Abstract
Ultrasound is generally regarded as safe but should be regarded with respect similar to other imaging modalities. As low as reasonably achievable techniques should be used. The temperature index and mechanical index should be monitored and kept at the lowest level possible. For example, Doppler evaluation should be avoided when imaging an embryo. Recommendations for exposure time and power output for different patients have been established by ultrasound organizations.
Infection control in ultrasound is important, and strict standards must be followed to avoid transmission of infective diseases because viruses and bacteria may persist on transducers and machines.
Keywords
Bioeffects of ultrasound, disinfection of transducers, infection, mechanical index, thermal index
Bioeffects of Ultrasound
The benefit of diagnostic ultrasound as an imaging modality in clinical practice cannot be overstated. Avoiding the radiation of radiography and computed tomography and the restrictive nature of magnetic resonance imaging coupled with the portability of ultrasound machines make ultrasound an attractive diagnostic tool. Although there are no known deleterious effects in humans when used within normal ranges, knowledge of potential bioeffects of ultrasound is important to avoid improper use, especially with newer techniques and equipment. Imaging professionals should have adequate knowledge of the potential bioeffects and methods to reduce risk to the patient, especially fetal and neonatal patients. This chapter reviews the known and theorized bioeffects of ultrasound and presents current guidelines for safe practice.
Ultrasonic waves deposit energy in the tissues with transmission. Tissues also undergo compression and rarefaction under the mechanical force of ultrasound ( Fig. 21.1 ). Whether or not the energy or waves cause deleterious outcomes to the cells depends on the characteristics of the ultrasound beam and time involved. Data have shown increased caspase levels in cells exposed to ultrasound, intimating an activation of an apoptotic pathway, although this has not been confirmed in the human model in the normal diagnostic range of output. The specific bioeffects of diagnostic ultrasound in the animal model have been examined for many years, through direct evaluation as well as applying results from other modalities.
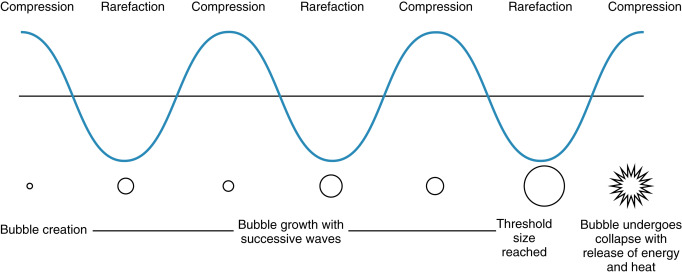
Bioeffects
There are two main bioeffects of ultrasound: thermal effects, resulting in localized tissue heating, and mechanical effects, resulting in tissue distortion and disruption. Another theoretical bioeffect is radiation force, although this is believed to be a minor effect in the output range of diagnostic ultrasound.
Thermal Bioeffects
Sound waves should be viewed as waves with energy. These waves lose amplitude as they pass through tissue by absorption and scatter, which leads to localized tissue heating. This localized increase in heat, if great enough, is presumed to create hydrogen peroxide, a free radical, which can create DNA strand breaks leading to cell death. Mild temperature increases have been shown to accelerate cellular processes without cellular detriment, but moderate increases in temperature can arrest or retard cellular division.
Tissue characteristics play a large role in temperature modulation. In regions of high blood flow, the blood acts as a heat sink and thus temperature increase is attenuated. In regions of poor blood flow, temperature increase is greatest. Due to low perfusion, the globe is particularly sensitive to ultrasound and thus output in ophthalmic ultrasound is limited to 50 mW/cm 2 . Although ophthalmic ultrasound is routinely performed on pediatric and adult patients, ophthalmic ultrasound on a fetus should not be performed unless absolutely necessary.
There are many other modifying factors, including body habitus, perfusion, distance to the transducer, bone, and fluid presence. The first 5 mm of tissue experiences the greatest temperature increase as the sound wave loses energy. This effect is amplified with high-frequency ultrasound, which is common for superficial evaluations.
Different tissues absorb energy at different rates. Soft tissues such as adipose and muscle absorb less heat than bone and cartilage. Bone quickly absorbs energy resulting in heat.
