23 The past two decades have witnessed an evolution in the robots developed for neurosurgery and spinal surgery. Although the earlier robots were machines capable of a limited number of specific and well-defined tasks, the newer generations of neurosurgical robots are designed to be able to perform more complex maneuvers and have more dexterity for enhanced tool manipulation. Up until the present time, manipulation of the robot has been under the direct control of the neurosurgeon. There is now, however, a trend toward automating some of the functions. We present here a review of the robots that have been developed for neurosurgery and spinal surgery, with an in-depth review of our project at the University of Calgary-neuroArm. Neurosurgical robotics unifies the advantages of high-resolution imaging, neuronavigation, and robotic technology into a tool that can advance the diagnosis and treatment of brain and spinal pathologies. This was a logical evolution over the past several decades. The introduction of the operating microscope propelled the refinement in neurosurgical technique and instruments. The development of computed tomography (CT) and magnetic resonance imaging (MRI) provided enhanced lesion localization and anatomic detail. This allowed for the development of neuronavigation systems which link surgical procedure to the preoperative or intraoperatively acquired images, further increasing precision and accuracy. Together, these technologies provided the basis for progressively narrower surgical corridors, pushing surgeons toward the limits of their spatial orientation and technical ability. Image-guided robotics evolved as a means to overcome these limitations. Computers and robotics are able to process and utilize large amounts of quantitative information and are well-suited to tasks requiring high levels of accuracy, precision, and repetition. We present here a review of the robots that have been developed for neurosurgery, with an in-depth review of neuroArm. Although this is by no means an exhaustive review, it includes systems that have contributed to the development of present day technology, which have clinical application (Fig. 23.1). The review also includes promising robotic systems that may be still in preclinical stages. The programmable universal machine for assembly robot (PUMA; Advance Research and Robotics, Oxford, CT) was the first robot to be used in neurosurgical procedures. In 1985, Kwoh et al used the PUMA 200 robot in a stereotactic biopsy, where the robot was used to orient a cannula through which a needle was inserted.1 In 1991, Drake et al used the PUMA 200 robot as a retractor holder in the resection of pediatric thalamic astrocytomas.2 The PUMA robot had a single arm with 6 degrees of freedom DOF.1 The arm was capable of gripping and manipulating a biopsy cannula or a brain retractor. During surgery, a stereotactic frame base ring was placed on the patient and attached to the CT table. Following CT image acquisition, the stereotactic coordinates of the target lesion are computed, and based on three-dimensional (3D) reconstruction of the preoperative images, a desired trajectory was selected at the workstation.2 The robot arm gripping the surgical instrument could then be programmed to move in line with and along the planned trajectory and the surgical instrument further manipulated with the computer interface. Calibration of the tool tip to the center of the stereotactic frame allowed for its position and orientation to be displayed in relation to the target lesion and the surrounding anatomic structures in 3D. Despite early successes, the project was terminated because of safety concerns related to the operation of an industrial robot in an operating room. Fig. 23.1 Neurosurgical robots: first published clinical use in humans. This timeline denotes the neurosurgical robotic systems in the present review that have been used clinically. The year noted represents that of publication in a peer-reviewed journal of the first clinical use. The systems that are still in their preclinical stages have not been included in this timeline. NeuroMate (Integrated Surgical Systems, Davis, CA) was designed to assist with stereotactic procedures using image guidance.3 It was based on earlier work by Benabid and colleagues at the Grenoble University Hospital in France in the mid 1980s.4 In 1997, it received United States Food and Drug Administration (FDA) approval, for use in stereotactic neurosurgery.5 NeuroMate has been successfully used in numerous stereotactic neurosurgical procedures, including tumor biopsy and placement of electrodes for deep brain stimulation.6,7 The robot has a single arm with 5 DOF and may be used in a frame-based or frameless mode for stereotaxy (Fig. 23.2A).3 In the frameless mode, NeuroMate uses an ultrasound registration system. Preoperative imaging is obtained in the form of CT, MRI, digital subtraction angiography, or digitized radiographs. These are transferred to the planning computer workstation where the target trajectory is defined, using PC-based kinematic positioning software. The