Nondegenerative Diseases of the Spine
Erik H.L. Gaensler
Derk D. Purcell
This chapter focuses on nondegenerative diseases of the spinal cord, meninges, and paraspinous soft tissues, and is divided into sections covering inflammation, infection, neoplasms, vascular diseases, congenital malformations, and trauma (1,2,3,4). The spine is composed of vertebrae, which house the spinal cord and proximal spinal nerves, and thereby represents a “border zone” between the CNS and musculoskeletal system (this is true politically as well as anatomically—with both neurosurgeons and orthopedic surgeons claiming the spine as their province). Disc degeneration and spinal stenosis are covered in Chapter 11. Primary osseous tumors involving the vertebrae are covered in Section X, Musculoskeletal Radiology.
Common Clinical Syndromes
The clinical syndromes produced by degenerative disease and nondegenerative disease can be indistinguishable. Patients with spine disorders present with focal or diffuse back pain, radiculopathy, or myelopathy. Focal back pain without neurologic compromise or fever is not usually an emergency, and is epidemic in our society, with tremendous implications in terms of lost productivity. Focal back pain can be due to a wide variety of both degenerative and nondegenerative processes. In the low back, the he most common causes of pain are orthopedic, such as muscle and ligament strain, facet joint disease, or discogenic disease that does not compromise the nerve roots. However, vertebral metastases or infectious discitis may also cause focal back pain. Since degenerative disease of the spine is far more common than nondegenerative disease, nondegenerative processes may initially be overlooked, with disastrous consequences. Therefore, a good clinical history that specifically addresses any previous cancers, or ongoing fevers and chills, is crucial in raising the suspicion for a nondegenerative process. When history and physical findings are nonspecific, as often is the case, imaging procedures become central to the diagnosis.
In patients with spinal neurologic findings, an attempt should be made to distinguish between the clinical syndromes of myelopathy and radiculopathy, as they differ in significant respects, including degree of urgency. Important distinctions between radiculopathy and myelopathy are summarized in Table 10.1.
Myelopathy results from compromise of the spinal cord itself, due to mechanical compression, intrinsic lesions, or inflammatory processes loosely grouped under the term “myelitis.” Classic symptoms include bladder and bowel incontinence, spasticity, weakness, and ataxia. With cord compression, a clear motor or sensory spinal cord “level” may develop, and knowing this level is helpful in focusing the imaging examination. However, the lesion may be several vertebral bodies higher than the apparent dermatomal sensory level, particularly in the thoracic region. Myelopathy often presents without a clear sensory level, and complete screening of the cord from the cervicomedullary junction to the conus may be required.
The spinal cord, like the brain, has limited healing powers. In fact, the spinal cord in many respects is less tolerant of injury than the brain. A small benign mass, such as a 2-cm epidural hematoma or meningioma, may permanently damage the cord, because of the small diameter of the spinal canal. A similar-sized mass may be asymptomatic within the voluminous calvarium. The “plasticity” of the brain, whereby remaining cortex can assume the function of injured areas through a complex network of redundant neurons, is well documented, particularly in younger patients. The spinal cord, which consists mostly of long linear axonal tracts, demonstrates far less plasticity. After 24 hours of acute severe cord compression, chances of full recovery are significantly diminished. Therefore, acute myelopathy is an emergency, and the radiologist should do everything to facilitate prompt imaging.
Radiculopathy generally results from impingement of the spinal nerves, either within the spinal canal, lateral recess, neural foramen, or along the extraforaminal course of the nerve. This compromise, typically because of mass effect, results in specific dermatomal sensory deficits and/or muscle group weakness. These are outlined in any neurology or physical diagnosis text, and are worth knowing. The most common causes of pain and neurologic deficit are disc herniations and spinal stenosis and, in the cervical spine, uncovertebral joint spurring. Of course, malignant and infectious processes compromise spinal nerves, but overall are less common. The peripheral nervous system, unlike the CNS, has significant ability to withstand injury and to regenerate. Therefore, pure radicular symptoms, although at times excruciatingly painful,
rarely represent a surgical emergency. Extensive epidural neoplasms and infections may present with mixed myelopathic and radicular signs. These patients must be imaged with the urgency of a pure cord syndrome.
rarely represent a surgical emergency. Extensive epidural neoplasms and infections may present with mixed myelopathic and radicular signs. These patients must be imaged with the urgency of a pure cord syndrome.