There are many documented effects of temperature increase in fetal animal model studies, including neural tube defects, microphthalmia, cataracts, microcephaly, and functional and behavioral problems. Hyperthermia is also a proven teratogen in animals and is considered teratogenic in humans. None of these congenital abnormalities have been shown to be caused by diagnostic ultrasound.
In the animal model, significant temperature increases of more than 4°C for more than 5 minutes have been shown to cause developmental and congenital abnormalities. This temperature increase is much greater than expected in human diagnostic ultrasound.
There has been no documented adverse effect on an embryo or fetus of an increase of less than 1.5°C in maternal temperature. An increase of 2.5–5.0°C can occur with 1 hour of ultrasound exposure. Effects in nonfetal ultrasound likely require an increase of 18°C for at least 0.1 seconds.
Data from a study on effects of maternal fever on fetal development demonstrate an association of maternal fever exceeding 38.3°C with increased fetal abdominal wall defects. Although maternal fever is a systemic process affecting the entire fetus, it is postulated that an increase in temperature via ultrasound could result in similar effects.
Mechanical
The main mechanical bioeffect of ultrasound is cavitation. This occurs when gas bubbles or voids are trapped in tissue and, under the effects of alternating compression and rarefaction of the acoustic waves of ultrasound, increase in size until the energy reaches a threshold during compression, and the bubble violently collapses resulting in localized release of energy with associated potential for injury ( Fig. 21.2 ). This process can occur where there are preexisting gas bubbles, such as within the lung and bowel. Lung hemorrhage has been observed in the animal model following ultrasound exposure. Cavitation is also the process of creating gas bubbles within tissue, which can then go on to cavitate. Although this is the desired outcome in new ultrasonic fat removal techniques, it is to be avoided in diagnostic ultrasound.
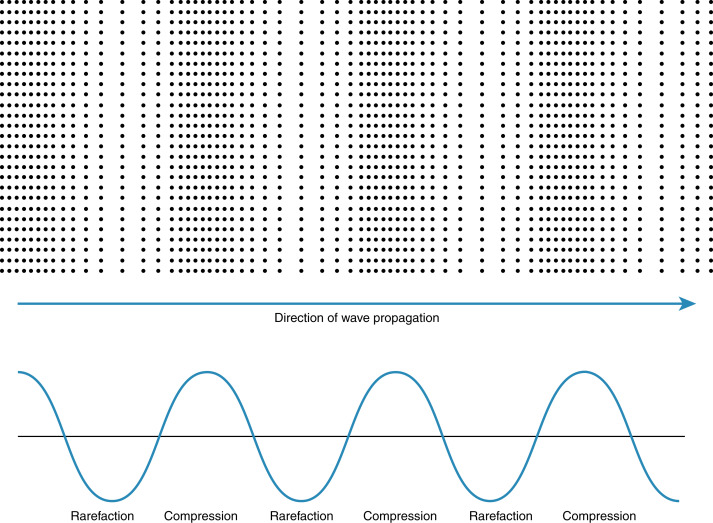
There are two types of mechanical cavitary effects, inertial/transient and noninertial. Inertial/transient effects result in cavitation, whereas noninertial effects result in only oscillation of the formed or preexisting gas bubbles. Noninertial mechanical effects are less likely to produce adverse bioeffects.
Ultrasound waves should be considered as high-pressure shock waves; when these waves produce cavitation, they release energy and heat as the gas bubble collapses. This localized release in energy and heat is believed to result in tissue damage.
Cavitation due to diagnostic ultrasound has not been documented in mammalian fetuses, presumably due to the lack of a gas-fluid interface, although theoretically, sustained high-powered ultrasound in a localized area could create gas bubbles that could subsequently cavitate. It should be noted that current epidemiologic data do not link fetal ultrasound with nonthermal adverse bioeffects. All of the adverse bioeffects in mammalian fetuses have been related to temperature effects.
The other ultrasound-induced mechanical bioeffects are radiation force and acoustic streaming. Radiation force is produced as the acoustic energy of the ultrasonic wave is absorbed along the path of the beam. As the ultrasound transducer produces pulses of ultrasonic waves, a pulsatile force is created. The result of the force can be seen in streaming debris within cysts.
