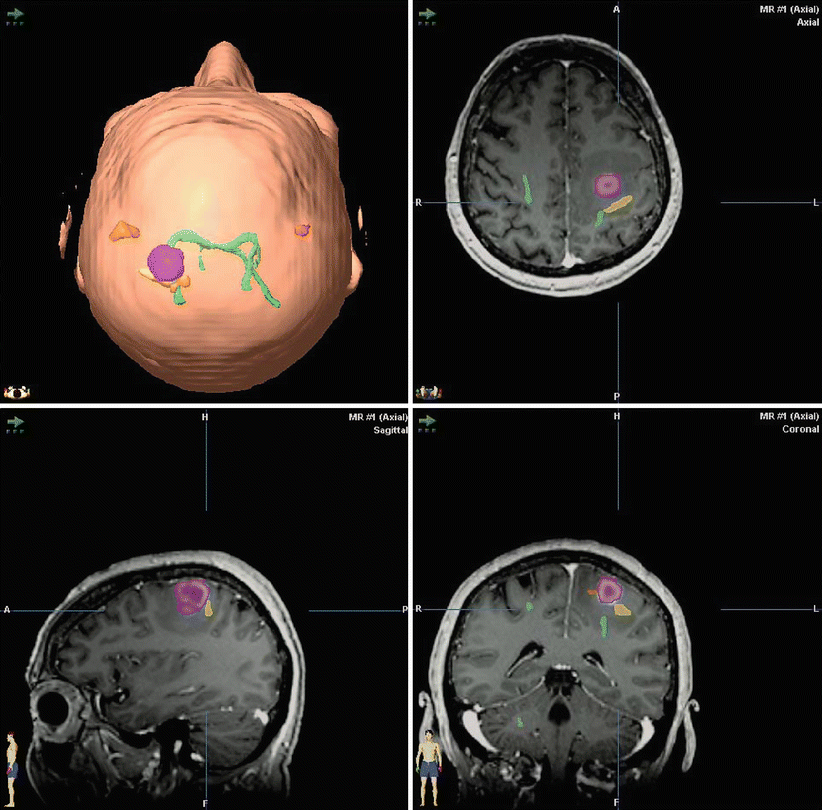
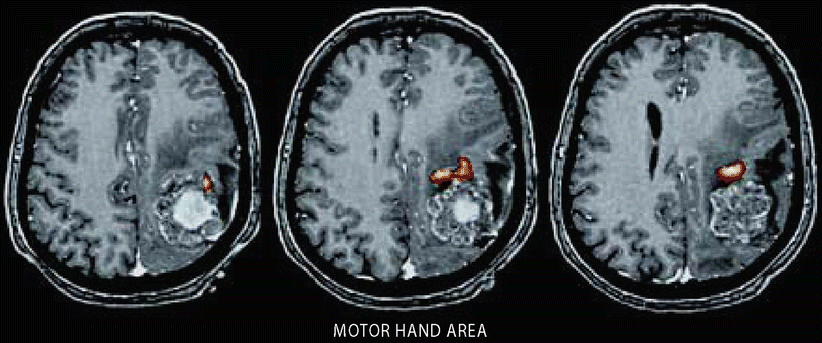
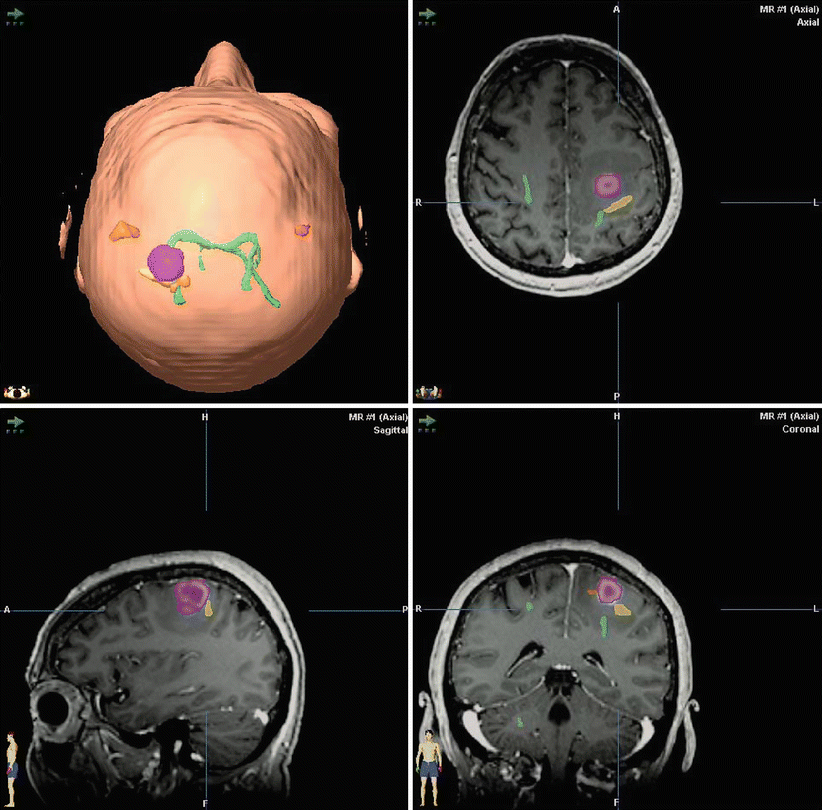
Fig. 2
Integration of BOLD-fMRI somatotopic mapping of the primary motor cortex and of diffusion tensor imaging (DTI) tractography of the pyramidal tract for functional neuronavigation in a patient with a left precentral glioblastoma (same patient in nn). Segmented tumor (pink), foot and tongue representations (orange clusters), hand representation (yellow cluster), pyramidal tract (green lines). The neurosurgeon is provided with detailed information about the spatial relationship between envisaged site of surgery, functional cortex and fiber tracts, facilitating the planning and the performance of function-preserving resection. Conventional surgical view (top left: left – left, right – right). Transverse, sagittal, and coronal views, radiological imaging convention (right – left, left – right) (Images: courtesy of C. Stippich, MD) (Color figures online)
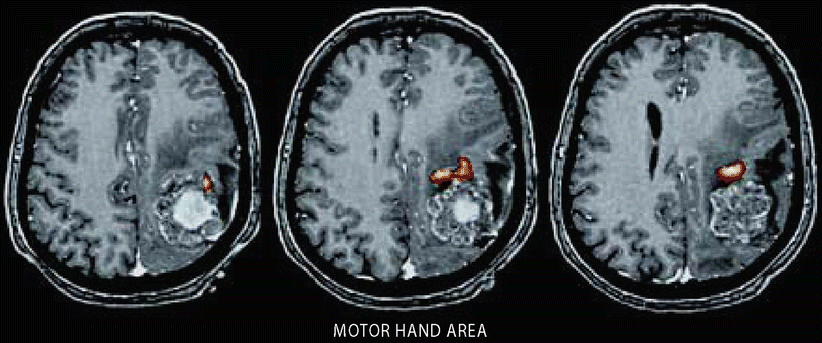
Fig. 3
Hemiparetic patient with a left rolandic metastasis from adenocarcinoma. BOLD-fMRI was performed using hand opening and closure (fist) as motor task. Primary sensorimotor fMRI activation indicated tumor growth into the central region via the postcentral gyrus. A parietal approach was chosen to resect the lesion from a posterior direction. Transverse sections with an overlay of functional activation maps on a T1-weighted contrast-enhanced 3D data set readily prepared for DICOM export to neuronavigation
Thus, functional imaging allows the display of sensori-motor activation in relation to rolandic brain tumors (Bittar et al. 2000; Fandino et al. 1999; Gallen et al. 1994; Jannin et al. 2002), and its validity can be confirmed by direct electrocortical stimulation during surgery (Leherici et al. 2000; Yousry et al. 1995; Meier et al. 2013). However, effects of plastic changes and reorganization also have to be considered (Carpentier et al. 2001; Fandino et al. 1999), and certain limitations with regard to the variability in the venous drainage and regional hemodynamic responses according to the pathology have to be taken into account.