Table 10.1 Myelopathy Versus Radiculopathy | ||||||||||||||||||||||||
---|---|---|---|---|---|---|---|---|---|---|---|---|---|---|---|---|---|---|---|---|---|---|---|---|
|
Imaging Methods
Conventional radiography of the spine was once the initial test in every spine evaluation, but with newer techniques, this is no longer logical or cost effective. Radiographs continue to be useful for ruling out trauma to the vertebral column and other acute screening settings. Plain radiographs and fluoroscopy are indispensable for correct localization in the operating room. Radiographs have a great deal of useful information to offer when evaluating degenerative processes, particularly with extensive osteophyte formation in the cervical spine. Flexion and extension plain films used to be the only dynamic imaging technique for assessment of spine stability. MR now also can be done in flexion and extension, which can be useful in evaluating cord compression that is positional (see Fig. 10.8).
In nondegenerative disease, careful attention must be paid to the integrity of the vertebral bodies and pedicles, frequent sites of metastases. However, plain radiographs cannot detect early infiltrative changes in the marrow space, which are readily seen on MR. The classic radiographic findings of widened interpedicular distance with tumors, and midline bony spurs with diastematomyelia, are rarely seen except on board examinations!
Myelography. The indications for plain film myelography alone are limited. Myelography today is almost always done in conjunction with CT (see later). Indications include complex postoperative cases and patients in whom MR is contraindicated due to MR incompatible implanted devices. Ionic contrast agents are absolutely contraindicated for myelography, as they can result in severe inflammation, seizures, arachnoiditis, and even death. Always personally inspect the vial of contrast you are using, and fill the syringe yourself!
The recommended dosage of nonionic contrast in adults depends on the region to be studied, the size of the patient, and the size of thecal sac. A convenient and conservative rule of thumb in adults is not to exceed 3 g of intrathecal iodine, which works out to 17 mL of 180 mg/mL, 12.5 mL of 240 mg/mL, or 10 mL of 300 mg/mL, three of the standard concentrations. In general, lumbar myelography should be performed using contrast media with a concentration between 180 and 240 mg/mL, and cervical and/or thoracic myelography should be performed with 200–300 mg/mL. The smaller the area of the subarachnoid space, the denser the contrast must be for good plain films. Plain films and fluoroscopic spot films, however, are becoming increasingly superfluous with the dramatic improvements in multiplanar CT reconstructions.
Myelography begins with a lumbar puncture, with the patient in prone position under fluoroscopy. The preferred puncture site depends on the clinical findings, and usually is the mid lumbar region, inferior to the posterior elements of L2 or L3. This injection level will avoid most disc herniations and spinal stenosis, which are usually worse at lower levels, and the conus, which in adults lies between T12/L1 and L1/L2 disc spaces. Care should be taken to place the needle near the midline to reduce the chances of an extra-arachnoid injection, or spearing of an exiting nerve root. Contrast should be injected only after spontaneous CSF backflow is established. The complications of poor needle placement include subdural and epidural injection. Examples of these complications are well illustrated in older neuroradiology textbooks, and have medicolegal implications, so if in doubt where the contrast is going, stop, take frontal and lateral plain films, and examine them carefully. If tumor or infection is suspected, collect adequate CSF for chemistry, cultures, and cytology if this has not already been done. For routine degenerative cases, CSF examination has not proved worthwhile.
C1–C2 punctures are rarely required, and are inherently more dangerous than lumbar injection, as direct injury to the cord or a low-lying posterior inferior cerebellar artery loop can occur. The puncture is best done under lateral fluoroscopy, placing the needle in the posterior third of the spinal canal between C1 and C2. Classic indications include known blocks caudally, or the need for dense opacification of the cervical and upper thoracic spinal canal for plain films. Today, one of the rare good reasons for a C1–C2 puncture would be complete spine block in the midthoracic region identified by lumbar myelography, with the need to define the upper extent of the block—in a patient with a pacemaker precluding MR. If the pacemaker were not an issue, MR would be the study of choice. MR is far quicker, more comfortable, and, most importantly, safer for the patient. Even if there is no technical complication with a myelogram, patients with spine block can deteriorate from the subtle fluid and pressure shifts that inevitably accompany needle placement in the subarachnoid space, a syndrome known as “spinal coning.” The multiple steps in the evaluation of spine block by plain film myelography followed by CT are shown in Figure 10.1. Contrast this with the simplicity and elegance of MR as shown in Figure 10.2. In oncologic cases, MR has the additional benefit of excellent evaluation of the marrow space—not available with CT.