Acoustic Output and Indices
The acoustic output limit from diagnostic ultrasound equipment from 1976 to 1992 was restricted to 720 mW/cm 2 for peripheral vascular, 430 mW/cm 2 for cardiac, 94 mW/cm 2 for fetal and other, and 17 mW/cm 2 for ophthalmic applications. In 1992, the US Food and Drug Administration (FDA), under pressure from manufacturers and clinicians, raised the limit to 720 mW/cm 2 for all applications except ophthalmic (50 mW/cm 2 ). With the increased limit came a need for imaging professionals to know, in real time, the potential for bioeffects. The FDA developed a regulation known as the Standard for Real-Time Display of Thermal and Mechanical Acoustic Output Indices on Diagnostic Ultrasound Equipment, also known as the output display standard. This standard mandated that the thermal and mechanical indices be displayed on ultrasound equipment capable of outputs up to the FDA limit.
Thermal Index
Currently, the thermal index (TI) is used to measure the potential thermal bioeffects of ultrasound. The thermal index is the ratio of emitted acoustic power to the power required to raise tissue temperature by 1°C. A larger TI indicates a higher heating potential. This index was intended to estimate the magnitude of temperature increase after an exposure to ultrasound. Unfortunately, this is a general index and does not take into account the duration of exam.
There are three display options for the TI:
- 1.
TIS, soft tissue: This option assumes that the beam impinges only on soft tissue; consider first trimester ultrasound examinations up to 10 weeks. After 10 weeks, the sonographer should change the machine settings.
- 2.
TIB, bone: This option assumes that the beam impinges on bone at or near its focus; consider second and third trimester ultrasound examinations.
- 3.
TIC, cranial bone: This option assumes that the beam impinges on bone of the cranium in the neonate, pediatric, or adult patient in transcranial ultrasound.
Mechanical Index
The mechanical index (MI) is meant to be an indicator of the potential for nonthermal bioeffects. The index is calculated as the maximum value of peak negative pressure divided by the square root of the acoustic center frequency. A larger MI indicates a higher potential for nonthermal bioeffects. There is a higher potential for damage above an MI of 0.4, particularly in organs such as the lung or intestines, which have surface gas bubbles.
Bioeffects of Ultrasound Modes
To understand the differing levels of potential bioeffects of the various ultrasound modes, an understanding of the physics of the specific mode is necessary.
B-mode, or brightness modulation or two-dimensional mode, produces a two-dimensional image using a linear array of transducers scanning simultaneously. This is the most commonly used ultrasound noted.
M-mode (motion mode) is not a Doppler-based ultrasound; instead, it is a real-time grayscale mode that uses high-frequency pulses in succession to evaluate movement of focal structures over time, predominantly for fetal cardiac imaging.
Doppler mode ultrasound uses the Doppler effect to visualize and measure flow away from and toward the transducer. Color Doppler overlays color-coded Doppler data on B-mode images. Continuous Doppler displays flow along a single line using a segmented transducer to allow continuous transmission and receipt of the Doppler signal. Pulsed-wave Doppler acquires the Doppler signal from a small volume designated by the sonographer. To localize the Doppler signal, there is a small delay between transmission and receipt of the Doppler signal.
Bioeffects are greatest with pulsed Doppler, followed by power and color Doppler, and finally B-mode and M-mode. Effects are additionally amplified with pulsed-wave Doppler because of sustained scanning in a relatively small focal area.