Finally, promising new imaging techniques such as DTI can track subcortical fibers through the corona radiata, the internal capsule, and the crura cerebri (Coenen et al. 2003; Krings et al. 2001; Le Bihan 2003; Zhu et al. 2012) (see chapters “Diffusion Imaging with MR tractography for brain tumor surgery”, “Presurgical functional localization possibilities, limitations, and validity” and “Multimodality in functional neuroimaging”. Although this technique is limited when these tracts pass though the tumor or of the surrounding edema, and correlations between DTI data and electrophysiological information from subcortical stimulation identifying fiber tracts (Duffau et al. 2002, 2003; Keles and Berger 2004) have to be performed, the integration of DTI and fMRI into one neuronavigation system is a major step towards a safe surgical planning and achievement of cortical and subcortical tumor resection (Kamada et al. 2003; Wu et al. 2007).
4.2 Language
Classical functional neuroanatomy locates the motor output of language to the left inferior frontal gyrus (Broca’s area, BA 44, 45) and the perception of language to the posterior temporal lobe (Wernicke’s area, BA 22). In the past language-related functional mapping for neurosurgical planning relied on two invasive procedures, the Wada test (intracarotid amytal injection) and intraoperative direct cortical stimulation. Language mapping is especially important in patients with lesions in and around the language-dominant hemisphere as well as in epilepsy surgery where it determines the extent of resection. The distance of resection from language sites, as determined by cortical stimulation, is the most important variable predicting recovery from postoperative speech disturbances. Thus, an accurate localization of all essential language areas is critical for the speed of recovery as well as the avoidance of postoperative neurological deficits.
For neurosurgical purposes, it was first important to demonstrate whether fMRI could replace the Wada test or not (see chapter “Diffusion Imaging with MR tractography for brain tumor surgery”). In general, there is an excellent correlation between the findings of the Wada test and fMRI for language lateralization (Binder et al. 1996; Rutten et al. 2002), especially in patients with epilepsy (Benson et al. 1999; Woermann et al. 2003). fMRI studies were also correlated with direct electrical cortical stimulation, and a good correlation was found, depending on the language task (Benson et al. 1999; FitzGerald et al. 1997; Signorelli et al. 2003). Based on the preoperative findings in functional imaging, the decision will be made, whether a biopsy, a subtotal resection, or a maximal resection using awake craniotomy will be carried out in brain tumor patients (Fig. 4). Different paradigms have been tried to determine the lateralization for expressive and receptive language areas (Partovi et al. 2012; Zaca et al. 2012), especially in patients with brain tumors or epilepsy. Also in cases with functional deficits, nowadays, resting-state fMRI can be used to demonstrate language neuronal networks (Tie et al. 2014).
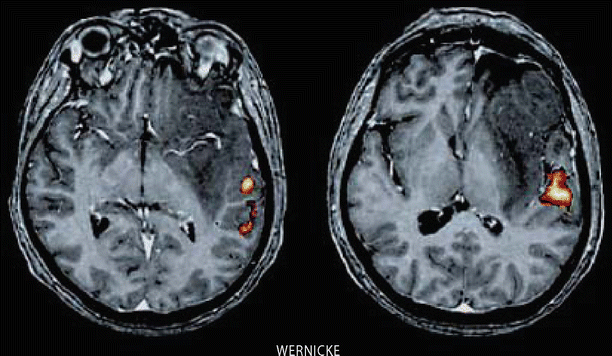
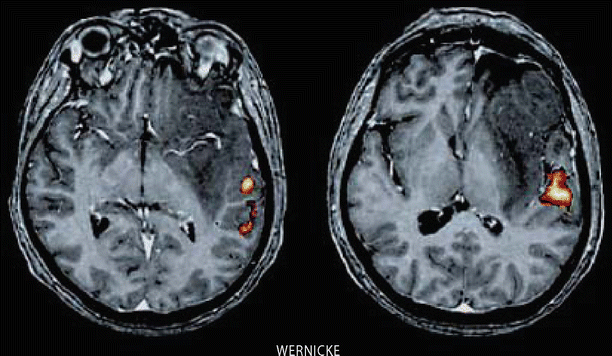
Fig. 4
Functional localization of the Wernicke’s language area in relation to a left frontotemporal low-grade glioma involving the basal ganglia and insula. Word listening (auditory stimulation) was used to elicit BOLD activation. Transverse sections with an overlay of functional activation maps on a T1-weighted contrast-enhanced 3D data set