![]() Figure 10.2. Acute Cord Compression: MR. Evaluation of thoracic cord compression, the easy way—compare with Figure 10.1. A middle-aged patient presented to a physician’s office with acute myelopathy. This emergency MR using T1- and T2-weighted sagittal (A, B) and axial (not shown) sequences took 20 minutes, was completely noninvasive, and gives excellent detail of the marrow space, unavailable on CT. The epidural soft tissue mass (arrows) proved to be lymphoma. |
Space-occupying lesions of the spinal canal are categorized according to their location as intramedullary, intradural-extramedullary, and extradural. This classification comes from myelography, but works equally well as on CT and MR, and is critical in formulating a differential diagnosis. Intramedullary lesions are usually confined to the spinal cord itself, but may be exophytic. Extramedullary lesions are by definition outside the cord, but may be either intra- or extradural. A summary of the radiologic appearance and differential diagnosis for each lesion location is outlined in Table 10.2.
The lesion must be seen in at least two (and preferably three) 90° orthogonal planes, since large intradural lesions may simulate an extradural mass on any single view. Similarly, bilateral extradural disease can flatten the cord, increasing its apparent anteroposterior dimension in sagittal view, giving the false impression of an intramedullary mass (Fig. 10.3). Correlation with axial imaging is invaluable in this regard. Also, remember that lateral lesions, such as lateral disc herniations, may be completely missed by myelography. In almost all cases today, a CT is performed after myelography.
Computed Tomography. The decline of plain film myelography for degenerative disease was initially because of CT, especially CT with intrathecal contrast, which is superior to myelography in diagnostic accuracy. CT has largely been replaced by MR for most screening examinations of the spine, except for acute trauma. Low-dose CT myelography remains the gold standard in cases where the limits of the thecal sac or nerve root sleeves need to be precisely defined, such as in complex postoperative states. Small leptomeningeal (drop) metastases can be identified (see Fig. 10.34); however, MR with gadolinium has replaced CT myelography as the initial screening examination for drop metastases (see Figs. 10.33, 10.35).
CT is far less effective than MR in depicting intramedullary diseases of the spinal cord such as primary tumors, myelitis, and syringohydromyelia. For example, a nonexpansile multiple sclerosis (see Fig. 10.5) plaque will escape detection on any imaging examination except MR.
MR imaging has done for the spinal canal what CT did for the calvarium, allowing for the first time a noninvasive “look inside.” Therefore, it is the examination of choice for any disorder of the spine resulting in myelopathy. The key to MR’s success has been its superior soft-tissue contrast (including the ability to evaluate the marrow compartment), multiplanar capabilities, noninvasiveness, and high sensitivity to gadolinium enhancement.
MR scanning techniques for the spine continue to improve, and with the wide variety of imaging systems available, it makes little sense to recommend specific protocols in a general text. A few general guidelines follow. Surface coils are an absolute must in order to obtain adequate signal to noise in most systems. Motion suppression techniques, such as anterior radiofrequency saturation bands, gradient moment nulling, and cardiac/respiratory gating, are critical to reduce motion artifact. “Fast spin-echo” (FSE) sequences have replaced conventional spin echo for spine work, with great timesaving and little cost when only degenerative disease is present. FSE technique, however, is poor for marrow evaluation, but this can be overcome by using fat saturation with the T2WI, a technique widely used in musculoskeletal MR to search for marrow edema. Short TR inversion recovery (STIR) probably offers the highest sensitivity for marrow space edema. “Fast inversion recovery” techniques compete with T2 FSE with fat saturation as the optimum marrow-screening exam (see Figs. 10.37, 10.43).
Gradient-echo images are poor for marrow space evaluation, because of susceptibility effects from the bony trabeculae, and are of little utility in evaluating nondegenerative spinal disease, except when searching for blood breakdown products (see Fig. 10.65). Ultra thin section imaging (<1 mm) without interslice gaps is now performed with three-dimensional Fourier transformation and rivals CT for thin slice profiles, critical in the cervical spine for examination of the foramina.
Despite motion compensation techniques, both physiologic motion and patient movement remain problematic. Both exaggerate osseous encroachment of the spinal canal and neural foramina, as far more movement occurs during the 4–6 minutes it may take to do a three-dimensional Fourier transformation cervical spine axial image than a 20-msec CT slice. Spin echo MR techniques are generally inferior to CT in the detection of subtle calcification. This may be important in defining
small osteophytic spurs, ossification of the posterior longitudinal ligament, identifying retropulsed bone fragments following trauma, or characterizing calcification in tumors. Gradient recalled techniques may overestimate the size of calcific structures, because of susceptibility effects. Gadolinium is essential in the evaluation of infection and intrathecal metastases, but may obscure vertebral metastases by making them isointense with surrounding marrow fat (Fig. 10.4). Also, it is difficult to evaluate hemorrhage on postcontrast images. Always obtain a precontrast T1 “scout” image to avoid these latter two pitfalls.
