Fig. 9.1
A 66-year-old female with bladder cancer referred for staging. PET imagery (transaxial slice, a; coronal slice, b) visualizes a focus of FDG activity at the bifurcation of the iliac vessels (arrow). Without CT fusion, such a focus is concerning for hypermetabolic metastatic adenopathy. With fusion imaging (c), the PET focus localized to the iliac vein (as artifact) on CT without IV contrast (d); further identified by a same-day CT with IV contrast (e). PET-CT fusion yields significantly higher diagnostic accuracy than PET alone, both in sensitivity and specificity. Moreover, fusion imagery has been shown to have a synergistic impact on PET and CT analyses: analysis of PET-CT fusion imagery has a higher diagnostic yield than the sum of independent PET and CT readings
Bone Scintigraphy and Osteoblastic Versus Osteolytic Lesions
Single photon-emitter technetium 99 m (99mTc)-labeled bisphosphonate scintigraphy, often referred to as the bone scan, visualizes the skeleton by a bisphosphonate, commonly methylene diphosphonate (MDP) or hydroxymethyl diphosphonate (HDP) labeled with 99mTc. Bone scintigraphy is for the detection of osseous lesions, usually by planar body imaging, with or without SPECT or SPECT-CT of selected body regions. The radiotracer localizes by chemical adsorption to the inorganic hydroxyapatite matrix of bone. Genitourinary cancer cells in bone metastases do not accumulate bisphosphonate tracer. Bisphosphonate scintigraphy detects osseous metastases indirectly by detecting the local response of a bone to a metastasis. Some GU cancers, notably prostate metastases, classically cause osteoblastic reactions that evoke a relatively high local exposure of hydroxyapatite matrix, which tracer can absorb. This manifests on scintigraphy as a bony hotspot. Osteolytic-type metastases, typical of renal and bladder cancer, disintegrate compact bone, sometimes without any osteoblastic-type bone reaction. Nonosteoblastic disease may be invisible to the bone scan. CT can detect osteolytic metastases of clinically relevant, macroscopic size, as well as radiodense-appearing osteoblastic-type metastases.
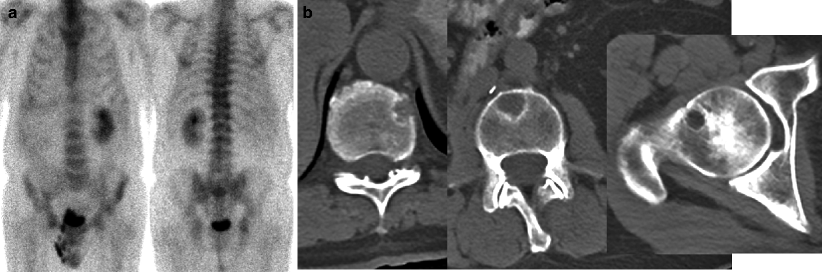
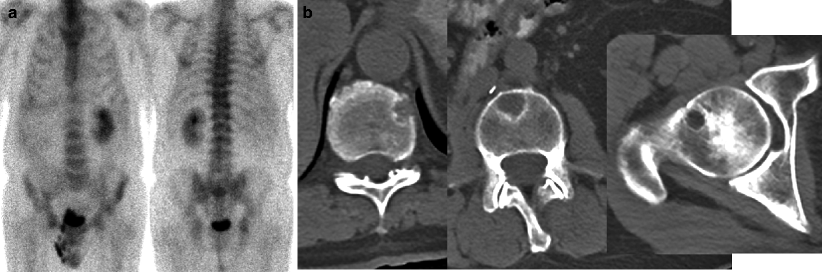
Fig. 9.2
Renal cancer patient with known metastatic disease referred for bone evaluation. Bone scintigraphy showed no osseous lesions of clear concern for a metastatic etiology (anterior planar torso projection, a; posterior projection, b). However, same-day CT study visualized multiple osteolytic metastases invisible to scintigraphy, for example, in several vertebrae (left and middle transaxial CT images in b) and right femoral neck (right transaxial CT image in b)
Radiographically Occult Osseous Metastasis
Other macroscopic intraosseous metastases may cause neither an osteoblastic-type reaction nor osteolytic damage detectable by CT. However, a radiographically occult bony reaction may be detected by bone scintigraphy. This is usually followed by changes on CT within several weeks.
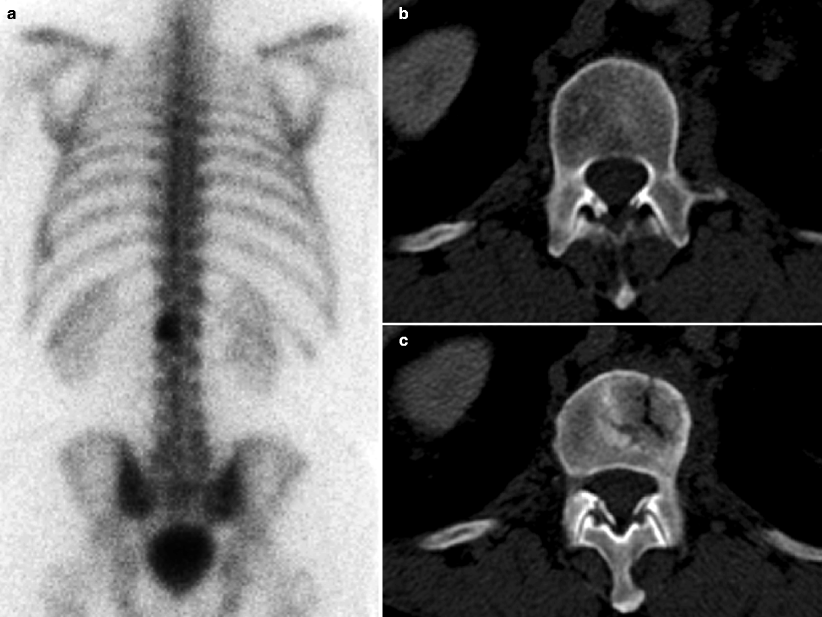
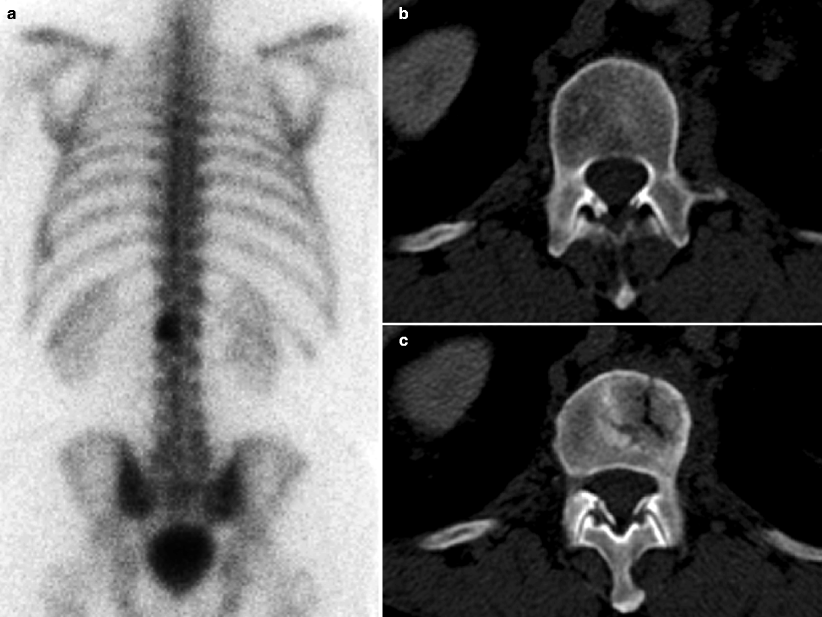
Fig. 9.3