4.3 Visual Cortex
The cerebral cortex involved in vision constitutes up to one-third of the cerebral cortex. This reflects the importance of vision in everyday life. Visual information is transferred from the retina to the lateral geniculate nucleus and finally to the visual cortex following a well-known retinotopic organization. Originally this information was obtained from PET imaging studies (Fox et al. 1986, 1987) and later confirmed by fMRI (Belliveau et al. 1991, 1992). Since it is important to select an optimal stimulation frequency for visual paradigms (Fox and Raichle 1984; Kwong et al. 1992), stimulation was originally performed with LED goggles. Nowadays computer-simulated patterns are commercially available. Tumors afflicting the visual cortex around the calcarine fissure or the optic radiation can produce changes in functional imaging tasks. Particularly in high-grade gliomas involving the occipital lobe or tumors situated in the posterior temporal horn as intraventricular meningiomas or plexus papillomas, critical planning regarding the extent of resection or the surgical access is necessary to avoid disruption of the optic radiation (Fig. 5). Despite the widespread use of functional mapping in surgery, the identification of visual structures is rarely reported with fMRI (Hirsch et al. 2000; Roux et al. 2001a; Schulder et al. 1999; Li et al. 2013), MEG (Nakasato and Yoshimoto 2000) or PET (Fried et al. 1995). More often DTI is nowadays applied to identify the optic radiation in temporal lobe surgery for tumors or epilepsy (Taoka et al. 2008; Winston et al. 2011). Also there are the first reports of electrical subcortical stimulation of the optical pathways (Gras-Combe et al. 2012) published. A combination of fMRI and DTI displaying visual cortical areas as well as the optic radiation would be desirable to minimize the postoperative risk of visual field defects, which can tremendously affect the quality of life.
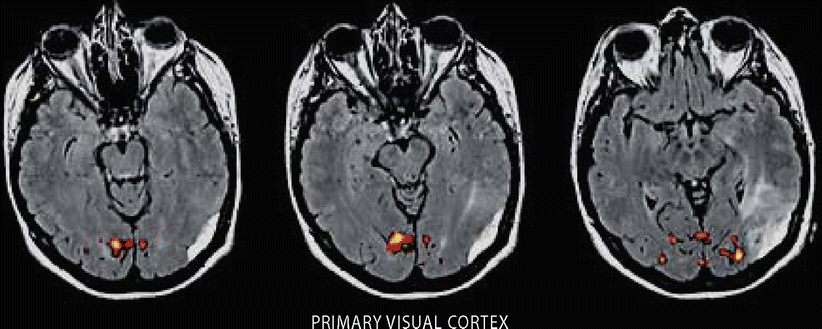
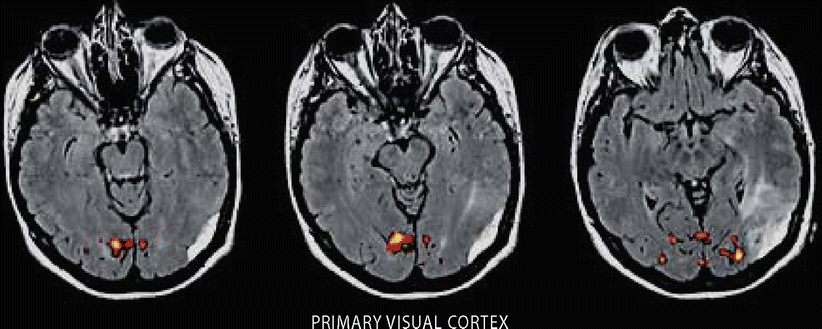
Fig. 5
Functional localization of the primary visual cortex in relation to a left parietooccipital meningioma with signal alterations (perifocal edema) in the left optic radiation. Transverse sections with an overlay of functional activation maps on a T2-weighted fluid-attenuated inversion recovery MR sequence (FLAIR)
4.4 Auditory Cortex
Functional activation of the auditory cortex has not been used for neurosurgical procedures or neuronavigation, yet. It is well known that a tonotopic organization of blood flow responses to different tonal frequencies exists. Sound stimulation activates the primary auditory cortex in the contralateral hemisphere, whereas speech causes bilateral activation (Binder et al. 1994; Lauter et al. 1985). Based on new hypotheses about the origins or consequences of tinnitus, causing neuroplastic changes in the auditory cortex (Langguth et al. 2003; Mahlke and Wallhäuser-Franke 2004; Mühlnickel et al. 1998), a case report described the effects of chronic auditory cortex stimulation implanted with the help of auditory fMRI neuronavigation (DeRidder et al. 2004). Auditory fMRI was superimposed on morphological 3D MRI and displayed together in a neuronavigation system, which was used to place an epidural paddle electrode. After successful test stimulation, an impulse generator was implanted with an excellent result after 10 months.