small osteophytic spurs, ossification of the posterior longitudinal ligament, identifying retropulsed bone fragments following trauma, or characterizing calcification in tumors. Gradient recalled techniques may overestimate the size of calcific structures, because of susceptibility effects. Gadolinium is essential in the evaluation of infection and intrathecal metastases, but may obscure vertebral metastases by making them isointense with surrounding marrow fat (Fig. 10.4). Also, it is difficult to evaluate hemorrhage on postcontrast images. Always obtain a precontrast T1 “scout” image to avoid these latter two pitfalls.
Table 10.2 Differential Diagnosis of Spinal Lesions By Location | |
---|---|
|
Diffusion imaging can help distinguish between vertebral body metastases and compression fractures, as tumor areas show diffusion restriction as compared with fracture zones (see Fig. 10.36). Diffusion and perfusion studies of vertebral tumors are not routinely performed; however, as needle biopsy is relatively straightforward. There is promise for diffusion, perfusion and spectroscopy techniques for intramedullary disease, as the spinal cord represents a “brain” in miniature. Research in this area, however, has lagged work in the brain for two reasons. The small size of the spinal cord makes MR sampling more difficult, and intramedullary lesions are rarer than brain lesions. There are already case reports of diffusion restriction in spinal cord stroke, and there is some promise for diffusion tensor imaging in evaluating the long axonal tracts of the cord in multiple sclerosis.
Spinal angiography is technically demanding, dangerous in untrained hands, and difficult to interpret. There is no reliable spinal “circle of Willis” allowing collateral flow
from multiple sources, although some variable interconnected vascular arcades exist. Therefore, an inadvertent catheter-induced complication can have tragic consequences. Excellent texts exist on spinal angiography, but this area has increasingly become the province of interventional neuroradiologists, who can both diagnose and often treat spinal arteriovenous malformations—the main indication for spinal angiography. As technology improves, both CT and MR angiography have be used to evaluate the spinal vasculature with increasing success.
from multiple sources, although some variable interconnected vascular arcades exist. Therefore, an inadvertent catheter-induced complication can have tragic consequences. Excellent texts exist on spinal angiography, but this area has increasingly become the province of interventional neuroradiologists, who can both diagnose and often treat spinal arteriovenous malformations—the main indication for spinal angiography. As technology improves, both CT and MR angiography have be used to evaluate the spinal vasculature with increasing success.
Nuclear medicine bone scans are unique in their ability to screen the entire skeleton for metastases in one sitting. When abnormal vertebral uptake is noted, however, plain films may fail to show the abnormality. MR is increasingly used instead, as it demonstrates early marrow space infiltration, and can rule out spinal cord compression. Bone scans are highly sensitive but quite nonspecific, as both degenerative and nondegenerative processes will show increased uptake. When such patients are referred for MR, it is critical to be aware of the bone scan findings in order to protocol the examination appropriately. Bone scans and PET scans are discussed further in the nuclear medicine chapters.
Ultrasound has limited applications in the spine, as in adults the posterior elements obscure the potential acoustic window. However, in neonates the unossified posterior elements provide a window through which spinal anomalies can be evaluated. Excellent work has been done in this highly specialized area, particularly in excluding tethered cord in neonates. Once the laminae have been removed surgically, intraoperative US has proven to be an excellent tool for the evaluation of the spinal cord for tumor, syrinx, and other intramedullary processes, minimizing the need for cord exploration.
Inflammation
This section focuses on inflammatory diseases that cause myelopathy, principally through direct involvement of the spinal cord (5,6,7,8,9,10,11,12). The mechanism of many of these disorders is not fully understood, and they are sometimes lumped under the term “myelitis.” Myelitis may be focal or diffuse. When both clinical and pathologic findings are confined to distinct level(s), the term “transverse myelitis” may be used. It must be recognized that this is not really a specific disease, but rather a category of diseases, and few agree on exactly what processes should be lumped under “transverse myelitis.” In my opinion, it is better to carefully describe the imaging findings, and give a differential diagnosis, than to invoke such nonspecific terms in MR reports.