Prostate cancer patient having follow-up bone scintigraphy during disease surveillance. Suspicious lesion of the L1 vertebra was newly visualized, scintigraphically (a), but same-day CT (transaxial slice through L1, b) showed no clear radiographic correlate of concern. Three months later, the lesion persisted on bone scintigraphy (not shown), and same-day follow-up CT (c) showed a corresponding L1 radiodense changes, with a pathological fracture. Osseous lesions may manifest on bone scintigraphy before CT changes become apparent
Flare Response Phenomenon
Other macroscopic intraosseous metastases may be present but invisible on both CT imagery and bone scintigraphy but can often be visualized by PET and/or MRI imaging. Such invisible osseous marrow metastases have an added complication: the scan flare-response phenomenon. The scan flare-response, also called pseudoprogression, is a well-documented (but probably underrecognized) biological-imaging phenomenon observed in serial imaging studies of cancer patients receiving anticancer therapy. In the scan flare-response, the effect of interim anticancer therapy induces the new visualization of osseous lesions (of metastatic etiology) that were preexistent but previously invisible in the prior study. Newly visualized osseous findings can easily be (mis-)attributed to interim development of a new osteoblastic-type osseous metastasis, when in fact metastatic cells might no longer be present in the osseous lesion (remembering that lesion is synonymous with wound and not synonymous with metastasis), possibly having been eradicated by interim therapy. Newly visualized osteoblastic-type changes may represent bony healing at the site. The 2008 guidelines of the Prostate Cancer Clinical Trials Working Group (PCCTWG) make the important recommendation that therapy ought not be discontinued, if bone scintigraphy shows new foci of osseous activity at the first (12 week) post-therapy assessment time-point but no other clear indications of disease progression are evident to avoid potentially being misled by flare response phenomenon, or pseudoprogression [1, 2]. Rather, bone scintigraphy should be repeated at least 6 weeks later. If this second post-therapy bone scan documents >2 additional, suspicious, new scintigraphic foci, then the findings are accepted as confirming disease progression [3].
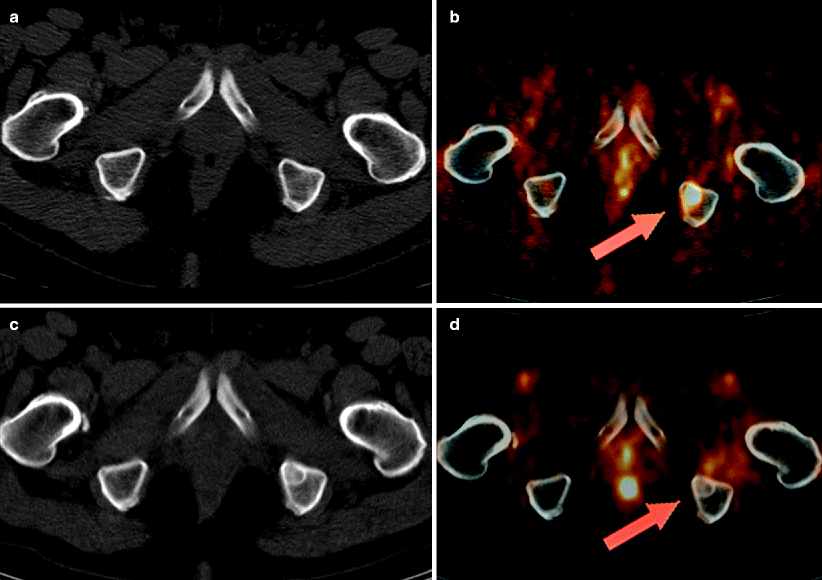
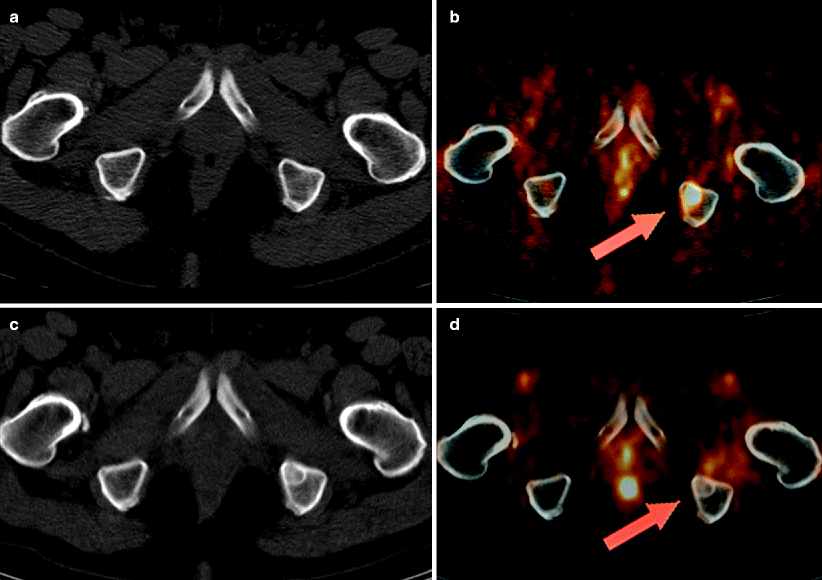
Fig. 9.4
Prostate cancer patient, with postsurgical rise in PSA, referred for imaging for restaging. (a,b) show a transaxial fusion FDG-PET-CT image (b) that visualized a previously unidentified lesion of the left ischium (red arrow) suspicious for metastatic disease. Same-day standard CT (a) showed no ischial lesion. Next-day biopsy confirmed metastatic prostate cancer in left ischium. Patient received salvage radiotherapy. (c,d) show restaging imaging, 20 months later. Standard CT (c) newly visualized a radiodense lesion of the left ischium, reported as a new osseous metastasis (though previously identified by prior PET, b). Same-day follow-up PET-CT (d) shows no residual FDG uptake in the previously identified metastatic ischial lesion, consistent with a favorable response to treatment, the newly visualized radiodense changes being consistent with bony healing (CT flare response)
Slow Healing of Osseous Lesions