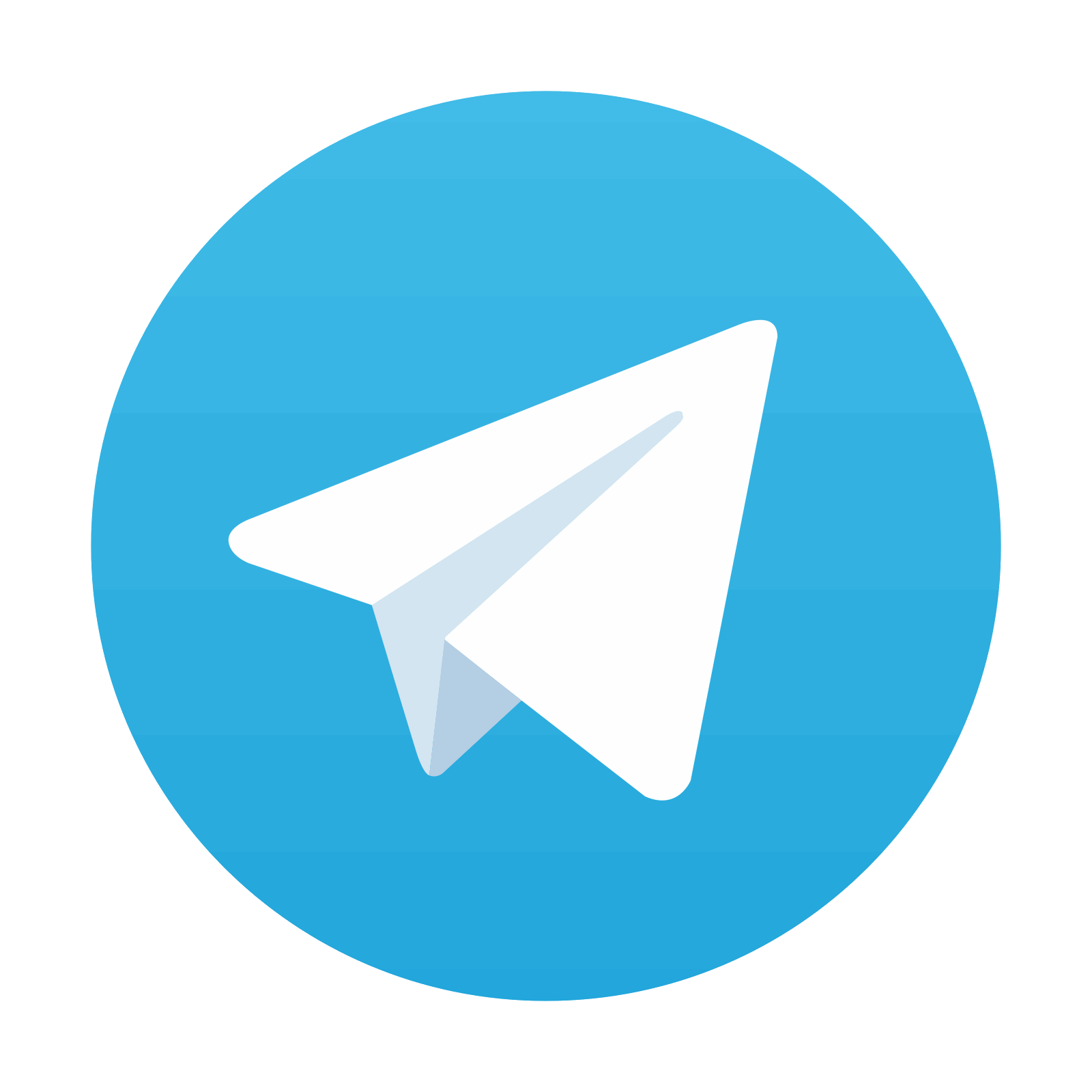
Stay updated, free articles. Join our Telegram channel

Full access? Get Clinical Tree
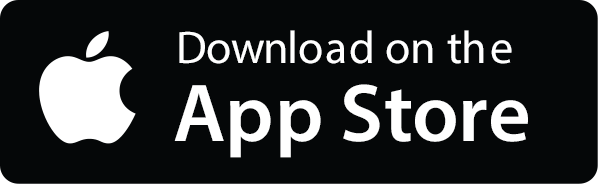
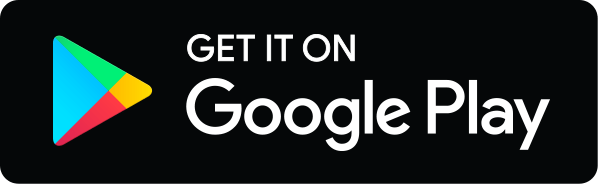