Fig. 16.1
Sixty-six-year-old man with a serum PSA of 5.9 nd/dL and Gleason 3+4 tumor within left mid peripheral zone at transrectal ultrasound-guided biopsy result. Axial T2 weighted MR image demonstrates a hypointense lesion (arrow) in left mid peripheral zone suspicious for cancer with a lateral bulge suggesting a possible extracapsular extension
Detection of extracapsular extension (ECE) is quite important in preoperative staging since its presence upstages the patient to T3A and alters the treatment to a more aggressive approach. On T2W images, ECE usually appears as a direct extension of the tumor into the hyperintense peri-prostatic fat tissue; however, frank ECE may not be seen in all situations. Under those circumstances, the secondary findings should be evaluated; these include asymmetry of the neurovascular bundle, envelopment of the neurovascular bundle, contour angulation, irregular gland margin, capsular obscuration or retraction, and obliteration of the rectoprostatic angle [8, 9]. Seminal vesicle invasion (SVI) can be directly visualized as an extension of tumor from the base of the prostate into the seminal vesicles and the presence of focal low-signal intensity within the normally hyperintense seminal vesicles [10]. Multiplanar evaluation of the T2W images enables more accurate evaluation of ECE and seminal vesicle invasion.
T2W MRI alone is reported to have a wide range of sensitivity and specificity for cancer detection of 27–100% and 32–99%, respectively, depending on many factors discussed below. Sensitivity and specificity for local staging and predicting the presence of extracapsular extension also demonstrate a wide range from 14.4 to 100% and 67 to 100%, respectively [11–25]. The wide range of sensitivities and specificities is mainly due to the significant variability in the patient populations, magnet strengths, coil designs as well as differences in the gold standard correlation methodologies (biopsy vs. surgery), and level of expertise used in different studies.
For a complete local staging, the status of nodal disease is crucial. However, nodal staging is challenging and depends on morphologic enlargement of the node and qualitative assessment of the enhancement pattern if intravenous contrast is used. Generally, MRI is insensitive for node involvement because it depends on node enlargement which occurs late in the natural history of advanced disease. Contrast-enhanced MRI, lymphotrophic ultrasmall superparamagnetic iron oxide (USPIO)-enhanced T2*W gradient-refocused echo MRI offers an alternative to traditional size-based lymph node evaluation [26–28]. After intravenous administration, this contrast agent is carried to lymph nodes where it is processed by dendritic cells. Benign lymph nodes can accumulate this contrast agent, whereas malignant lymph nodes, lacking macrophages, are unable to take the agent up. This allows earlier detection of nodal involvement even if the overall dimensions of the affected lymph node are of normal size [29]. The original iron-based contrast agent (Combidex®) is no longer available; however, another similar agent carboxymethyl dextran-based magnetic nanoparticle (ferumoxytol, tradename: Ferheme®) is available in the USA as an iron replacement therapy in kidney failure patients. Ferumoxytol can be injected as a bolus unlike Combidex® which required infusion over 30 min. It has been reported to be safe for lymph node staging in prostate cancer patients [30], but little data about its efficacy are available.
Diffusion weighted MRI (DW-MRI) evaluates the Brownian motion of free water within tissues. It has been in use for evaluation of acute stroke patients in the last decade and recently there is growing interest in its use for the depiction and follow-up of prostate tumors. Cellularity increases in most cancer types and tissue water diffusion, thus, becomes more restricted since the mean water path length is interrupted by cell membranes. This results in reduced diffusion of water. Similar to other cancer types, prostate cancer often includes tightly packed glandular elements with increased cellularity, abundant stroma, and diminished extracellular spaces which reduce diffusion. DW-MRI is quantified by calculating the apparent diffusion coefficient (ADC) and displayed as a parametric map reflecting the relative ADC. DW-MRI is a time-efficient MR imaging technique and does not require exogenous contrast media. Prostate cancers demonstrate hyperintense signal characteristics when compared to healthy PZ on raw high “b” field DW-MR images, whereas on ADC maps they show decreased signal intensity relative to healthy PZ, reflecting lower water diffusion (Fig. 16.2) [31]. DW-MRI demonstrates a wide range of sensitivity and specificity (57–93.3% and 57–100%, respectively) for tumor detection in various studies, depending on field strength, imaging parameters, patient selection, and validation [32–38]. Additional studies have also demonstrated differences in ADC values among different Gleason grades, thus helping to stratify low-risk from higher-risk prostate cancers [39, 40]. DW-MRI is an intrinsically low SNR imaging sequence, but the emergence of high field strength magnets, the widespread use of endorectal coils filled with inert material such as perfluorocarbon, and parallel imaging approaches have yielded better SNR and spatial/temporal resolution for DW-MRI. Additionally, use of higher gradient strengths (i.e., b values of 1,000–2,000 s/mm2) improves performance for lesion detection [33, 41–43]. Air in the rectum can lead to susceptibility artifacts on DW-MRI and thus studies obtained without endorectal coils or those in which the endorectal coil is filled with air will have suboptimal DW-MRI scans.