During follow-up of patients with a history of osseous metastases, many imaging specialists will describe persistent lesions, on bone scintigraphy, as “metastases” in their reports, whereas others prefer phrasing such as “osseous lesions of metastatic etiology,” recognizing that bone scintigraphy does not visualize osseous metastases directly, only the osseous wounds that metastases cause. More than mere semantics, the latter phrase recognizes a fundamental limitation of bone scintigraphy: the bone scan and CT are both often unable to identify in a timely fashion bony metastasis response, in osteoblastic lesions, to anticancer therapy. At best, usually, osteoblastic lesions appear stable in the short term, generally lingering for months or years after an osseous metastasis has been essentially eradicated at that site [2]. Hence, in prostate cancer, for example, Prostate Cancer Clinical Trials Working Group guidelines consider a favorable therapeutic response of osseous disease as the absence of new bony lesions and the stable scintigraphic appearance of previously identified bony lesions [1].
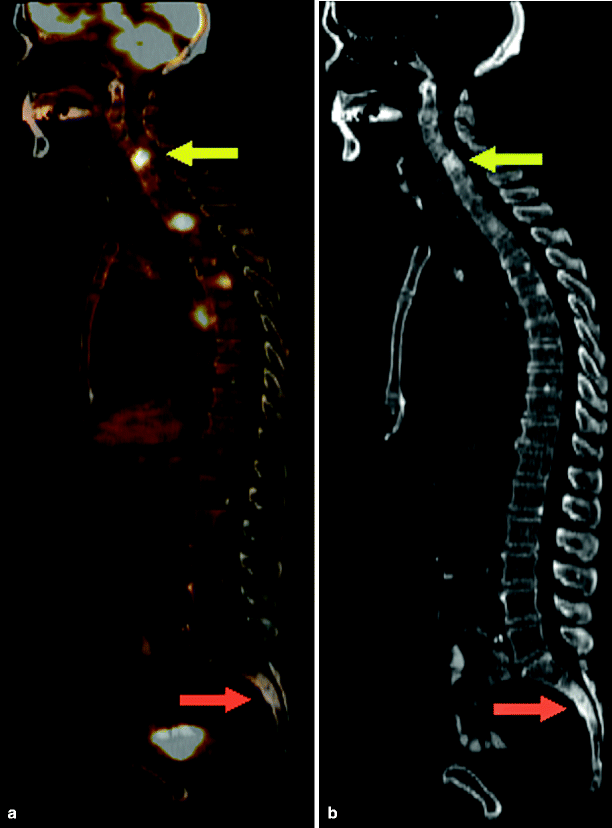
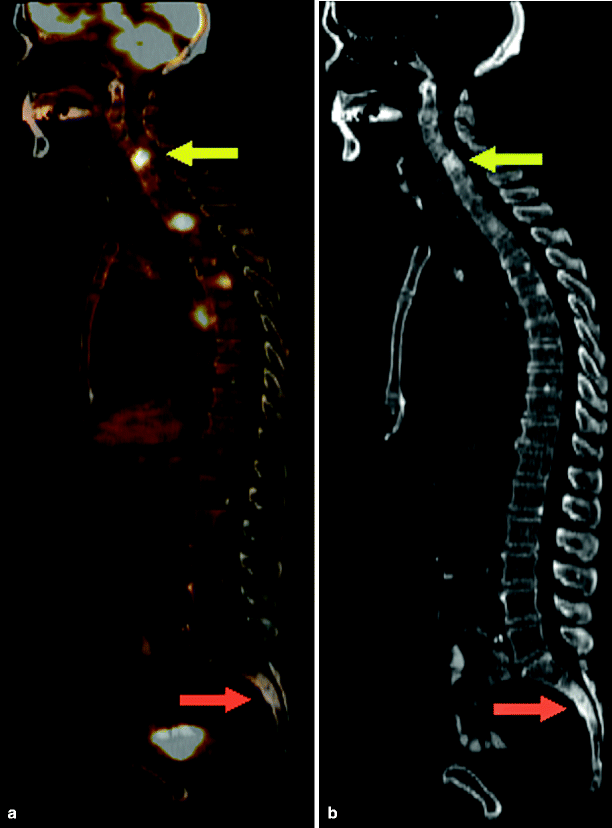
Fig. 9.5
Prostate cancer patient with bony metastases. (a), Fusion FDG-PET-CT, sagittal tomograph. (b), Companion CT, bone window setting. Note that multiple radiodense vertebral lesions are FDG avid (e.g., yellow arrow), consistent with viable osteoblastic-type metastases. However, the radiodense sacral lesion (red arrow), a site of biopsy-proven metastatic disease in the past, is not FDG avid, consistent with treated-disease (i.e., a lesion of metastatic etiology lacking viable tumor)
Lymphoscintigraphy in GU Oncology
The sentinel lymph node paradigm involves injecting a lymphatic tracer around the tumor region and then using imaging and intraoperative radioprobes to find the node or lymph nodes that drain the tumor region, indicated by nodal radioactivity due to uptake of the lymphatic tracer. Initial histological sampling is restricted to these sentinel nodes, followed by more extensive lymphadenectomy if intraoperative histology identifies sentinel nodal metastasis. If sentinel nodes reveal no metastatic involvement, this is taken to be sufficient for pathological staging (as pN0, in American Joint Committee on Cancer parlance), and the patient is spared extensive nodal dissections and the morbidity it too frequently entails [4–7]
Lymphoscintigraphy has long been [8, 9] and continues to be an active area of clinical research in prostate cancer [10] and penile cancer for guiding nodal sampling for pathological staging. No noninvasive imaging technique currently available offers a reliable substitute for histopathology in prostate and penile cancer nodal staging, fundamentally because micrometastatic disease is undetectable by currently available imaging methods [4]. The technique shows promising accuracy [4, 10, 11]. Exceptions [12, 13] point to a need for technical improvements and application of the sentinel node approach to appropriate patient populations. Presurgical SPECT-CT will likely improve lymphoscintigraphy imagery as an intraoperative reference aid to the surgeon [14]. Penile cancer research suggests lymphoscintigraphy is probably best-applied to patients with no palpable inguinal adenopathy (cN0), in which the technique is highly accurate [15, 16]. Cancer cells in enlarged metastatic lymph nodes might have thoroughly displaced the phagocytic cells responsible for nodal tracer uptake, resulting in false negatives.
