, Bakhos A. Tannous3, 2 and Xandra O. Breakefield1, 4, 2
(1)
Department of Neurology, Neuroscience Center, Massachusetts General Hospital, Boston, MA, USA
(2)
Program in Neuroscience, Harvard Medical School, Boston, MA, USA
(3)
Experimental Therapeutics and Molecular Imaging Laboratory, Department of Neurology, Neuroscience Center, Massachusetts General Hospital, Boston, MA, USA
(4)
Department of Radiology, Center for Molecular Imaging Research, Massachusetts General Hospital, Boston, MA, USA
Abstract
Extracellular vesicles (EVs) including exosomes and microvesicles are nanometer-sized vesicles released by cells to deliver lipids, cellular proteins, mRNAs, and noncoding RNAs, thereby facilitating intercellular communication without direct cell-to-cell contacts. Due to their nanoscale size, EVs have been visualized under microscopy in vitro. We here describe a strategy to label EVs with Gaussia luciferase for noninvasive bioluminescence imaging and monitoring of systemically administered EVs in vivo.
Key words
Extracellular vesiclesMicrovesiclesExosomes Gaussia luciferaseBioluminescenceCoelenterazine1 Introduction
Cells release various types of extracelluar vesicles (EVs) ranging from 40 to 4,000 nm in diameter, which are capable of transporting lipids, DNAs, RNAs, and proteins to recipient cells. These vesicles include exosomes (40–100 nm), microvesicles (100–1,000 nm), and apoptotic bodies (50–4,000 nm) [1, 2]. Since EVs are diffuse within the extracellular matrix and taken up by cells, they can mediate intercellular communication between neighboring cells, as well as cells at distal sites [3–9]. Importantly, cancer cells were found to produce an abundant amount of EVs containing a select subset of cellular proteins, mRNAs and noncoding RNAs, thereby modulating normal cells in their microenvironment to promote angiogenesis and tumor progression [10–20]. On the other hand, since EVs are small and poorly immunogenic, several recent studies have engineered EVs to shield therapeutic genes/drugs for targeted delivery to tumors, even overcoming biological barriers such as the blood–brain barrier [21–26]. Until recently, EVs have only been visualized under high-resolution microscopy due to their nanometer size, and localizing EVs in vivo has been largely unachievable [27].
Photinus pyralis luciferase (firefly; Fluc), Renilla reniformis luciferase (sea pansy; Rluc), and Gaussia princeps luciferase (marine copepod; Gluc) are among the most commonly used luciferases in biomedical research [28]. Unlike reporters conjugated to fluorescent proteins, such as CD63-GFP for EVs [19, 29], luciferase-based reporters do not need an excitation source for their subsequent light emission. Instead, luciferases emit bioluminescence via conversion of their respective substrate with either ATP and Mg2+ (Fluc; D-luciferin), or oxygen alone (Rluc and Gluc; coelenterazine). This makes luciferase an ideal reporter for in vivo studies where specialized equipment for excitation of reporter proteins is not required.
Gluc is unique from other luciferases such that it is naturally secreted and capable of emitting flash bioluminescence (480 nm peak) that is over 1,000-fold more sensitive than Rluc and Fluc [30]. Combining these features and the fact that Gluc only requires oxygen as its cofactor for bioluminescence reaction, we engineered a membrane-bound (mb) variant of Gluc fused to a biotin acceptor peptide (BAP), termed mbGluc-BAP [31], for EV labeling. By overexpressing mbGluc-BAP in human embryonic kidney (HEK) 293T cells followed by EV isolation, one can successfully track systemically administered EVs in athymic nude mice via in vivo bioluminescence imaging or by ex vivo analysis of tissues.
2 Materials
2.1 EV Reporter Construct and Donor Cells
1.
EV reporter construct: pCSCW2-mbGluc-BAP-IRES-GFP, a lentivirus vector encoding Gluc fused to the transmembrane domain of platelet-derived growth factor receptor (PDGFR) and a biotin acceptor protein (mbGluc-BAP) as well as GFP, separated by an internal ribosomal entry site (IRES), all under the control of the constitutively active cytomegalovirus (CMV) promoter [32].