Multiple sclerosis (MS) is the most common spinal cord “inflammatory” disorder and the most common cause of intramedullary lesions seen on MR. The epidemiology and pathophysiology of MS is reviewed in detail in Chapter 7. Multiple sclerosis of the brain and spinal cord are similar in terms of patient profile, with the hallmark of the disease being multiple neurologic deficits separated both anatomically and temporally. While imaging can be helpful, the diagnosis ultimately rests on clinical grounds. When spinal MS predominates, it tends to follow a progressive clinical course, as opposed to the relapsing/remitting pattern more characteristic with brain involvement. The majority of MS patients have mixed presentations, with both brain and spinal cord involvement. Spinal cord disease is isolated in less than 20% of cases. Two thirds of spinal MS lesions occur in the cervical region.
The best screening protocols are sagittal T2-weighted or inversion recovery sequences, where MS plaques appear as areas of increased signal intensity. Usually there is no significant change in cord diameter, which is why cord MS plaques were typically occult myelographically. Occasionally, there may be subtle cord expansion in the acute phase because of edema, (Fig. 10.5) and “burnt out” MS plaques can present as myelomalacia (literally “cord softening” or atrophy). Cord plaques, unlike brain plaques, may not be visible as areas of hypointensity on T1WI.
As in the brain, plaque enhancement correlates with acute lesion activity (Fig. 10.6). Since the white matter in on the “outside” of the cord, MS plaques tend to be peripheral (Fig. 10.6). However, edema and enhancement can extend into the central gray matter as well, probably because of perivenular inflammatory changes. Differentiation of a solitary plaque from a glial tumor may be difficult, although MS plaques typically are under two vertebral segments in length, and involve less than half the cross-sectional area of the cord. When a mysterious bright intramedullary lesion is seen on T2WI (Fig. 10.5A), the next step should be MR of the brain to search for concomitant MS plaques. The brain and spinal cord are composed of the same tissue types, are physically connected, and share the CSF. Therefore, a good general rule is when presented with any diffuse spinal process, either intramedullary or leptomeningeal, remember to look “upstairs,” because the same process may be involving the brain and its coverings.
Devic disease, or neuromyelitis optica (NMO) is an autoimmune disorder affecting the spinal cord and optic nerves. The spinal cord lesions are longer than in MS, and the brain is often spared initially. NMO was once felt to be a variant of MS, but may be more closely related to acute disseminated encephalomyelitis (ADEM). There is now a specific test for the NMO IgG antibody, which targets the Aquaporin 4 protein in
astrocytes. Treatment differs from MS, and NMO should be mentioned in cases of acute myelitis and optic neuritis.
astrocytes. Treatment differs from MS, and NMO should be mentioned in cases of acute myelitis and optic neuritis.
Lupus Erythematosus. Other CNS inflammatory processes are seen in both the brain and spinal cord. A classic example is systemic lupus erythematosus (SLE), where a necrotizing arteritis leads to cord ischemia and injury. Antibodies may also damage neuronal elements directly. The spinal cord will show diffuse areas of increased signal intensity with cord swelling on T2WI. SLE “lesions” have less well-defined margins than the discrete plaques of MS, and may involve the cord over 4 to 5 vertebral body segments (Fig. 10.7). Lupus of the cord may show dramatic improvement on MR after corticosteroids. Multiple sclerosis plaques, in contrast, represent areas of focal myelin destruction, and although the symptoms improve with corticosteroids, the MR findings may improve less dramatically.
Rheumatoid arthritis (RA) is another “collagen-vascular” disease that can compromise the spinal cord, although the mechanisms are different. Focal inflammatory changes termed “pannus” destroy the transverse ligament of C1, allowing the odontoid to slide posteriorly relative to C1, and compressing the cord, particularly in flexion (Fig. 10.8B). Therefore, the neurologic injury in RA is due to atlantoaxial (A-A) instability rather a primary intramedullary lesion. The instability leads to intermittent cord compression, which in time leads to myelomalacia. Sixty percent of RA patients have cervical spine findings, and frank A-A instability is seen in 5%. Patients with spinal RA typically will have involvement in their hands and elsewhere. This is a useful discriminator, as a soft-tissue mass at the C1–C2 articulation with instability does not necessarily imply RA. A fibrous pseudotumor may occur in the same location with os odontoideum, and can develop in response to any chronically unstable spinal anatomy, including an ununited Type 1 dens fracture.
Radiation myelitis is similar to radiation injury to the brain: peak incidence occurs roughly 6 to 12 months after initial treatment, with affected areas demonstrating increased signal intensity on T2WI, with variable enhancement (Fig. 10.9). Radiation myelitis can lead to paralysis, and fear of this complication is often the limiting factor in radiotherapy for vertebral body metastases. Radiation has a very characteristic effect on the vertebral bodies. The normal erythropoietic marrow is destroyed and replaced by fat, making the vertebrae homogeneously bright on T1WI (Figs. 10.9, 10.10). In growing children, the vertebrae may show stunted growth due radiation injury to the their epiphyses (Fig. 10.10).