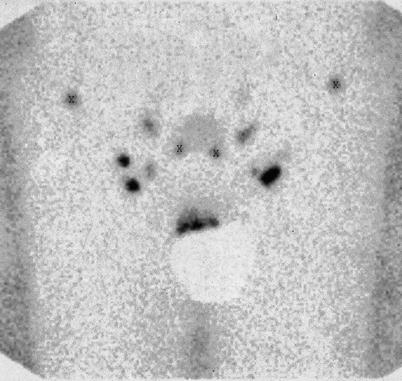
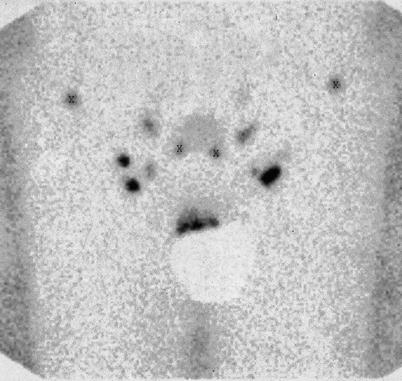
Fig. 9.6
Planar pelvic lymphoscintigraphy 3 h after injection of 99mTc tracer adjacent to a cancer of glans penis. Bilateral scintigraphic hotspots indicate draining sentinel lymph nodes draining the penile region. An external lead shield covers the penis and manifests as a “cold” region. The shield improves image clarity because residual radioactivity at the injection site can potentially obscure adjacent nodal uptake, scintigraphically. (Images courtesy of Jørgen Bjerggaard Jensen, MD, Department of Urology, Aarhus University Hospital, Skejby, Denmark)
FDG-PET Value in Prostate Cancer Staging
Older clinical trials predating PET-CT suggested FDG-PET had poor diagnostic sensitivity for prostate cancer [17, 18], possibly explaining why current prostate cancer guidelines do not include FDG-PET, even as an optional imaging study [1, 19]. However, in clinical practice, as of 2008, the US National Oncologic PET Registry (NOPR) found that prostate cancer patients were most frequently studied by FDG-PET and that FDG-PET results changed therapeutic management in a third of prostate cancer patients studied, with prognostic implications [20, 21]. Though metastatic prostate cancer can indeed manifest with mild or no FDG avidity, recent clinical data using state-of-the-art technology suggest that FDG-PET, particularly PET-CT [22], is an effective modality for disease (re)staging in high-yield prostate patient populations defined by threshold values of serum prostate-specific antigen (PSA) level or velocity [23, 24] and progressive, castration-resistant disease [17, 25–27]. In such patients, FDG-PET offers a means for evaluating bony tumor response in a timely fashion [26], potentially avoiding the pitfalls and limitations of bone scintigraphy [28]. CT and MRI also have difficulty distinguishing viable versus treated osseous metastases and even misleading increases in serum PSA levels (a tumor biomarker commonly used as a surrogate index of systemic prostatic tumor burden) can occur, preceding a favorable tumor response to treatment, not uncommonly—a so-called PSA flare response [29]. PET visualization of skeletal foci of FDG uptake, consistent with metastases, however, reliably indicates the presence of viable metastases at those sites [23, 28]. FDG-PET may be useful when conventional biochemical and imaging assays are discordant or suspected to be false-negative [25] (e.g., PSA-negative prostate cancer [30]).