4.5 Pain
There are numerous studies dealing with the activation of different cortical and subcortical brain areas evoked by mechanical or thermal noxious stimuli. Also therapeutic effects of surgical and nonsurgical measures have been studied. fMRI is used for neuronavigation in cases with central or peripheral neuropathic pain and therapeutic motor cortex stimulation (Pirotte et al. 2005; Roux et al. 2001b; Sol et al. 2001). Depending on the localization of the painful area and the patients’ ability to perform the required tasks, a mapping of the motor cortex is performed. The fMRI data are integrated into a neuronavigation system and displayed in the operating room together with the morphological MRI images. In cases of missing limbs or in plegic patients, virtual movements can be trained or the opposite healthy motor cortex is projected onto the affected site, although in these latter cases, neuroplastic distortions have to be taken into consideration. Via a burrhole approach, an epidural lead electrode can then be placed over the somatotopic representation corresponding to the painful body area (Figs. 6 and 7). Challenging is the fact that due to neuroplastic changes, the functional areas might differ from the morphological data, which is known from several studies on neuroplasticity in pain (Flor 2003; Karl et al. 2004). However, a valid correlation between direct cortical stimulation and fMRI with a mean distance of 3.8 mm has been found in the majority of cases (Pirotte et al. 2005). DTI is used for the detection of the integrity of corticothalamic tracts as presurgical assessment for motor cortex stimulation in central post-stroke pain (Goto et al. 2008). Furthermore, DTI is meanwhile integrated in functional surgery as well. Besides for indications in neuropsychiatric diseases (Bathia et al. 2012; Coenen et al. 2011a, b), it is also used for the stimulation of fiber tracts in chronic pain (Hunsche et al. 2013).
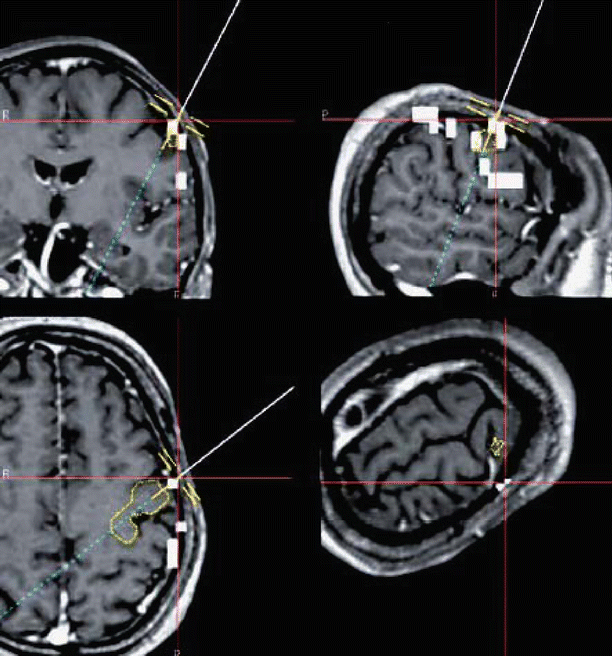
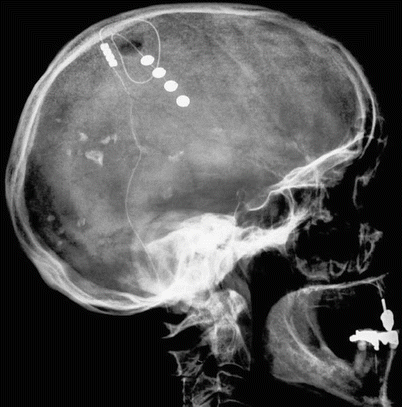
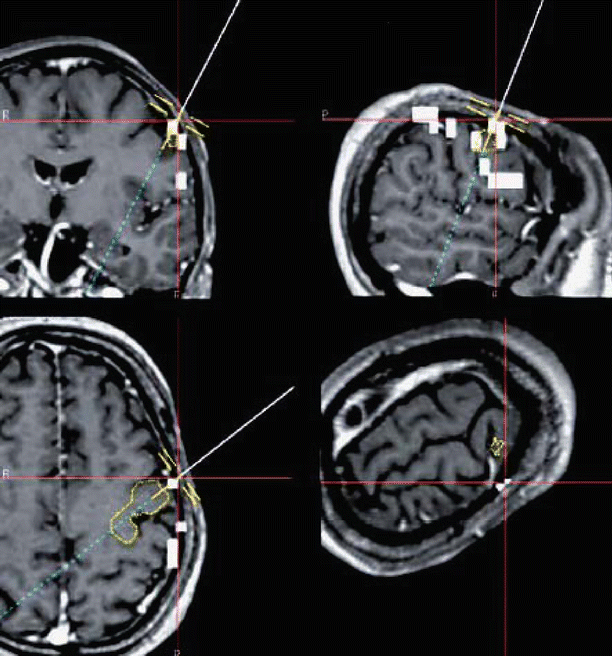
Fig. 6
Functional neuronavigation used for computer-assisted placement of an epidural motor cortex stimulation leads in the treatment of chronic pain. The fused images display anatomical and functional information as well as the site planned for electrode placement (yellow circular lines) along with the navigation device (pointer instrument, blue line). Coronal (top left), sagittal (top right), and transverse views (bottom left), “navigation view” (bottom right). White clusters indicate primary sensorimotor BOLD activation after DICOM export (Color figures online)
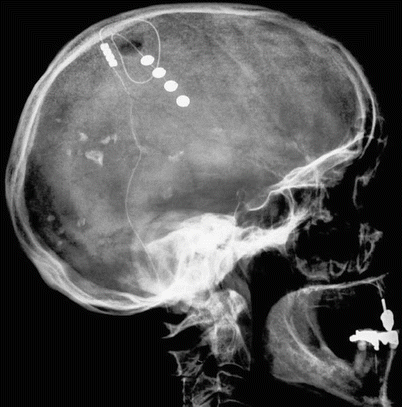
Fig. 7
Lateral x-ray plain film showing the localization of a paddle electrode implanted via a burrhole
4.6 Psychological Function, Memory