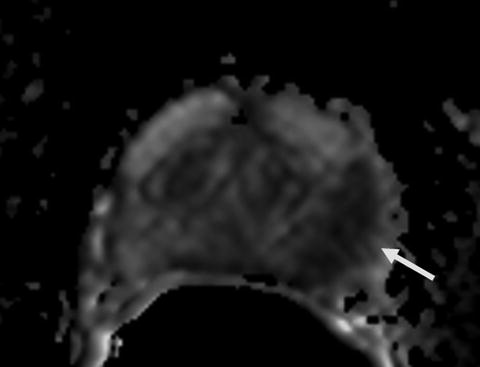
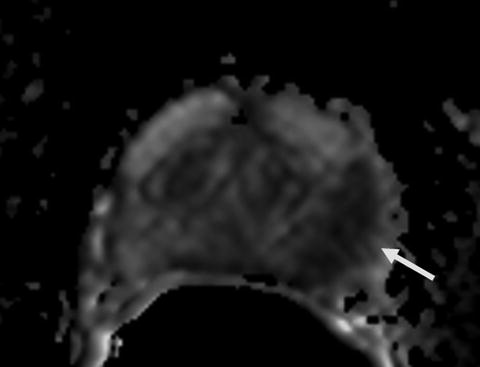
Fig. 16.2
ADC map of diffusion weighted MR image demonstrates a hypointense lesion (arrow) consistent with restricted diffusion secondary to tumoral involvement in the left mid peripheral zone (same patient in Fig. 16.1)
Proton MR spectroscopy imaging (MRSI) provides a display of the chemical composition of the prostate gland through specific metabolites, such as citrate, choline, and creatine which have characteristic resonance frequencies that can be measured with MRSI. The normal prostate gland contains low levels of choline and high levels of citrate, whereas prostate cancers demonstrate increased levels of choline and diminished levels of citrate. The high choline level within prostate cancers is primarily related to increased cell turnover. There is an increased amount of soluble, free choline due to overexpression of choline kinase in prostate cancer tissue [44, 45]. Normal secretory epithelial cells of the prostate gland produce high levels of citrate, and normal prostate glandular cells possess excess zinc with the highest levels the body. Zinc inhibits the citrate oxidizing enzyme “aconitase” and blocks the Krebs cycle, which results in accumulation of citrate. However, in prostate cancer cells, levels of zinc are depleted resulting in unblocked aconitase activity, leading to further oxidation of citrate and thus depleted levels in prostate cancer cells [46, 47]. Owing to the changes in the metabolite levels within prostate cancer lesions, the ratio of choline to citrate (cho/cit) can be used as an index for detecting malignancy (Fig. 16.3). During MRSI, signal from lipid and water must be suppressed in order to focus on the signals coming from the key metabolites, choline, citrate, and creatine which resonate at 3.2, 2.6, and 3.0 parts per million (ppm), respectively. If left unsuppressed, the signal from water and lipid would overwhelm the relatively minor peaks of these key metabolites. The concentration of these metabolites is estimated by measuring the area under each peak after baseline corrections for water and lipid.
Currently, the majority of the experience on prostate MRSI has been at 1.5 T magnets; however, using 3 T or higher field magnets has several advantages for MRSI. Increased field strength enables smaller voxel sizes, improved temporal resolution, and more accurate separation of metabolite peaks (e.g., better separation of creatine and choline peaks). Recent advances in image acquisition and data processing software have led to consideration of other metabolites such as polyamines which are also implicated in prostate cancer metabolism [48]. Integration of MRSI into routine prostate MRI practice improves tumor detection rates [49–51] and estimation of tumor volume, extracapsular extension, radiotherapy response, and post-radiotherapy recurrence [52–54]. However, despite its benefits, MRSI is technically challenging to run since it usually requires the assistance of an onsite MRSI physicist and it is time consuming (∼13–18 min for multi-voxel MRS applications). Moreover, given the long scan duration, patient motion can be a problem. Currently there is no consensus on whether the cho/cit or the cho+cre/cit ratio should be used to analyze the data and what criteria are employed for positivity (generally the ratio should be two deviations above the mean normal ratio) [55, 56].