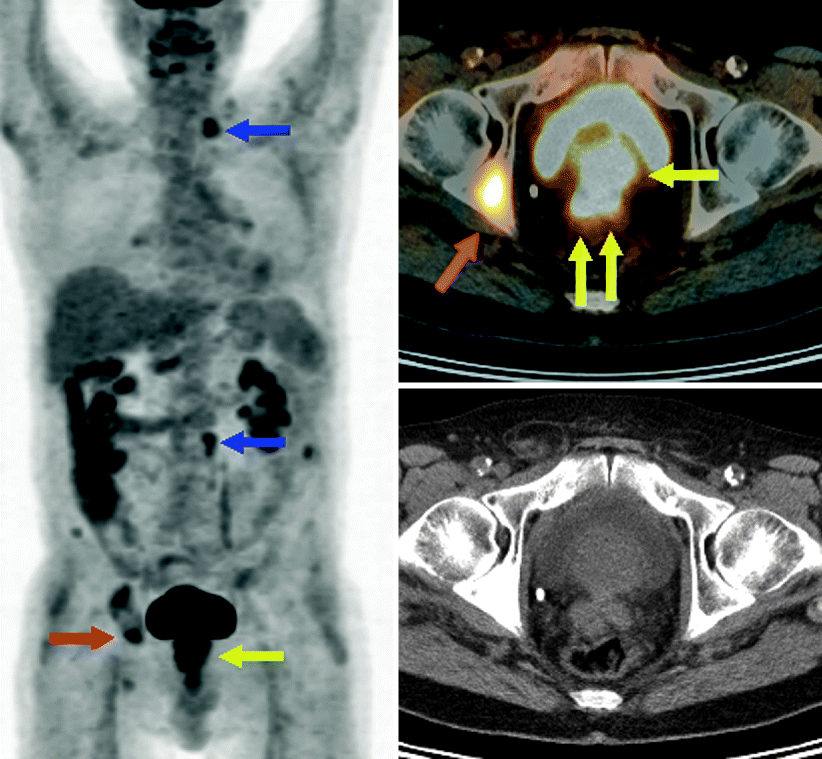
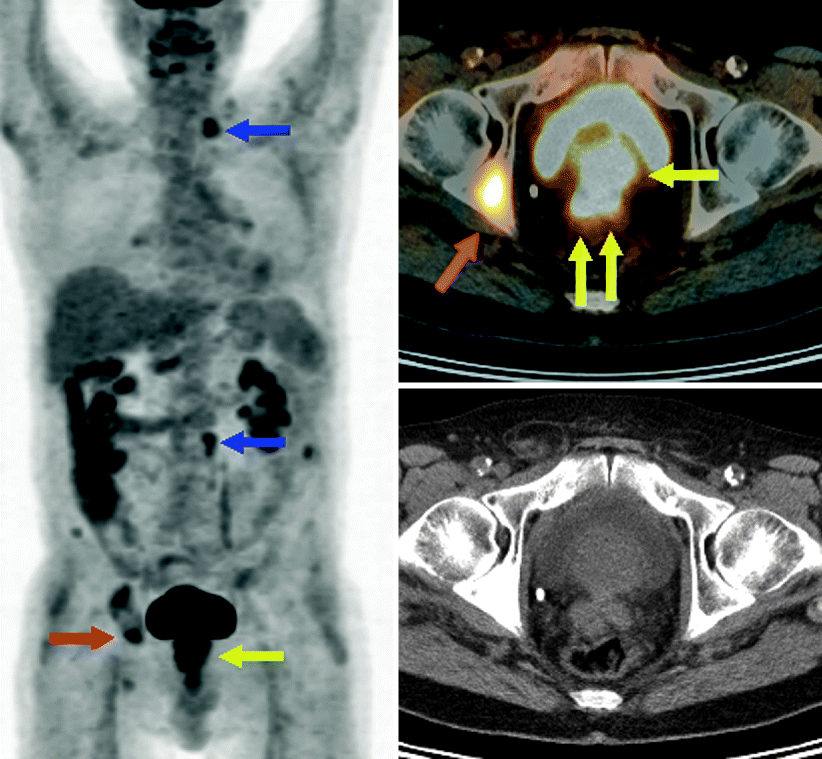
Fig. 9.7
PET-CT imagery shows FDG-avid primary prostate cancer invading seminal vesicles bilaterally (yellow arrows), metastatic adenopathy in the neck and abdomen (blue arrows), and multiple osseous metastases, including, for example, in the right acetabulum (red arrow)
FDG-PET and the Primary Prostate Lesion
For evaluation of a known or suspected primary prostate tumor, MRI is the modality of choice [31], providing excellent detection of extracapsular extension and involvement of the seminal vesicles or other neighboring tissues [23]. If an FDG-avid lesion of the prostate lesion is discovered incidentally in a patient without known prostate cancer (i.e., having a PET scan for another indication), the finding must receive further appropriate evaluation to exclude a neoplasm [30]. Most untreated primary prostate tumors are FDG avid [20]. The differential diagnosis also includes other FDG-avid diseases, such as benign prostatic hyperplasia and focal prostatitis [18, 32], which may require further clinical management. In the prostate cancer patient, after radical prostatectomy, FDG-PET appears unable to distinguish local recurrence from scarring [33].
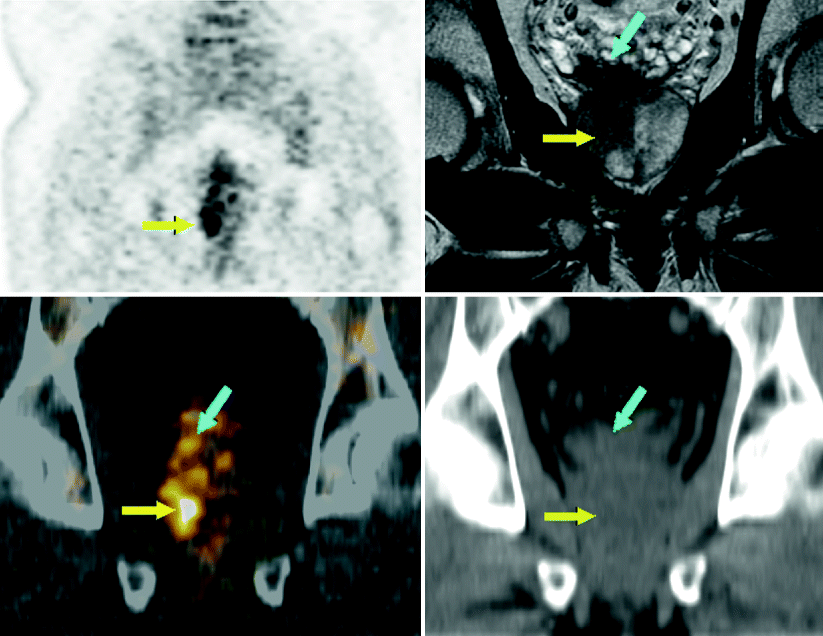
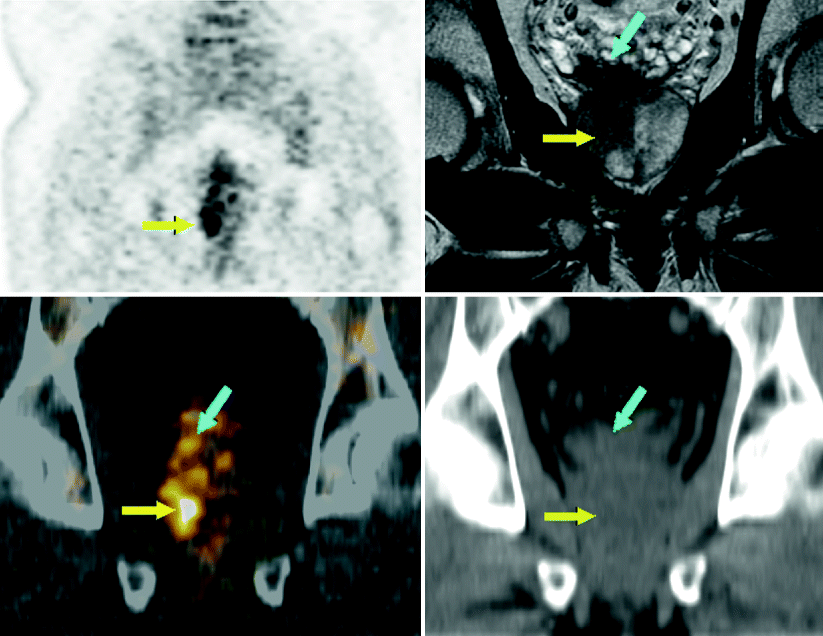
Fig. 9.8
FDG-PET-CT imagery of a 50-year-old man with a history of synovial sarcoma. PET visualized an incidental hypermetabolic lesion in the rightward region of the prostate gland (yellow arrow). Prostatic MRI evaluation confirmed a large prostatic tumor, also showing gross right seminal vesical invasion not evident on PET (blue arrow). Coronal PET image of pelvis (top left). Coronal T2W MRI prostate image (top right). Coronal CT image of prostate region (bottom right). Coronal PET-CT fusion image of prostate region (bottom left)
New PET Tracers Selective for Prostate Histology
A commonly noted fundamental limitation of FDG, as a tumor tracer, is that a wide variety of nonneoplastic diseases are also FDG avid. New tracers selective for prostate histology are in development as imaging agents for tumor detection. 16β-[18F]fluoro-5α -dihydrotestosterone (FDHT) is an analog of the main prostatic form of testosterone, radiolabeled for PET. FDHT-PET has already undergone initial testing, in prostate cancer patients, demonstrating the feasibility of its use, in tumor detection [34–36].