Functional imaging has revolutionized our understanding in different neuropsychiatric diseases. Task-related and resting-state fMRI has revealed the neural circuits and functional connectivity in untreated and treated patients as compared to controls (Cheng et al. 2013; Jung et al. 2013). Additionally morphometric and MR spectroscopy is used to highlight treatment effects (Athmaca 2013; Zurowski et al. 2012). Morphological, functional metabolic, and volumetric data point to several brain regions that are important to the etiology and maintenance of obsessive – compulsive disorder (OCD). However, these imaging techniques have not been used for individual deep brain stimulation targeting, although first attempts are well on the way (Gutman et al. 2009). Another interesting topic is the possibility to predict memory changes or deficits after surgical procedures (Henke et al. 2003). This is most important in patients undergoing epilepsy surgery, especially temporal lobectomy (Powell et al. 2004; Rabin et al. 2004). The implementation of activation patterns in the medial temporal lobe (for episodic-like or “event” memory) or temporal cortex (for semantic-like or “fact” memory) into a neuronavigation system will decrease the probability of postoperative memory deficits. This also holds true for frontal lesions, where the patients, different from temporal amnesia, exhibit impairments in memory of temporal events (order, source, or context). The (pre-) frontal cortex is responsible for the active retrieval of memory and encoding, while the temporal cortex is mainly responsible for memory storage and automatic retrieval (Miyashita 2004). Therefore, in the future specifically designed event-related fMRI paradigms will help to predict the risk of certain types of postoperative memory deficits. Also the use of preoperative noninvasive neurostimulation techniques in combination with fMRI (so-called concurrent TMS-fMRI) and the integration of these data in a neuronavigation system will be a territory of future research (Bestmann and Feredoes 2013; Blankenburg et al. 2010; Zaehle et al. 2010)
4.7 Resting-State fMRI
There have been an increasing number of studies using resting-state fMRI to characterize abnormal brain connectivity in patients with different neurological and psychiatric disorders (Horwitz et al. 2013). However, it has not been proven that these functional (neuroplastic) changes can be correlated with anatomical structural changes relevant for the planning of surgical approaches. Although measuring spontaneous activity and generating resting-state correlation maps similar to functional maps from activation tasks, imaging of these networks is technically very labor intensive and underlies several conditions as the selection of the “seed regions” in order to identify the “optimal” networks. In a recent study, the implementation of resting-state data into surgical decision making in a restricted number of patients with epilepsy has been tried. The authors described an advantage in identifying areas responsible for speech arrest, but they did not look for paraphasic errors, which are also important for the functional outcome (Mitchell et al. 2013). For more details on resting-state fMRI, we refer to chapter “Presurgical resting-state fMRI”.