Acute viral illnesses are associated with myelitis in a number of ways, some of which are well described, and others, which are still poorly understood. The poliovirus causes direct injury to the anterior horn cells. Herpes zoster is invisible to imaging when latent, but cord swelling and enhancement have been reported with acute “shingles” outbreaks, appearing at spinal levels corresponding to the dermatologic outbreak. Measles provokes an autoimmune reaction that can damage the cord, which has been studied experimentally as a model for MS, and is termed subacute sclerosing panencephalitis. ADEM (see Chapter 7) is a monophasic postviral syndrome, which also affects spinal cord as its name, “encephalomyelitis,” suggests.
These patients typically have sudden high fevers, (presumably viral in origin), followed within four weeks by rapid onset of motor, sensory, and usually autonomic dysfunction, referable to a specific spinal cord level. Many neurologists reserve the catchall term “transverse myelitis” for this clinical presentation, which may be due to an autoimmune process. The imaging findings typically are a focal area of cord swelling with high signal on T2WI, with variable enhancement. It is difficult not to draw comparisons with Guillain–Barré syndrome (aka acute inflammatory polyradiculoneuropathy—unfortunately the modern name is more difficult than the classic eponym!) By whatever name, this is a progressive ascending motor weakness that affects more than one limb, but involves peripheral nerves rather than the spinal cord. Guillain-Barré has been seen after vaccinations, and evolves over a maximum of 4 weeks. Often the spinal nerves enhance, (Fig. 10.11), although this finding of is nonspecific, and can be seen in infections and neoplasms involving the CSF pathways, and even is present occasionally in disc disease.
Myelopathy is seen in AIDS patients, with vacuolar changes in the spinal cord. This appears to be a direct effect of the HIV virus itself, rather than concomitant infections, or a post infectious syndrome. The role of MR in AIDS myelopathy is more to exclude other treatable conditions, such as unsuspected cord compression, than to make a highly specific diagnosis.
Neurosarcoidosis. Inflammatory conditions involving pia and arachnoid have a similar differential diagnosis whether they involve cerebral or spinal leptomeninges. A classic example is
neurosarcoidosis, which can present as diffuse leptomeningeal granulomatous nodules, which typically enhance (Fig. 10.12). This appearance is similar to that of carcinomatous (see Fig. 10.35) and mycobacterial meningitis (see Fig. 10.20), and the distinction must be made on clinical grounds. Sarcoid can also present with intramedullary or even vertebral body granulomatous changes.
neurosarcoidosis, which can present as diffuse leptomeningeal granulomatous nodules, which typically enhance (Fig. 10.12). This appearance is similar to that of carcinomatous (see Fig. 10.35) and mycobacterial meningitis (see Fig. 10.20), and the distinction must be made on clinical grounds. Sarcoid can also present with intramedullary or even vertebral body granulomatous changes.
Arachnoiditis. One “physical agent” that causes leptomeningeal irritation is Pantopaque, which was once used in myelography. Keep this complication in mind in patients whose plain spine films show the telltale pearly white beads of residual Pantopaque. On MR, these droplets appear bright on T1WI because of their lipid content. Soon (happily) such cases will appear in teaching files only.
Today, the most common causes of arachnoiditis remain iatrogenic, including inflammation after spine surgery, spinal anesthesia, or spine “injection” procedures such as epidural nerve blocks. In arachnoiditis, the normally free-layering lumbar roots become adherent to each other, or to the peripheral wall of the thecal sac, giving the sac a “bald” appearance on myelography or T2WI images.
The remaining list of “inflammatory” conditions of the spinal cord is long, and parallels the differential considerations in the brain. Chemotherapy and other toxins, metabolic disorders, electrical burns, and lightning are physical factors that can injure the cord. Much of the damage that occurs in spinal cord trauma is not due to the mechanical forces, but the inflammatory reaction that follows. Deficiencies in vitamins such as B12 and folate, while not strictly inflammatory, can cause degeneration of the posterior columns.
Infection
Infections involving the spine can be classified according to the causative organism, or according to their anatomic location. Both approaches are useful. Certain infections, such as pediatric pyogenic meningitis, are so dramatic in their presentation that there is little need for imaging, with emergent lumbar puncture and CSF analysis being the cornerstone of diagnosis. Other processes, such as fungal osteomyelitis in the immunocompromised cancer patient, can be difficult to distinguish from metastatic infiltration or mild compression fracture (13,14,15,16). Evaluation of the pathologic vertebral body is a constant challenge, and the many “rules of thumb” sprinkled throughout this chapter are summarized in Table 10.3.