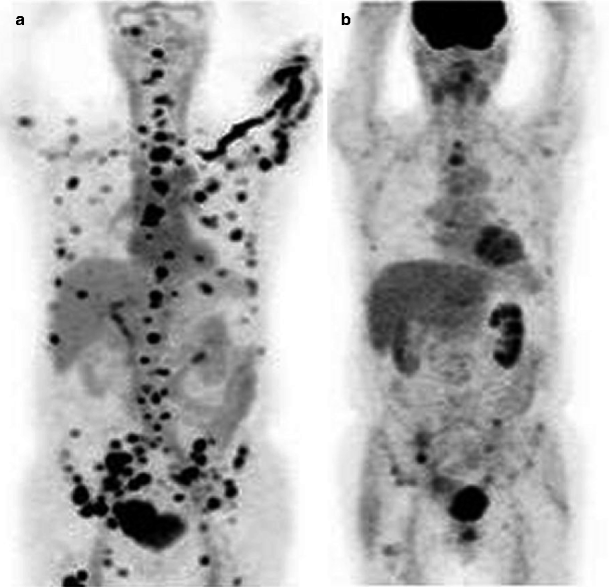
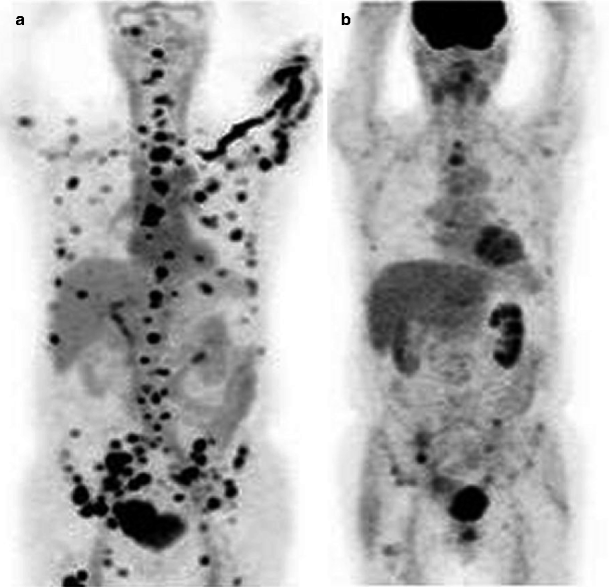
Fig. 9.9
A 69-year-old male with metastatic prostate cancer. FDHT-PET image (a) reveals far more scattered widespread metastases than evident on FDG-PET (b). The two PET studies were obtained 24 h apart (Images courtesy of Dr. Josef Fox, Memorial Sloan Kettering Cancer Center, NY)
Capromab Pendetide Scintigraphy and Prostate Cancer
Early clinical trial data showed the feasibility of detecting metastatic prostate cancer in patients, scintigraphically, by use of a radiolabeled antibody, capromab pendetide (ProstaScint; EUSA Pharma, Langhorne, PA), which targets glutamate carboxypeptidase II, also known as prostate-specific membrane antigen (PSMA) [37, 38]. National Comprehensive Cancer Network (NCCN) prostate cancer guidelines support capromab pendetide radioimmunoscintigraphy as an optional diagnostic tool in salvage setting evaluation. FDA approved in 1996 with promising early trials [39, 40], postmarketing clinical experience with capromab pendetide radioimmunoscintigraphy has been criticized by some as being of low diagnostic yield, both in the diagnostic clarity of the imagery it provides and in terms of its incremental diagnostic value beyond standard imaging assays (CT and MRI) [41, 42]. Recent technological advances in scintigraphic imaging hardware and software have spurred a reevaluation of the clinical diagnostic performance of radiolabeled capromab pendetide. Notably, SPECT-CT fusion imaging appears to increase the diagnostic sensitivity and specificity of capromab pendetide radioimmunoscintigraphy [43]. Increasing the diagnostic yield of capromab pendetide radioimmunoscintigraphy, in routine clinical practice, may also require better patient selection: identifying clinical variables that provide a higher pretest likelihood that capromab pendetide radioimmunoscintigraphy results will be positive (similar to the aforementioned discussion of FDG-PET in prostate cancer) [44]. Capromab pendetide radioimmunoscintigraphy is utilized predominantly for detection of metastatic disease, with no clear evidence that it can supplant MRI for evaluation of local tissue invasion at the primary site [45]. Recent research has explored a novel application of capromab pendetide radioimmunoscintigraphy in defining a biological target volume in planning conformal prostate brachytherapy [46].