2.
EV donor cells: Human embryonic kidney 293T (293T) cells obtained from American Type Culture Collection (ATCC; Manassas, VA, USA). These cells are cultured in Dulbecco’s modified Eagle’s medium (DMEM) supplemented with 10 % fetal bovine serum (FBS), 100 U/ml penicillin, and 0.1 mg/ml streptomycin at 37 °C and 5 % CO2.
3.
Filter: 0.22 μm polyethersulfone filter (EMD Millipore, Billerica, MA, USA).
4.
EV-depleted FBS: Using ultracentrifugation equipment described (see Subheading 2.2, items 1–3), centrifuge FBS at 100,000 × g for at least 16 h at 4 °C. Collect supernatant (EV-depleted FBS) and filter it through a 0.22 μm filter to further minimize EV contamination of the FBS. Aliquot EV-depleted FBS into 50 ml canonical tubes and store at −80 °C.
5.
EV-isolation medium: Supplement DMEM with prepared 10 % EV-depleted FBS, 100 U/ml penicillin and 0.1 mg/ml streptomycin.
6.
Polybrene: 10 mg/ml in tissue culture grade water.
7.
Epifluorescence microscope capable of detecting GFP expression.
2.2 EV Isolation Components
1.
Ultracentrifuge: Optima L-90K (Beckman-Coulter, Indianapolis, IN, USA).
2.
Rotor: Type 70Ti rotor (Beckman-Coulter).
3.
Ultracentrifuge tubes, seal former, tube sealer: Quick-Seal® tubes (Cat#: 342413, Beckman-Coulter), seal former and a tube sealer (Beckman-Coulter).
4.
Filter: 0.22 μm polyethersulfone filter (EMD Millipore).
5.
60 ml syringes.
6.
Double filtered phosphate buffered saline (PBS): 8 g/L NaCl, 0.2 g/L KCl, 1.78 g/L Na2HPO4∙2 H2O, 0.27 g/L KH2PO4, adjust to pH 7.4 and filter twice through a 0.22 μm filter.
2.3 Gluc Activity Assay
1.
A luminometer such as the Dynex MLX Microtiter Plate Luminometer (Vienna, VA, USA).
2.
96-well white or black microtiter plates.
3.
Coelenterazine (CTZ), the Gluc substrate: Reconstitute CTZ to a stock concentration of 5 mg/ml with methanol pre-supplemented with a few drops of HCl to minimize CTZ oxidation and precipitation. Aliquot CTZ and store at −20 °C. Pre-warm CTZ to room temperature before use and protect from light.
2.4 In Vivo Bioluminescence Imaging
1.
Animals: Immunodeficient athymic nude mice or any other mouse strain of choice.
2.
An optical imaging system such as the IVIS® Spectrum connected to XGI-8 Anesthesia System (Perkin-Elmer, Waltham, MA, USA).
3.
Isoflurane (Baxter, Deerfield, IL, USA).
4.
XGI-8 Anesthesia System (Perkin-Elmer).
5.
Sterile PBS: Autoclaved or 0.22 μm filtered PBS.
6.
Coelenterazine: prepare as described in item 3 in Subheading 2.3. Alternatively, for more stable Gluc signal during imaging, prepare fresh CTZ before each injection according to “Inject-A-Lume” kit manual (Nanolight, Pinetop, AZ, USA).
7.
1.5 ml microcentrifuge tubes.
8.
Insulin syringe needles: 29½ gauge, 0.3 ml.
9.
Heating device: 43 °C water in a 500 ml beaker to induce vasodilation of tail veins.
3 Methods
3.1 Generate EV Donor Cells
1.
Seed 0.5 × 106 HEK 293T cells per well in fresh culturing medium in a 6-well tissue culture plate (34.8 mm diameter).
2.
When 60–70 % confluence is reached, transduce the cells with lentiviral vectors encoding mbGluc-BAP-IRES-GFP in 3 ml medium supplemented with 1 μl polybrene (10 mg/ml) for each well (see Note 1).
3.
48 h post-transduction, examine GFP reporter expression under an epifluorescence microscope to determine transduction efficiency. At least 80–90 % of cells should be transduced to generate sufficient EVs labeled with mbGluc-BAP (see Note 2).
