Nuclear Cardiology Applied to Electrophysiology: Introduction
The electrophysiologist needs precise electromechanical investigation tools to quantify the electrical propagation and its impact through the heart muscle. Analysis of the timing of the myocardial contraction based on Fourier phase histograms allows mapping of the kinetics of the different ventricular segments from functional phase pictures and, by extrapolation, mapping of the electrical activity of the heart. Nuclear cardiology combines mechanical and electrical analysis in real time. In this chapter, we report on our experience of gated blood pool scintigraphy combined with phase analysis and myocardial innervation imaging in various diseases with electrical disorders.
Specific Technical Background of Nuclear Imaging in Arrhythmias
Radionuclide functional imaging of the heart addresses multiple aspects of cardiac function.1 Although most applications of nuclear cardiology are focused on coronary artery disease, in which the combination of stress/rest myocardial perfusion and/or viability imaging plays a major clinical role, many other less common cardiac diseases may benefit from this noninvasive diagnostic approach.
Perfusion, Viability, Innervation, and Mechanical Function
Perfusion and viability imaging modalities are based on cellular uptake of a radioactive molecule such as thallium-201 (201Tl), which presents an active transport comparable to that of potassium cation (K+), or sestamibi or tetrofosmin labeled with technetium 99m (99mTc), which are larger cations whose cellular penetration is passive due to concentration gradient.
Imaging is usually performed in tomographic mode (SPECT) and is a two-step procedure that combines stress imaging (shortly after physical exercise or pharmacologic coronary vasodilation) with resting imaging after stress-injected tracer redistribution with time (thallium) or a second resting administration (technetium-labeled molecules). The first set of images describes coronary perfusion reserve at stress, whereas the second set reflects cellular viability.
In addition to single photon (gamma ray) methods, positron emission tomography (PET) may also be a choice for a comparable approach, but PET uses specific positron-emitting radiopharmaceuticals such as the generator-produced rubidium-82 for perfusion and fluorine 18–labeled fluorodeoxyglucose.
Myocardial sympathetic innervation imaging is based on the presynaptic uptake of catecholamines. The gamma-emitting tracer is meta-iodobenzyl guanidine (MIBG) labeled with iodine 123. Comparable positron-emitting tracers are used as well. Image acquisition can be planar or SPECT. Innervation defects appear as hypoactive areas, which have to be compared with perfusion defects using an adequate perfusion agent.
Imaging the mechanical contraction of the heart needs a dynamic acquisition of the periodic motion of the myocardium. Electrocardiographically (R wave) triggered acquisitions can be performed on myocardial perfusion SPECT images. Another option consists of blood pool labeling using an intravascular tracer such as albumin or red blood cells, both labeled with 99mTc. Gated blood pool pictures can be acquired in planar mode as well as in SPECT, but in any case, one single cardiac cycle is unable to provide a sufficient number of counts. Therefore, a high number of successive cardiac cycles (≥100) have to be superimposed for an adequate image (Fig. 9–1). Measurement of left ventricular ejection fraction is the most common variable obtained by these studies, but as will be shown later in this chapter, several other variables can be of special interest in the context of arrhythmias.
Cardiac Cycle Selection in the Gating Process
The reliability of gating is the key to adequate imaging of cardiac contraction. In patients with a stable heart rate, superimposition of multiple cardiac cycles in electrocardiogram (ECG)-triggered synchronism is not a crucial problem. However, this is far from true in a patient population with cardiac rhythm abnormalities. More or less sophisticated technical approaches may be able to at least partially solve this problem. Their common principle consists in selecting the acquired cardiac cycles based on the R-R interval duration.
Cycle selection can be made a priori after acquiring for a given time a series of successive cardiac cycles and construction of the R-R histogram. By defining a temporal window on the desired part of the histogram, only the cycles matching inside the window will be accepted for final image acquisition. The R-R histogram may present a continuous variation around an average value (eg, in atrial fibrillation) or may show distinct peaks (eg, in extrasystoles where the average peak of sinusal cycles can be surrounded by a peak of shorter cycles—extrasystoles—and a second peak of longer cycles—postextrasystoles).
The option of “a posteriori” gating is more flexible because cardiac cycles are then acquired in “list mode,” which means without immediate superimposition. Cycle combination is performed in a second time, when acquisition has been completed, which allows changes in R-R windowing as well as multiple window acquisitions.
Planar and Three-Dimensional Right Ventricular Imaging
Diagnosis of coronary artery disease is mainly focused on the left ventricle. Perfusion tracers, as well as thallium and technetium-labeled molecules, show a very low uptake in the right ventricular wall, which is difficult to see on the images. Only in right ventricular hypertrophy can the right ventricle be clearly visualized, but it is always visualized with a lower intensity than the left ventricle. Therefore, myocardial SPECT is a limited tool for assessment of right ventricular diseases.
Blood pool imaging does not present the same limitations, and the labeling of the circulating blood allows adequate visualization of all of the heart chambers and thoracic vessels. The drawback of this global imaging of cardiac structures is the risk of superimposition of different cavities, and technical solutions have to be found in order to minimize these overlaps.
For planar imaging, the projection must be performed in the anterior oblique view with optimization of the angle for each individual patient with regard to the orientation of the interventricular septum in order to obtain the best separation between the right and left ventricles (Fig. 9–2).