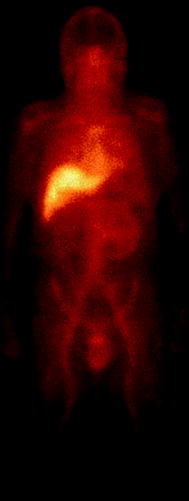
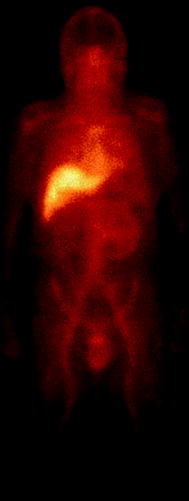
Fig. 9.10
An anterior planar scintigraphic image of the head, neck, and torso, showing the normal biodistribution of radiolabeled capromab pendetide (ProstaScint). (Image courtesy of Gary Ulaner, MD, Memorial Sloan-Kettering Cancer Center, New York, NY)
FDG-PET Value in Bladder Cancer Staging
For detection and evaluation of primary tumors and local recurrence, nonradiological approaches remain the standard [47, 48]; CT urography offers no clear advantages over cystoscopy, histopathology, and urinalysis [49, 50]. Hence, recommendations regarding the use of FDG-PET in urinary bladder cancer should focus on its potential role in detecting synchronous upper urinary tract neoplasms, which are of significant incidence, in urinary bladder cancer patient populations [49] and detecting nodal or distant metastatic disease [48–50]. Pretreatment imaging guides tissue biopsy and helps determine what course of treatment is appropriate in bladder cancer [47].
Neither NCCN [47], European Association of Urology [51], nor American College of Radiology guidelines [49, 50] currently recommend a role for FDG-PET in the management of bladder cancer. Yet FDG-PET imaging results change bladder cancer management in 38 % of patients [20]. FDG-PET/CT is sensitive and specific for detecting metastatic bladder cancer. When added to standard imaging, PET/CT information changed clinical management in 68 % of cases [52]. State-of-the-art fusion PET/CT and iterative PET image reconstruction algorithms improve diagnostic accuracy [22], as these were unavailable for FDG-PET in the 1990s.
As is typical for FDG-PET (and all current imaging modalities), patients with microscopic lymph node metastases were the most common false-negatives [53, 54]. Yet FDG-PET/CT can identify occult nodal metastases in patients with prior negative conventional abdominopelvic CT [53].
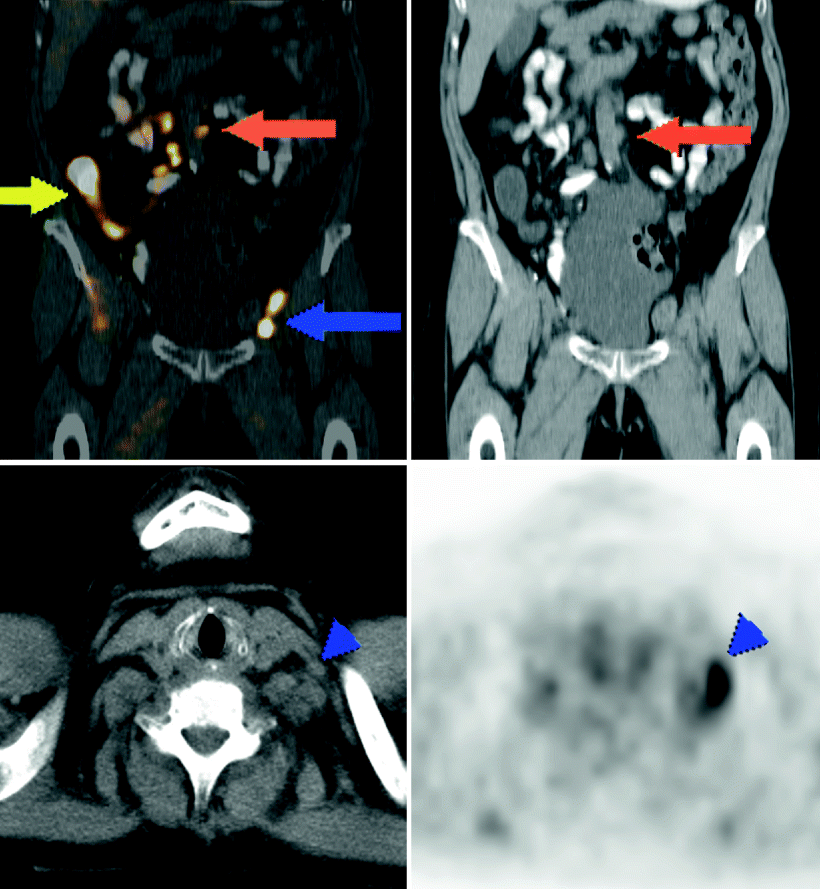
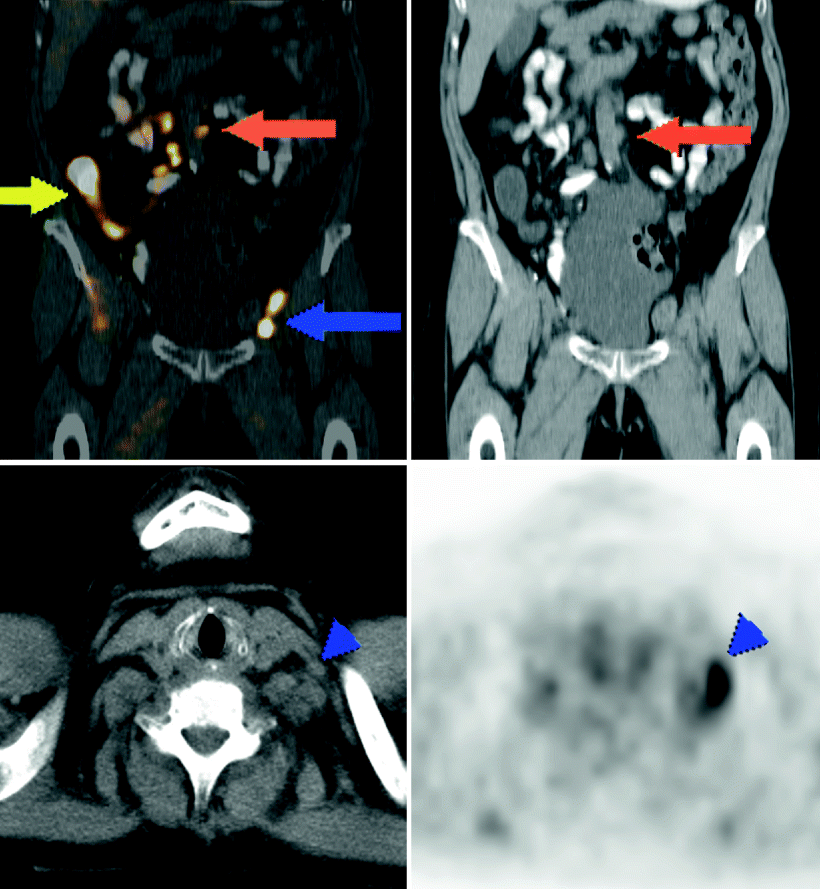
Fig. 9.11
A 58-year-old female with bladder cancer, status postcystectomy with ileal conduit (yellow arrow) filled with excreted FDG. Hypermetabolic adenopathy in the left external iliac region (blue arrows) was also evident on CT by its enlarged size. Markedly hypermetabolic para-aortic adenopathy (red arrow), suspicious for metastatic disease, was subcentimeter in transaxial short axis, reported as equivocal on same-day dedicated CT. Not identified by CT was adenopathy in the left supraclavicular region (blue arrowhead), hypermetabolic on PET, suspicious for a Virchow nodal metastasis
FDG Excretion Is a Problem in Urinary Tract/Renal PET Imaging
FDG is excreted via the urinary tract and the radioactive signal from excreted FDG can obscure PET evaluation of the urinary tracts, as well as renal parenchyma and adjacent nodal groups [55–57]. Yet multiple studies have shown that FDG-PET has excellent diagnostic accuracy for detection of urothelial neoplasms in the bladder and upper tracts when imaging is performed after clearance of excreted FDG, with urinary clearance facilitated by delayed imaging [56, 57] (e.g., imaging at 2 h after tracer injection instead of the more conventional 1-h time-point); oral or intravenous hydration [53, 56, 57]; diuretics [53, 56, 57]; urinary bladder drainage and irrigation [55, 58]; and/or combined use of excreted radiographic contrast [59]. However, because such practices to facilitate urinary clearance for FDG-PET are probably not in widespread use, referring clinicians may wish to approach their local imaging specialists to discuss the matter.