In most spine infections, the organism is seeded via the arterial route, although bacteria may reach the lower spine through Batson venous plexus. As with most bacteremia, the source of the organism is usually the skin, or GI, GU, and respiratory tracts. Exceptions are children with spinal dysraphism, or immediate postoperative patients, where a direct portal for infection exists.
Osteomyelitis/Discitis. In adults, the disc itself has a relatively poor blood supply, so primary infection is rare. In children, however, arteries penetrate the growing disc, providing a direct route for hematogenous primary infection. Once these vessels have involutes, the most common spinal site of hematogenous infectious “seeding” is the vertebral body, particularly the portions near the end plates, which have the richest blood supply. Vertebral osteomyelitis then develops (Fig. 10.13), with loss marrow signal on T1WI and end plate definition. As pyogenic infection breaks through the end plate into the disc, discitis ensues, with inevitable infection of the adjacent vertebral body. This creates an osteomyelitis/discitis complex that has been termed “pyogenic spondylodiscitis” (Fig. 10.14). This pattern is highly suggestive of infection, and unusual with neoplasms (Table 10.3). The epidural space can also be seeded hematogenously, but more often is involved by direct extension. Once the disc and epidural space are involved, extension into the paraspinous soft tissues, such as the psoas muscle, often occurs.
Epidural Abscess. Many epidural infections do not have the well-encapsulated “liquid” collections we associate with abscesses elsewhere in the body, and technically are better termed “epidural phlegmon.” The dura presents a relative barrier to infection, so infections tend to spread in a craniocaudal fashion within the epidural space, extending as many as three to four interspaces away from the vertebral abnormality, which is unusual with neoplasms (Table 10.3). Epidural abscesses have little room to expand axially, given the confines of the spinal canal, and can lead to cord compression (Fig. 10.15).
Table 10.3 Imaging Evaluation of the Pathologically Collapsed Vertebral Body | ||||||||||||||||||||||||||||
---|---|---|---|---|---|---|---|---|---|---|---|---|---|---|---|---|---|---|---|---|---|---|---|---|---|---|---|---|
|
Subdural empyemas are rare and tend to be associated with surgery or other violation of the dura. This is fortunate, as subdural infections could rapidly spread through the arachnoid layer, resulting in meningitis. Infection of the subarachnoid space is termed “meningitis,” whether it is in the brain or spinal canal. Indeed, the theory of a lumbar puncture is that there is continuous mixing of the CSF, with the fluid in the lumbar recesses being representative of the fluid bathing the brain. The clinical presentation of meningitis is discussed in detail in Chapter 6.
Meningitis typically is due to direct hematogenous seeding of the CSF, rather than contiguous spread of adjacent vertebral infection, unless there is a disruption of the leptomeninges on a congenital or acquired basis. Postcontrast MR is the most sensitive imaging examination for meningitis in both the brain and the spine, but the finding of leptomeningeal enhancement often appears relatively late in the infection’s course, and sometimes not at all. Therefore, a negative enhanced MR does not exclude meningitis and should never delay or be a substitute for a lumbar puncture.
Spinal cord abscesses are rare and are usually the result of direct seeding of the cord from overwhelming sepsis. MR allows a direct look inside the cord in these patients. Spinal cord pyogenic abscesses, not surprisingly, appear similar to those in the brain: bright on T2WI, with rim enhancement (Fig. 10.16).
Conventional radiographs cannot identify a spinal infection unless some disc or bone destruction has occurred, which may take 4 to 8 weeks, with the earliest sign being erosion of the vertebral end plates. Since older patients often have significant loss of vertebral body and disc height because of degenerative processes, evaluation of plain films in the setting of suspected infection is difficult, even after months of symptoms. Late in the infectious course, the end plates may become sclerotic bone as healing occurs, sometimes leading to fusion across the obliterated disc space. Radionuclide bone scans can turn positive in infection far sooner than plain films, but suffer from the same ambiguity: degenerative and nondegenerative processes can look the same. Indium-labeled white cell studies and gallium scans are more specific for infection, but relatively insensitive for small foci of vertebral osteomyelitis.