4.8 Diffusion Tensor Imaging (DTI)
While fMRI may be used to highlight cortical structures, DTI is used to map white matter structures and specific fiber connections. It is based on the preferential diffusion of water along white matter tracts within the CNS and allows the neurosurgeon to delineate a lesion with respect to subcortical projection fibers. DTI data can be incorporated into navigational data sets to facilitate the surgeon a radically postoperative resection and to reduce the risks of complications (Elhawary et al. 2011; Wu et al. 2007). Because also the whiter matter undergoes anatomical changes due to brain shift, the intraoperative update of neuronavigational data with high-field MRI is a promising tool for image-guided surgery. The gold standard for the detection of subcortical fibers remains intraoperative subcortical stimulation. Correlational studies estimate that the via DTI-determined white matter tracts are in a vicinity of 8 mm of the stimulated areas, at which a range between 0 and 15 mm has been described (Maesawa et al. 2010; Prabhu et al. 2011; Zolal et al. 2012). Therefore, a combination of DTI integrated neuronavigation with subcortical stimulation is considered the most effective way for a safe resection within subcortical areas.
Another new approach for intraoperative decision making or preoperative planning is the use of navigated transcranial stimulation (nTMS) for neuronavigation. nTMS is currently examined for its applicability and reliability for the delineation of cortical and subcortical projection areas which have to be spared during surgical resection of brain lesions (Krieg et al. 2012, 2013). The information obtained with nTMS has been compared with direct cortical stimulation, preoperative fMRI, and fiber tracking (Frey et al. 2012). Although nTMS has advantages over fMRI because it does not rely on patients’ compliance, it depends on several parameters (resting motor threshold, intraoperative brain shift), and the value of its use in the daily clinical routine has to be determined yet.
4.9 Implementation of Functional Imaging Data into Intraoperative Imaging
The benefits of integrating functional imaging data into a neuronavigation system have been described so far. In contrast to MEG, fMRI is more widely distributed and therefore nowadays used on a clinical routine basis. fMRI however, depending on the technique and the special requirements, still needs an intense and time-consuming processing phase before the data can be merged into a neuronavigational system. Real-time fMRI packages provided by MR scanner manufacturers now enable the radiologist to get immediate post-imaging results for more “simple” activation tasks. In an earlier study (Moeller et al. 2004), two different routine clinical 1.5 T scanners were used (Magnetom Vision, Siemens and Intera, Philipps) for a motor paradigm (finger tapping). The integrated software performed an automatic motion correction and cluster filtering displaying the activated brain area during each scan. An overlay with the 3D T1-weighted data set could be performed semiautomatically. Integrating these software packages into high-field MR scanners situated in the operating room allows direct intraoperative functional imaging (Nimsky et al. 2004). The extent of resection of brain tumors or lesions in epilepsy surgery can nowadays be further tailored according to the functional information obtained from intraoperative fMRI.