3.2 EV Isolation and Gluc Activity Assay
1.
Add 2 × 106 of 293T cells expressing mbGluc-BAP per 150 mm plate in fresh medium.
2.
When the cells reach approximately 50 % confluence, gently aspirate the growth medium and replace with 20 ml of fresh EV-isolation medium.
3.
Incubate the cells for another 48 h at 37 °C, 5 % CO2 to generate conditioned medium containing EVs.
4.
Collect conditioned medium in 50 ml canonical tubes and centrifuge at 300 × g for 10 min at 4 °C to remove cell debris.
5.
Collect supernatant in separate 50 ml canonical tubes and centrifuge at 2,000 × g for 10 min at 4 °C to remove additional cell debris.
6.
Prepare a 60 ml syringe attached to a 0.22 μm filter followed by a 16 gauge needle.
7.
Insert the assembled filtering syringe into a Quick-Seal® tube and filter the supernatant through the syringe (see Note 3).
8.
Weigh all supernatant-containing Quick-Seal® tubes and adjust to equal weight by removing excess supernatant as needed and seal the tubes with a sealer. Mark one side of each tube with a marker pen for later orientation purposes (Fig. 1a).
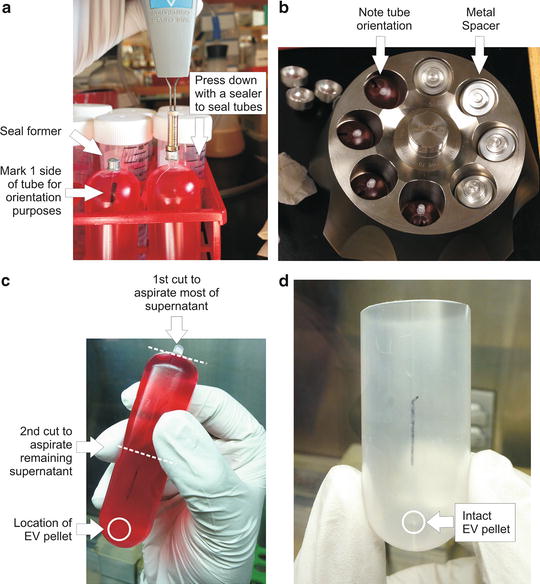
Get Clinical Tree app for offline access
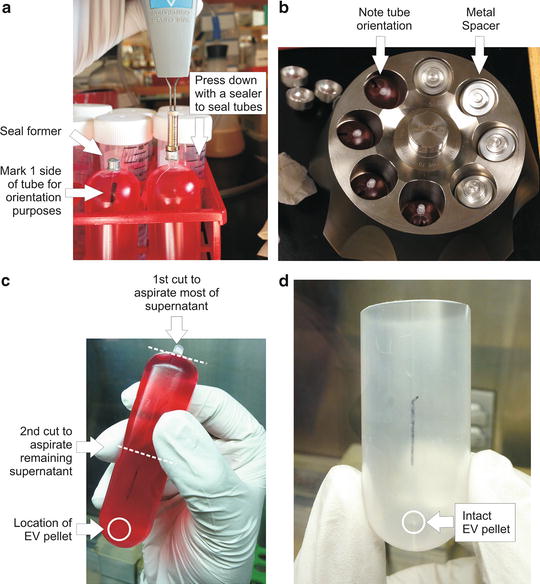
Fig. 1