Angiogenesis is a well-known property of tumor growth. Neoangiogenic tumor vessels are anarchic, inefficient, and highly permeable that are distinct in morphology and overall architecture compared with normal vasculature. The enhancement pattern of a neoplasm depends on the richness of its blood supply and its mean capillary permeability. DCE-MRI is performed with fast T1 weighted MR imaging sequences (mostly gradient recalled echo-GRE) before, during, and after the rapid bolus administration (2–4 cc/s) of a low molecular weight gadolinium chelate. Region of interest measurements over the tumor generate enhancement curves that can be analyzed subjectively or mathematically fit to a two compartment pharmacokinetic model. Tumors show early intense enhancement compared to normal tissue. Moreover, tumors more rapidly de-enhance or washout than normal tissue. The pharmacokinetic model produces several transport parameters, such as K trans (transendothelial transport of contrast medium from vascular compartment to the tumor interstitium), k ep (reverse transport parameter of contrast medium from the extracellular space back to the plasma space), fpV (fraction of plasma volume compared to whole tissue volume) and V e [extravascular, extracellular volume fraction of the tumor (the fraction of tumor volume occupied by EES)] for describing tumor and tissue permeability [57]. Higher grade tumors tend to have higher K trans and k ep parameters compared to normal surrounding prostate tissue (Fig. 16.4). Abnormal enhancement patterns are seen in both tumor foci and BPH nodules, making assessment of the central gland difficult [58, 59]. DCE-MRI alone has reported sensitivity and specificity ranges of 46–96% and 74–96%, respectively, for detection of tumor lesions but, as always, these ranges are highly dependent on patient selection, technique, and diagnostic criteria [32, 60–64]. Despite its accepted utility for detection of primary prostate cancer and posttreatment recurrence, some challenges exist. The parameters generated by the two compartment model have high standard deviations within a tumor and between patients. Moreover, inter-institutional variability is even greater due to variations in implementation. Standardization of image acquisition protocols and analysis techniques is needed to overcome this problem. There is also now new concern over inducing nephrogenic systemic fibrosis (a severe interstitial fibrosis that occurs after intravenous injection of gadolinium chelates) in patients with severe renal failure, especially those undergoing dialysis; therefore, the use of gadolinium-contrast agents should be carefully evaluated in such patients.
Positron Emission Tomography
Positron emission tomography (PET) is a promising tomographic technique that displays the three-dimensional distribution of radioactivity based on the emission of coincidence gamma rays that are emitted after the annihilation of positrons. Because the two gamma rays are emitted approximately 180° from each other, they can be detected by a ring of detectors, and the source of the event can be determined with good accuracy and excellent sensitivity. PET allows noninvasive quantitative assessment of biochemical and functional processes. In contrast to PET, CT uses an X-ray beam to generate tomographic images. CT allows the visualization of morphological and anatomic structures with a high anatomical resolution. Anatomical and morphological information derived from CT can be used to increase the precision of localization, extent, and characterization of lesions detected by PET. Integrated PET/CT combines PET and CT in a single imaging platform. Tumors present a variety of specific biochemical alterations such as increased glucose metabolism, increased protein and DNA synthesis, increased lipid metabolism, and altered receptor phenotypes, which can be imaged by PET with the proper choice of radiopharmaceuticals as targeting probes.
Many PET radiotracers are currently being explored for intraprostatic prostate cancer imaging, including 18F-FDG (18F-Fluorodeoxyglucose), 11C-labeled or 18F-labeled acetate or choline, 11C-labeled methionine, androgen-receptor-avid agents such as 18F-FDHT (16β-18F-fluoro-5α-dihydrotestosterone), anti-FACBC (1-amino-3-18F-fluorocyclobutane-1-carboxylic acid) a synthetic l-leucine analog, a radiolabeled PSMA inhibitor, such as 18F-DCFBC (N-[N-{S-1,3-dicarboxypropyl} carbamoyl]-4-18F-fluorobenzyl-l-cysteine), and 18F-fluoride (for metastatic bone imaging).