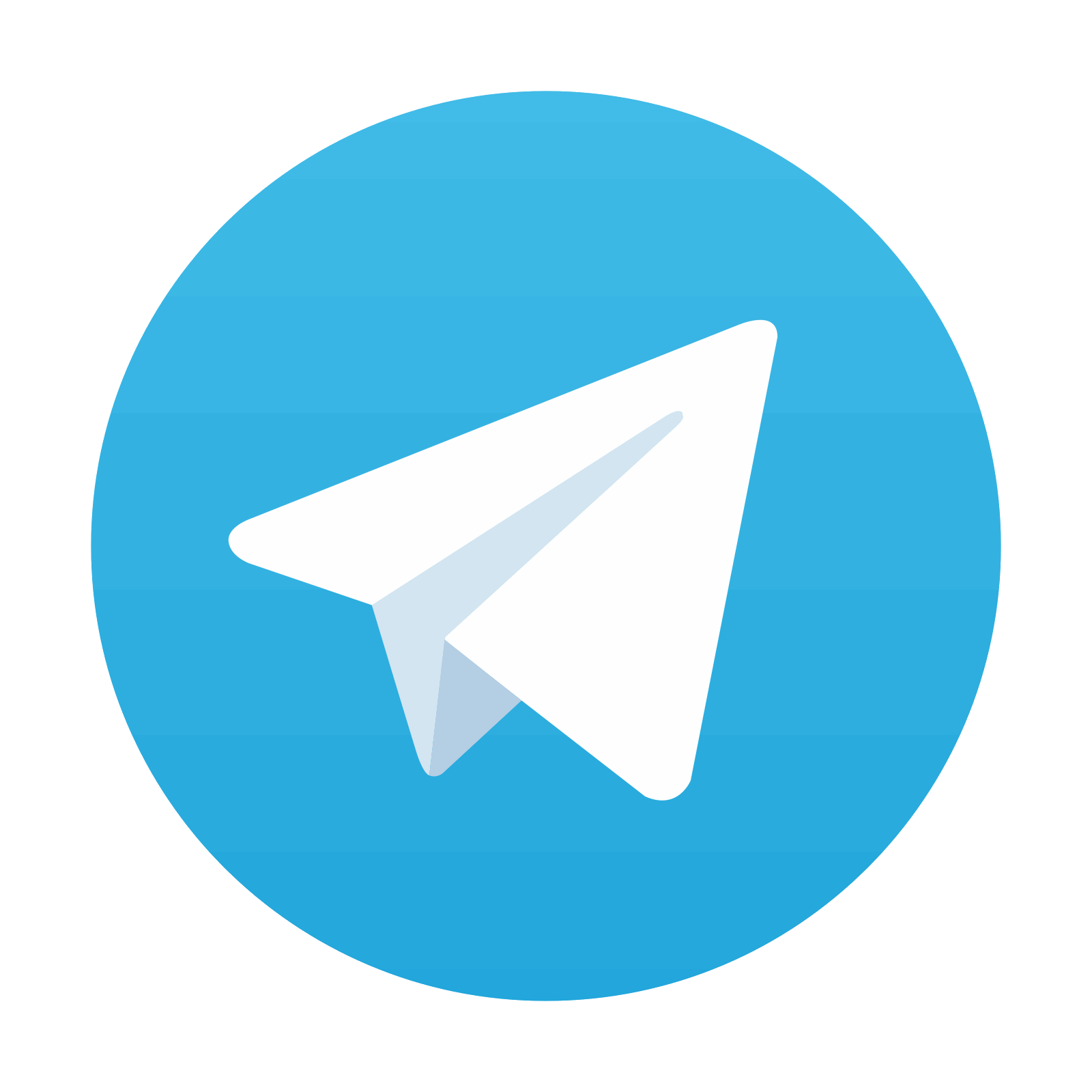
Stay updated, free articles. Join our Telegram channel

Full access? Get Clinical Tree
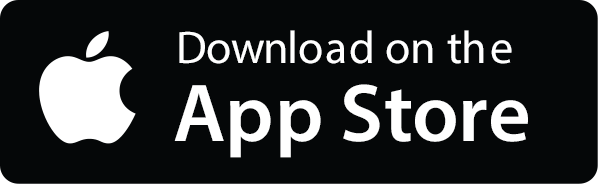
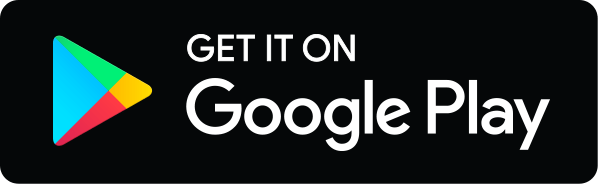