finalized plan is then transferred via Ethernet to the control workstation in the operating room. The surgeon uses the defined trajectory to command the robot arm holding the mechanical guide to move into target position, where it is fixed in place (Fig. 23.2B). The surgeon uses the mechanical guide to direct the trajectory of manual drilling or the insertion of a biopsy needle or an electrode. NeuroMate is not able to actuate a biopsy tool or other instruments. The main use of the NeuroMate system was to assist with lesion localization and targeting and provide tool stabilization and support. Fig. 23.2 NeuroMate (Integrated Surgical Systems, Davis, CA) was designed to assist with stereotactic neurosurgical procedures under image guidance. (A) For frameless stereotaxy, registration of the NeuroMate arm to the patient occurs using an ultrasound-based system. (B) After the planned trajectory is defined, NeuroMate moves into the surgical field in preparation for the biopsy. (Courtesy of Qing Hang Li, MD PhD, Wayne State University, Detroit, MI.) The Evolution 1 robot (Universal Robot Systems, Schwerin, Germany) was designed for precision in neurosurgical and endoscopic procedures. Evolution 1 has a single arm and utilizes hexapod platform with 6 DOF.8 Positional accuracy is reported on the order of 10 μm.9 Speed ranges from slow (0.5 mm/s) to fast (2 mm/s). The robot has a small working distance and therefore must be prepositioned by the surgeon ~5 cm above the entry point, in the general direction of use. User interface at the workstation is a touchscreen; intraoperative steering of the robot arm is performed with a joystick. Use of Evolution 1 has been reported in neuroendoscopy as a tool for the positioning and maneuvering the ventriculoscope.8,9 After navigation has been achieved using a combination of preoperative MRI and VectorVision system (BrainLab, Heimstetten, Germany), the skin incision and burr hole are performed manually by the neurosurgeon. The ventriculoscope is then maneuvered through the brain parenchyma into the ventricle, at which point the endoscope is inserted. It has also been adapted for endoscopic transsphenoidal resections of pituitary adenomas.10 Robotic assistance with the endoscope in these cases allows the neurosurgeon to use two instruments simultaneously during surgery. The next logical step in the evolution of the neurosurgical robot was to couple intraoperative imaging with the robot to achieve more accuracy and precision during surgical dissection. The problem of brain-shift invalidates neuronavigation technology based on preoperative images. Intraoperative imaging, with up-to-date rendering of the brain, compensates for brain-shift that might occur during the procedure. In addition, real-time imaging allows the neurosurgeon to be able to make appropriate adjustments to the trajectory of the biopsy cannula or electrode during a stereotactic procedure. Initial efforts with an intraoperative CT system quickly stalled, soon to be followed by the more successful application of intraoperative MRI (iMRI). The Minerva robot (University of Lausanne, Lausanne, Switzerland) was the first real-time image-guidance system, designed solely for stereotactic neurosurgical procedures. The robot was designed to work within a CT scanner. This meant that instrument position during a stereotactic procedure could be monitored by taking successive scans, producing real-time imaging. Minerva is positioned behind the CT scanner with the arm operating inside the CT gantry.11 The robot is linked to the CT table and moves freely along the longitudinal axis on rails. The stereotactic head frame is coupled to the scanner table to ensure precision with regard to the patient’s head position. The robot arm has 5 DOF. Depth electrodes and biopsy instruments have been specially developed for use by the robot. Once the target lesion and trajectory have been selected, the authors claimed that skin incision, bone drilling, dural perforation, and probe manipulation could occur in an automated fashion.12 Minerva offered the potential benefit of decreased total procedure time as the surgery could be performed within the scanner without having to transfer the patient from room to room and without the intermediate step of transferring and calculating coordinates. The first clinical applications of Minerva were the successful aspiration and biopsy of malignant intracerebral cystic lesions in two patients.11 The same group went on to report at least eight subsequent successful stereotactic biopsies.12 Minerva has also been reported in use in phantom studies with 3D electrode probe placement at an accuracy of 1.3 mm.13 Unfortunately, CT scanners can only obtain images in a single dimension, and repetitive scanning meant that the patient was exposed to large doses of radiation: this contributed to the termination of the project. Harvard Medical School, the Surgical Planning Laboratory (SPL) at Brigham and Women’s Hospital (Boston, MA), and the Surgical Assist Technology Group of the Agency of Industrial Science and Technology (AIST; Tsukuba, Japan) developed a robot that is able to work within an iMRI system (Signa SP/i, 0.5 tesla [T], General Electric Medical Systems, Waukesha, WI).14 The open gantry parallel-facing donut-shaped magnets had an air gap of 56 cm, which provided enough room for a surgeon to work within the magnet. The main body of the robot and the actuators are mounted at the top of the magnet, above the head of the surgeon. The end-effector, which has 5 DOF, is attached to the main body of the robot by two rigid arms. The robot was built with titanium and plastics and includes piezoelectric actuators. Essentially, the robot functions as an aid for the precise positioning of probes and biopsy needles in stereotactic procedures. The main clinical use for the SPL/AIST robot has been in prostate biopsies and brachytherapy.15,16 Masamune et al at the Mechatronics Laboratory at the University of Tokyo developed an MRI-compatible needle manipulator for use in stereotactic neurosurgical procedures.17 The manipulator arm and frame attachment are made from polyethylene terephthalate, whereas nonferromagnetic materials, such as brass, aluminum, polyoxymethylene (Delrin; DuPont, Wilmington, DE), ceramics, and polyetheretherketone were used for the bearings, screws, and gears. Piezoelectric ultrasonic motors are used for the actuators. The manipulator and frame, with 6 DOF, are mounted on an XYZ-base stage that is attached to the scanner bed for precision. The entire system fits within an MRI gantry of 60 cm in diameter. Using a personal computer at the workstation, the target lesion can be localized and a trajectory can be selected. Data transfer from workstation to the robot occurs by way of a direct cable connection. Although the earlier neurosurgical robots functioned mainly as mechanical guides for stereotactic procedures, more recent systems saw the development of the robot as a tool for microsurgery. Newer robots incorporated various features to enhance the dexterity of the end-effector, such as haptic feedback, tremor filters, and motion scaling. They had specially designed instruments to be able to accomplish specific tasks. They were designed to enhance microsurgical techniques and procedures. The robot-assisted microsurgery system (RAMS) was designed by the National Aeronautic and Space Administration’s Jet Propulsion Laboratory (Pasadena, CA) in collaboration with MicroDexterity Systems, Inc. (Memphis, TN). RAMS is based on a master-slave control system and is neither MRI-compatible or image-guided. The single robot arm is designed to simulate the movements of a human upper extremity.18 The slave robot has 10 joints and 6 DOF and is controlled by a master robot input handle, which has eight joints and 6 DOF. A laptop computer at the workstation uses a graphics user interface (GUI) for configuration of system parameters. The robot arm is 34.6 cm long, from base to tip, and 2.5 cm in diameter, allowing for a large working field.18 It is mounted on a cylindrical base and driven by tendons. Together, the arm and the base weigh 2.5 kg, and can sustain a load of over 3 pounds. Its working area is 30 cm diameter. While force scaling, motion scaling and tremor filtering have been incorporated into the system; there is no haptic feedback to the neurosurgeon.19 RAMS was designed to enhance the surgeon’s performance. The neurosurgeon holds the master input handle the same way he would hold a conventional surgical instrument, in either his right or left hand, to command the slave robot in the manipulation of tissues or sutures.20 Le Roux et al conducted feasibility studies regarding the use of RAMS for microvascular anastomosis in neurosurgery.20 Rat carotid arteriotomies were performed and closed using either RAMS or conventional microsurgical techniques by surgeons, students, and engineers. Performance was measured in terms of vessel patency, surgical time, and surgical errors. Although RAMS could not be used to hold needles or to place sutures, it was used to manipulate the vessel or hold the vessel wall that was being sutured. The robot was also used to perform knot tying. All the procedures were successfully completed. RAMS was found to be as effective as the surgical group in all areas of performance except for the surgical time, which was twice as long. Taylor et al at Johns Hopkins University (Baltimore, MD) approached robotics systems as a tool to enhance the performance of the surgeon. With the Steady-Hand robotics system, the surgeon manipulates tools that are held by the robot, while the robot controls for the forces exerted and scales down motion amplitude (Fig. 23.3).21
Neurosurgical Robots: A Review
Introduction
Early Neurosurgical Robots
Programmable Universal Machine for Assembly
NeuroMate
Evolution 1
Neurosurgical Robots with Image Guidance
Minerva
MRI-Compatible Robots
Neurosurgical Robots for Enhanced Tool Manipulation
Robot-Assisted Microsurgery System
Steady-Hand Robotics System
Stay updated, free articles. Join our Telegram channel

Full access? Get Clinical Tree
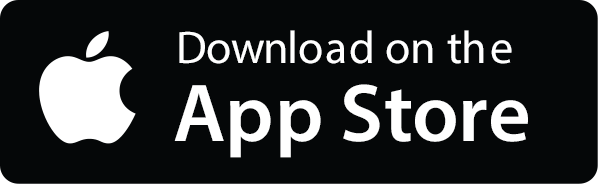
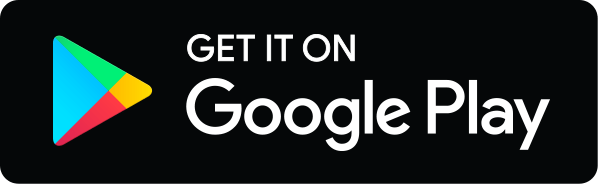