Nuclear Medicine Techniques for Imaging of Prostate Cancer
PET Tracers for the Imaging of Prostate Cancer
18F-Fluoro-2-deoxy-2-d-glucose (18F-FDG) was first introduced in the 1970s [65] and has been the most commonly used tracer in PET imaging for more than 30 years. However, its use in the last 10 years has been explosive due to the institution of reimbursement. This has created a vast infrastructure of FDG providers and PET/CT units around the developed world.
FDG is an indicator of increased glycolytic activity. This commonly occurs in cancer cells since there is a glycolytic switch as predicted by the Warburg hypothesis. FDG is actively transported into cancer cells by glucose transporter protein 1 (GLUT1) and is then converted into FDG-6 phosphate by a hexokinase (HK2) [66]. Since FDG-6-phosphate is not a substrate for the enzyme responsible for the next step in glycolysis, it is then trapped and accumulates in the cell, proportionally to the cells transport of glucose and hence its metabolic activity.
Although prostate cancer is reported to be one of the first cancer types studied on PET, the current literature suggests that 18F-FDG has a limited role for detecting localized prostate cancer [67]. In fact, well-differentiated prostate cancers have lower metabolic glucose activity than many other tumor types due to their low expression of GLUT1 transporters (Fig. 16.5) [68]. These tumors are not usually glucose dependent and the tracer often does not accumulate in the prostate tumor. Besides, there is an overlap in the degree of uptake of normal prostate, benign prostate hyperplasia (BPH), prostate carcinoma, postoperative scar tissue, or local recurrence after radical treatment [69–71]. Another major limitation of the FDG study in prostate tumors is the presence of intense bladder activity due to the urinary excretion of FDG, which may easily mask an intra-prostatic lesion in the vicinity of bladder [72].
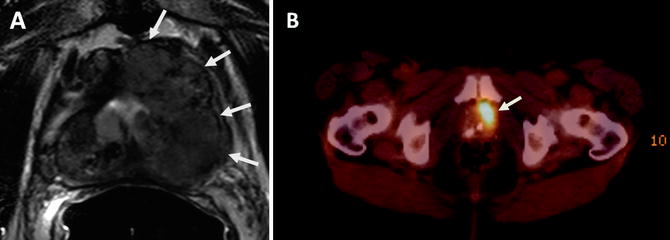
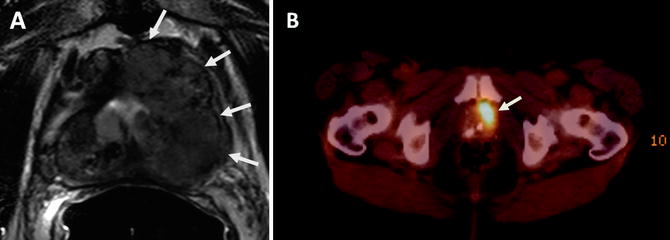
Fig. 16.5
Sixty-seven-year-old male with Gleason 9 prostate cancer in the left hemi-prostate gland. Axial T2W MR image demonstrates a large mass with extracapsular extension (arrows) (a). 18F-FDG PET/CT image demonstrates significant tracer uptake within the left-sided large mass (arrow) (b)
Effert et al. found low uptake of 18F-FDG in 81% of primary, untreated prostate tumors and no correlation between the uptake and the tumor grade or stage [69]. Oyama et al. found a low sensitivity of 64% for the detection of primary, histologically confirmed prostate tumors, but they identified a positive correlation between agent uptake and tumor stage [73]. A few studies have suggested that 18F-FDG becomes more useful in patients with more advanced, androgen-independent tumors and in patients with high Gleason scores and serum PSA levels [74–76].
It is evident that early stage prostate cancer does not tend to follow the Warburg hypothesis. Therefore, there has been a growing interest in other PET radiotracers, which are able to target other possible pathways in tumor growth and progression such as cell membrane turnover, protein synthesis, DNA synthesis, cell surface expression, or testosterone metabolism.
Choline PET may be useful in imaging of prostate cancer. Choline can be labeled with either 11C (11C-Choline) or 18F (18F-Fluorocholine, FCH). Choline is incorporated into malignant cells by conversion into phosphorylcholine, which is trapped inside the cell. This is followed by synthesis of phosphatidylcholine, which constitutes a main component of cell membranes [77]. The biological basis for using the radiolabeled choline is that prostate cancers show elevations of phosphatidylcholine, suggesting the cell needs to incorporate and trap choline first. The expression of the rate-limiting enzyme choline kinase is frequently elevated in prostate cancer cells. Prostate cancer is associated with upregulated choline kinase activity and increased levels of choline.