4.10 Implementation of Functional Imaging Data into Intraoperative Mapping
The delineation of cortical structures can be performed intraoperatively either via direct visualization, anatomical correlation to a navigational device, or neurophysiological methods. In case of a large craniotomy, the surgeon is able to identify anatomical landmarks on the exposed cortex and to determine various gyri. The localization of eloquent areas by direct inspection of the brain’s surface is very limited as there are no reliable landmarks except for the motor hand area of the precentral gyrus. Especially in cognitive functions, localization of the recruited brain areas is highly variable precluding any reliable prediction in individual patients. In patients with distorted anatomy or limited surgical exposure, neuronavigational devices or neurophysiological techniques help the surgeon to reliably determine the boundaries of eloquent brain regions. Especially in perirolandic tumors, neurophysiological methods facilitate the delineation of the motor or sensory cortex. Direct electrical stimulation elicits movements in muscle groups corresponding to the homunculus. However, intraoperatively, electrical cortical stimulation can be hampered by agents as relaxants causing a neuromuscular blockade or by the degree of depth of anesthesia. On the other hand, motor responses are also elicited by stimulation of premotor and even sensory areas. Sensory responses by electrical stimulation of the median or the tibial nerve are able to delineate the sensory cortex. The combination of somatosensory evoked potentials (SEPs) and cortical stimulation has already been described in the late 1970s (Woolsey et al. 1979) and later been refined by the use of the phase reversal to differentiate between the motor and sensory cortices (Cedzich et al. 1996; Romstöck et al. 2002) in brain tumor surgery. Nowadays these techniques are used for the validation of functional imaging data by displaying the distance between cortical activation and neurophysiological response and finally by correlating the data with the clinical outcome or neurological function (Cosgrove et al. 1996; Kober et al. 2001; Krishnan et al. 2004; Puce et al. 1995; Schiffbauer et al. 2003) (Fig. 8). Functional imaging has the advantage of being able to depict activation deep in a sulcus, which is not easily accessible for direct stimulation. For further data regarding the validity of functional imaging data, see chapter “Presurgical functional localization possibilities, limitations, and validity”. A combination of both techniques is also used in functional surgery, e.g., in motor cortex stimulation for chronic pain states or tinnitus (Sol et al. 2001; Tronnier et al. 1996).
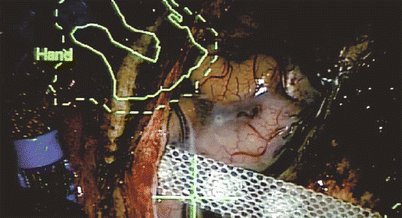
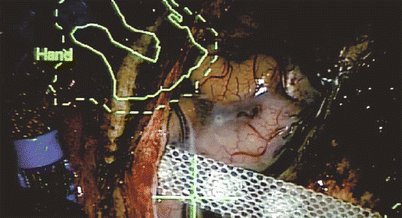
Fig. 8
Operative site showing the correlation of intraoperative cortical stimulation and fMRI localization
Interesting new developments are to operate using a real-time atlas-based neuronavigation adapting intraoperative changes as brain shift to all morphological and functional images (Vabulas et al. 2014)
4.11 Cost-Effectiveness
There are no direct cost analyses of functional imaging in presurgical evaluation. However, it is well demonstrated that neuronavigation is able to lower the duration of hospital stay (Paleologos et al. 2000), hereby significantly reducing the incoming expenses. A study reported the costs of the Wada test to be 3.7 times higher as compared to fMRI (Medina et al. 2004). Increasing the amount of preoperative (functional) imaging will certainly increase the costs produced by manpower and hardware. However, the improved postoperative quality of life is difficult to balance by money. At least in benign lesions or epilepsy surgery, it seems obvious that less invasive procedures will reduce postoperative morbidity and therefore lower the financial burden.
5 Perspectives
5.1 Single-Rack Solution
The creation of a single-rack information system for the surgeon consisting of a collection of data for functional imaging (MEG, fMRI, DTI, and perfusion MRI), navigation and electrophysiological monitoring with online information to support the surgeon’s intraoperative decisions is an important goal. A special focus should be put on the imaging and electrophysiological localization of subcortical pathways. This integrated “functional” neuronavigational approach should be beneficial in surgical planning and pre- and intraoperative decision making, providing online information to facilitate surgical resection and decrease postoperative morbidity by protecting eloquent cortical and subcortical areas. Up to now, the surgeon has to work with different information systems and thus has to integrate all this information during surgery separately when appropriate. An integrated multimodal navigation system taking over all these tasks would reduce the time of surgery, allow a safer resection of brain tumors, and presumably ultimately improve the patients’ outcome.
Several topics have to be addressed to create such a system:
The integration of more complex resting-state fMRI data to allow to predict neuropsychological changes in brain tumor surgeryStay updated, free articles. Join our Telegram channel
Full access? Get Clinical Tree