The gated blood pool SPECT approach is another option for selection of the right ventricle and evaluation of its functional variables. Measurements of ejection fractions, whether left or right, presuppose proportionality of labeled blood volumes with collected gamma counts in selected regions of interest. After adequate correction for extracardiac background activity, the end-diastolic and end-systolic counts are representative of the corresponding blood volumes.
Contraction Chronology Using Temporal Fourier Analysis
The ECG gating process provides a series of images covering an average cardiac cycle over the R-R interval. The temporal course of the blood contents within the ventricles (ie, the corresponding radioactive counts) evolves from end-diastole to end-systole during the contraction phase and from end-systole again to end-diastole during the filling phase. This periodic change can be approximated by a single sinus function (Fig. 9–3
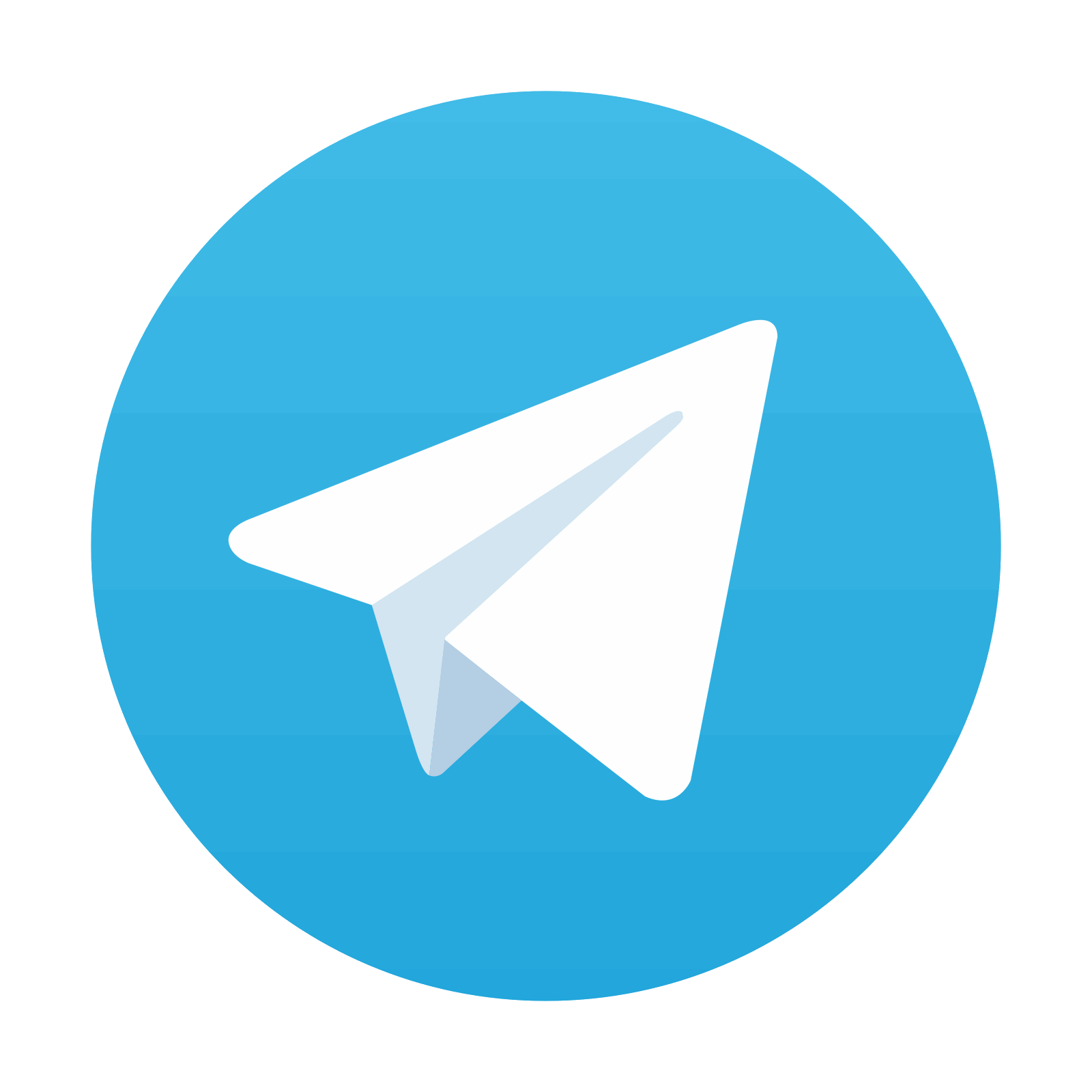
Stay updated, free articles. Join our Telegram channel

Full access? Get Clinical Tree
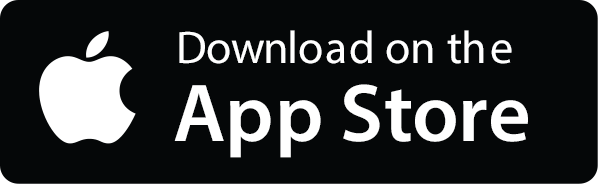
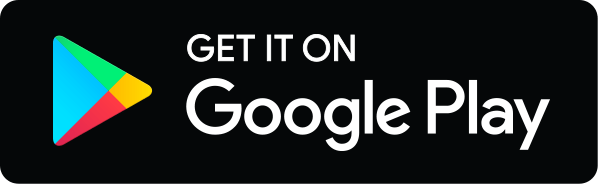