Choline has a rapid clearance from the blood (∼7 min) and a rapid uptake within prostate tissue with an optimal tumor/background contrast that enables imaging soon after injection. Urinary excretion is very low, minimizing bladder artifacts.
The short half-life of 11C-labeled components (∼20 min) is a major limitation for the widespread clinical use of these compounds as it necessitates an on-site cyclotron and in-house radiochemistry. Choline imaging has been used in the setting of the detection of primary tumors as well as restaging patients with biochemical relapses [78, 79]. However, 11C-Choline uptake was seen in both prostate cancers and hyperplasic prostate tissue [80]. Furthermore, in vivo uptake does not necessarily correlate with tumor aggressiveness [81]. Longer-lived radiopharmaceuticals are now being used to develop related tracers, such as 18F-Fluorocholine (18F-FCH) (half life ∼110 min), but they may not have the same pharmacokinetics as 11C-labeled compounds since they are different at a basic chemical level [78]. Recently, Lord et al. performed simultaneous MRI/PET after 18F-FCH injection in a 47-year-old man with Gleason 9 prostate cancer and was able to localize the tumor and the affected lymph node [82].
11C-acetate is a naturally occurring metabolite that is converted to acetyl-CoA, a substrate for the tricarboxylic acid cycle that is incorporated into cholesterol and fatty acids. The mechanism by which 11C-acetate is taken up in cancer cells is still unclear, but it is postulated that acetate is involved in cytoplasmic lipid synthesis and then becomes incorporated into the cell membrane of tumor cells [83]. It has been suggested that prostate cancer relies more on fatty acid metabolism than glycolysis, with an increase in fatty acid synthesis and the overexpression of fatty acid synthase [84], thus accounting for increased acetate uptake and minimal glucose uptake.
11C-acetate is excreted mostly via the pancreas and intestines and has no urinary tract excretion, which enables imaging of the pelvis without masking caused by bladder activity. On the basis of its metabolic properties and biodistribution, 11C-acetate PET can detect both primary lesions and metastatic disease and may also be useful for monitoring treatment response after prostatectomy or radiation therapy. In a pilot study, Oyama et al. investigated 22 prostate cancer patients with 11C-acetate and showed a positive accumulation of the tracer in all primary tumors, albeit with variable uptake values. 11C-acetate was more sensitive than 18F-FDG PET in the detection of primary prostate tumor [85]. Generally, 11C-acetate uptake appears to be greater in the tumor than in the normal tissue; however, acetate is not a cancer-specific tracer and also accumulates in hyperplasic prostate tissue or even in the normal prostate gland [86]. Jambor et al. assessed the ability of 11C-acetate PET/CT and MRI to localize prostate cancer in 22 patients and reported sensitivity, specificity values of 80%, 29% and 89%, 29% for 11C-acetate PET/CT and MRI. 11C-acetate PET/CT was unable to predict cancer aggressiveness [87]. A recent trial conducted by NCI aiming to characterize the uptake of 11C-acetate in prostate cancer, BPH, and normal prostate tissue in comparison to multiparametric MRI, whole-mount histopathology, and clinical markers concluded that the average SUVmax(10–12 min) of prostate tumors was significantly higher than that of normal prostate tissue, but it was not significantly different from that of BPH. Moreover, 11C-acetate was found to have a comparable accuracy to MRI at 3 T in localizing tumors >0.9 cm (Fig. 16.6) [88]. 18F-labeled formulation of acetate, which would eliminate the need for anon site cyclotron, has been tested in monkeys and pigs and this compound was found not to be a functional analog of 11C-acetate [89].