EV isolation procedure. (a) Following conditioned medium collection into ultracentrifuge tubes, place a seal former onto each tube stem. Seal the tubes with a tube sealer by applying gentle, downward pressure until the seal former rests on top of the tube shoulder. Do not press the seal former beyond the tube shoulder since it will result in leakage during centrifugation. Using a permanent marker, mark one side of the tubes (both top and bottom) for orientation purposes. (b
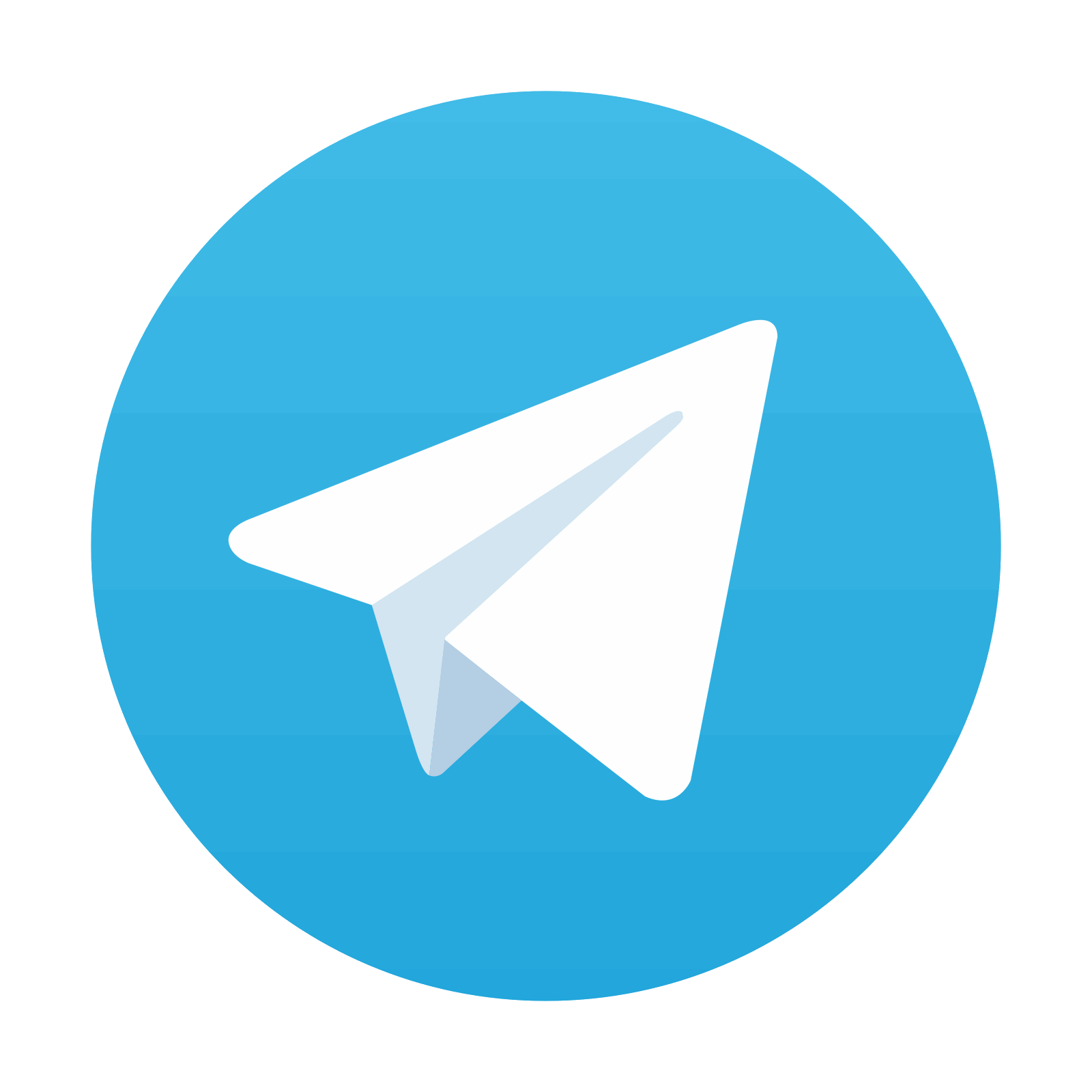
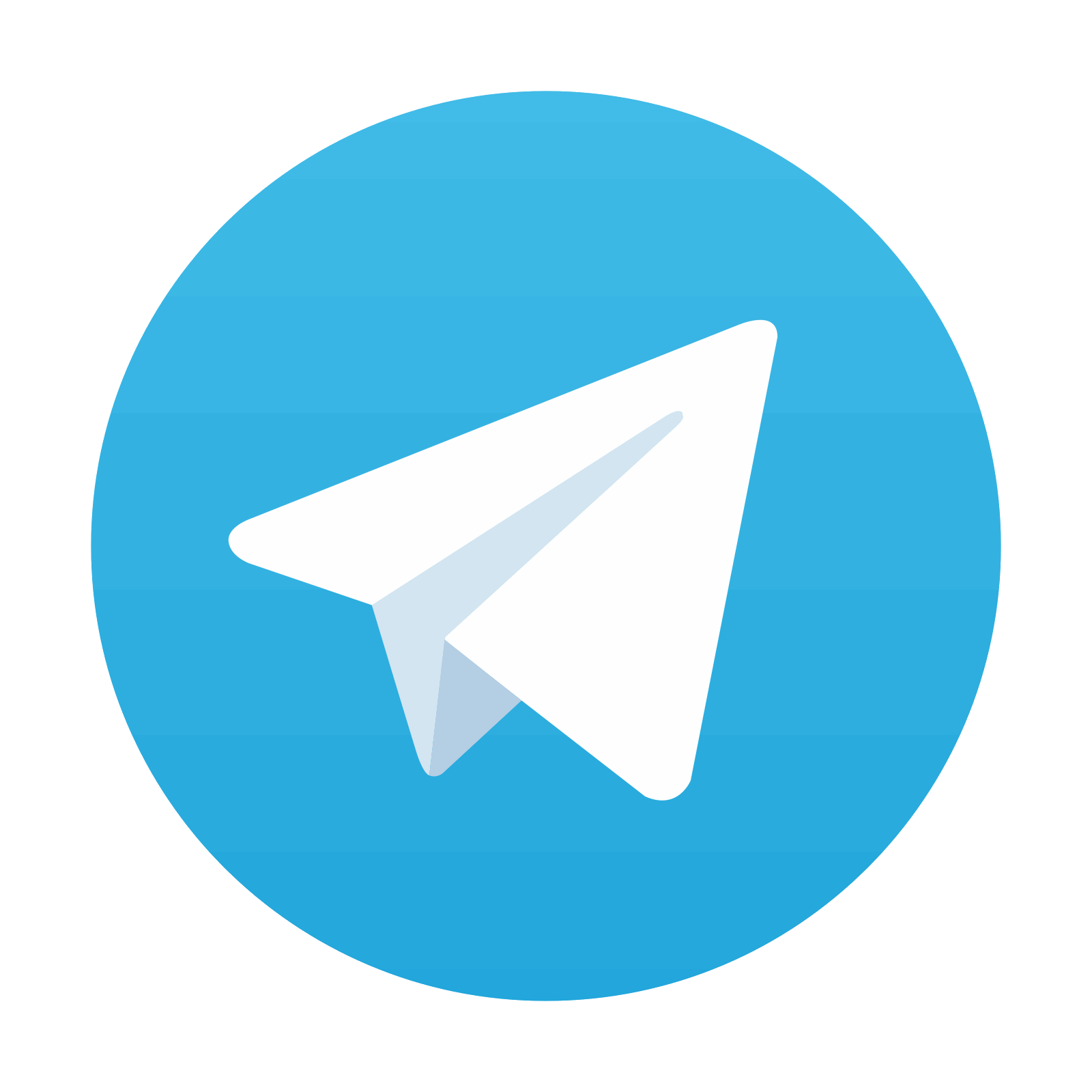
Stay updated, free articles. Join our Telegram channel

Full access? Get Clinical Tree
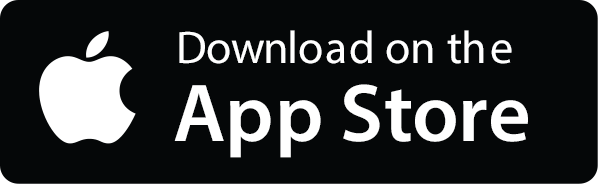
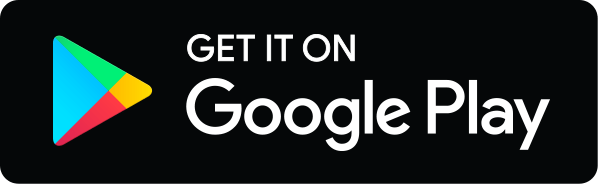