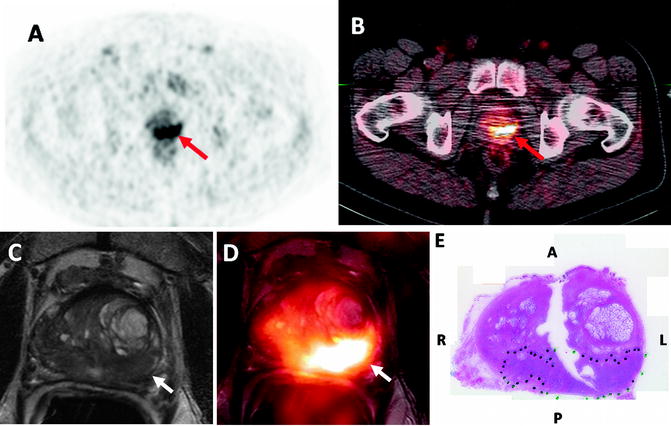
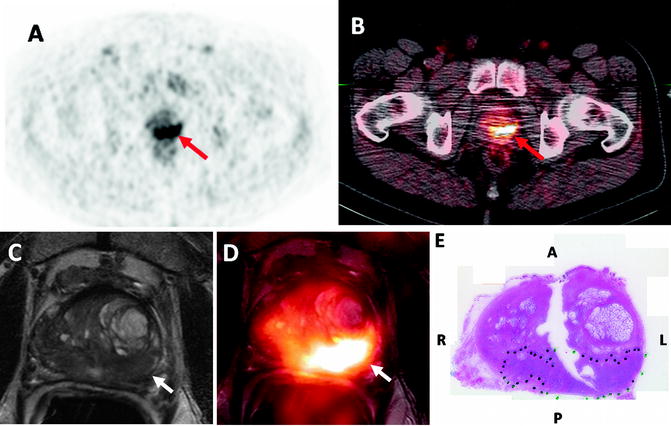
Fig. 16.6
Fifty-eight-year-old male with prostate cancer. 11C-Acetate PET (a) and PET/CT (b) images demonstrate increased uptake in the left mid peripheral zone (arrows). Axial T2 weighted MR image (c) shows a low-signal intensity lesion in the left mid peripheral zone (white arrow) and the software based fused 11C-Acetate PET/MR image (d) better localizes the tumor focus (white arrow). Corresponding pathology slide (e) shows a Gleason 4+5 tumor in the left mid peripheral zone (inked in green) (courtesy of Dr. Esther Mena from NCI, NIH, Bethesda, MD, USA)
11C-methionine is another potential tracer for visualizing cellular proliferation and amino acid transport. Methionine is an amino acid analog that is rapidly cleared from the blood pool and primarily metabolized in the liver and pancreas without significant urinary excretion and, therefore, allows high tumor/background uptake; 11C-methionine uptake reflects increased amino acid transport and resultant protein synthesis related to tumor cell proliferation and turnover. Clinical experience is limited with 11C-methionine, but it has shown advantages over FDG for detecting bone and soft tissue metastases from prostate cancer [90].
Dihydrotestosterone is a primary ligand of the androgen receptor that plays an important role in the growth and proliferation of prostate cancer. Androgen dependence is a characteristic of less advanced disease, whereas androgen independence portends a worse prognosis. Antiandrogen therapy is one of the most effective treatments in prostate cancer, delaying recurrences for years in some cases. 18F-DHT is the PET radiolabeled analog of DHT and has shown high affinity for the androgen receptor and, therefore, may be useful in monitoring therapy response and potentially, in identifying resistance to androgen ablation therapy. A direct correlation between 18F-DHT PET and increased PSA levels has been found by Dehdashti et al. in a cohort of 20 men with advanced disease [91].
18F-DHT has been investigated in patients with progressive metastatic prostate cancer where it can detect metastatic disease not visible with other methods such as bone scans [92]. However, this agent is still highly experimental but remains highly promising as a future research imaging probe.
Recently, the imaging of amino acid transporters with anti-1-amino-3-18F-fluorocyclobutane-1-carboxylic acid (18F-FACBC) has shown promising results for prostate cancer [93]. Anti-18F-FACBC is a synthetic amino-acid l-leucine analog whose uptake by prostate carcinoma cells is likely mediated via the sodium-independent L large-neutral amino acid transport system. It was initially developed to image brain lesions, but it has shown in vitro uptake within the DU-145 prostate carcinoma cell line and orthotopically implanted prostate tumors in nude rats [94]. Compared with 18F-FDG, 18F-FACBC shows no significant renal excretion. Schuster et al. studied 18F-FACBC in 15 patients with newly diagnosed and recurrent prostate carcinoma. The presence or absence of disease was correctly identified in 40 of 48 prostate sextants; pelvic nodal status correlated in 7 of 9 patients, with higher uptake seen in malignant than benign lymph nodes [93]. Schuster et al. also compared the utility of 18F-FACBC with 111In-capromab, an approved anti-PSMA radiolabeled antibody for detection of recurrent prostate cancer in a restaging cohort, and concluded that 18F-FACBC was more sensitive in the detection of recurrences (89 vs. 69%) [95
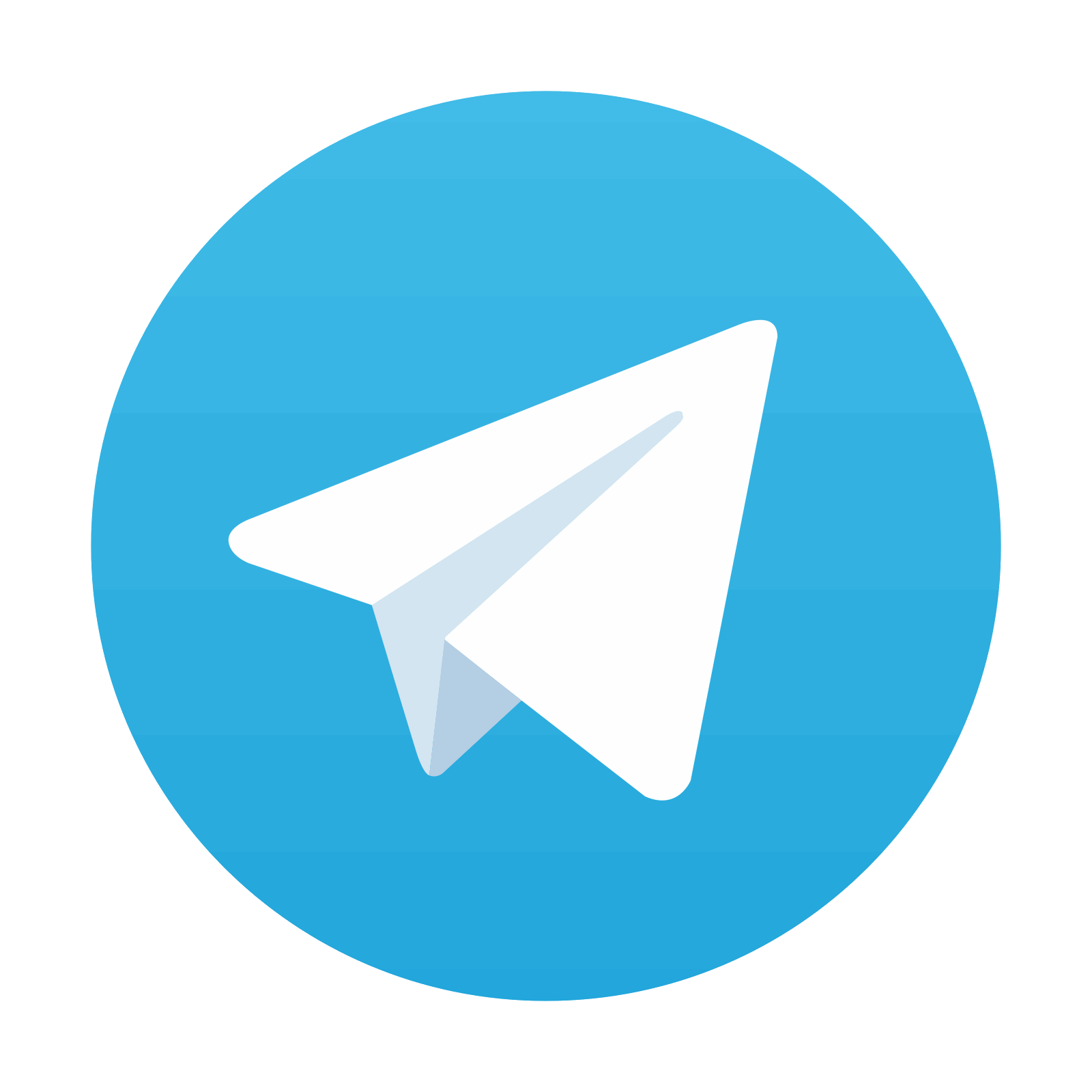
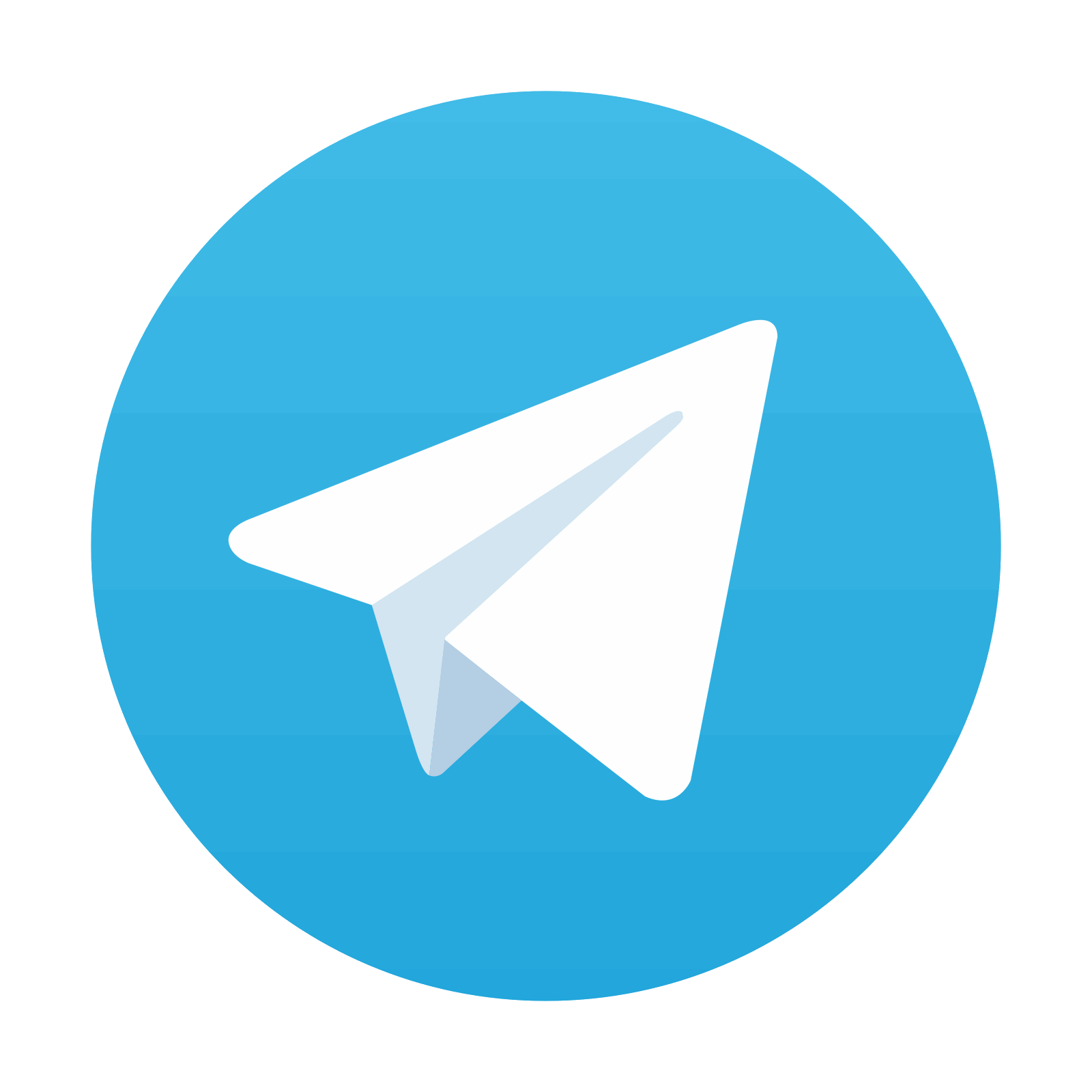
Stay updated, free articles. Join our Telegram channel

Full access? Get Clinical Tree
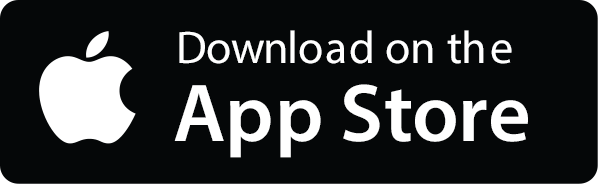
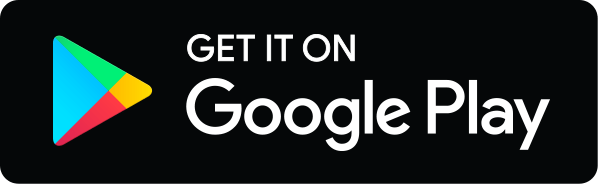