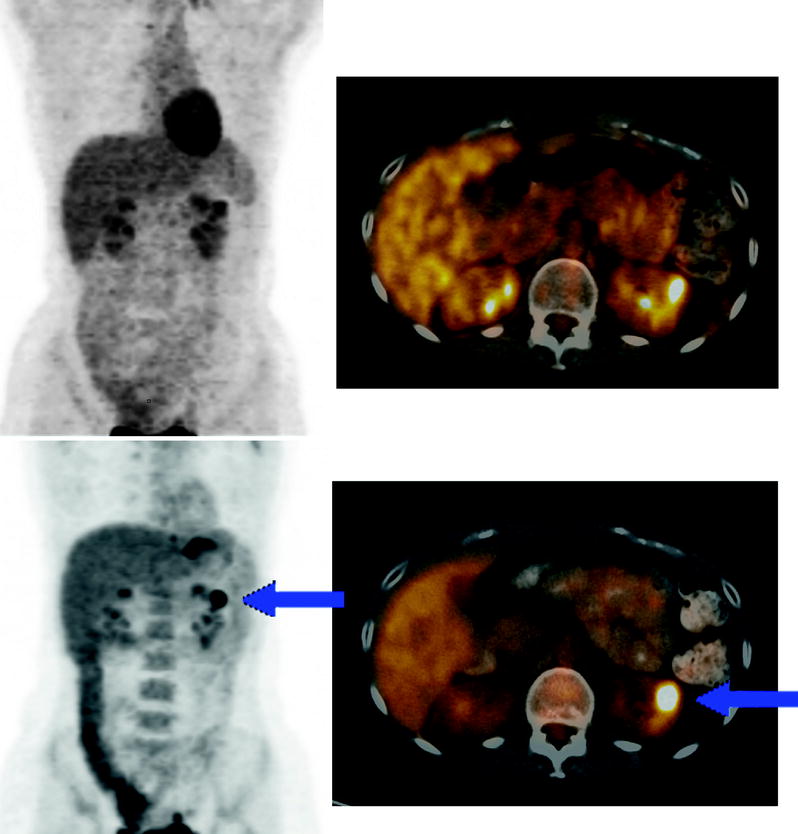
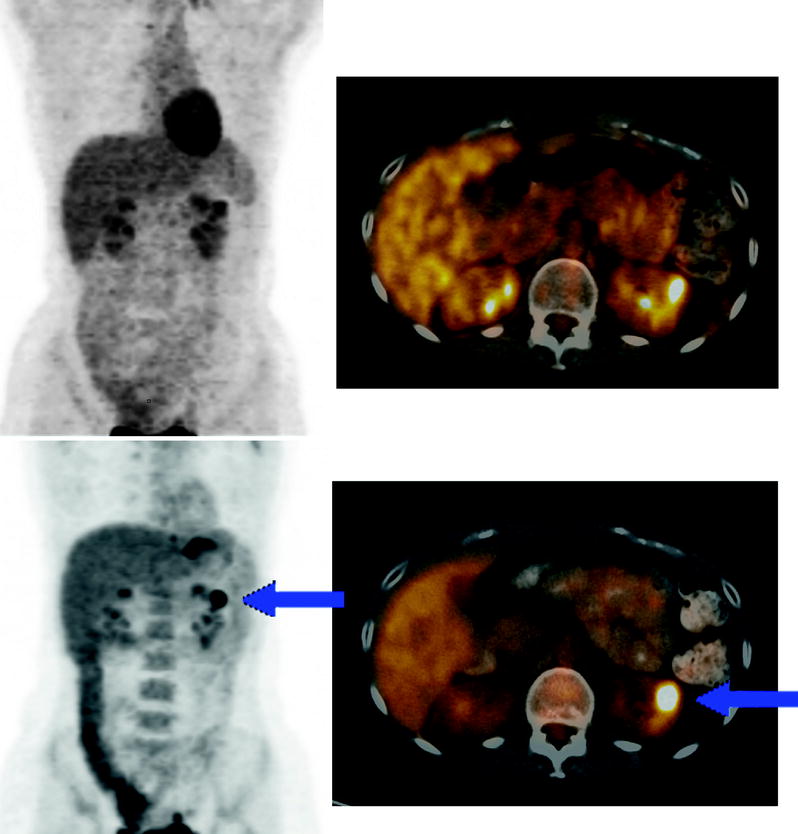
Fig. 9.12
PET scan was performed standard 60 min after FDG injection and was negative. Follow-up PET scan obtained 120 min after FDG injection. A hypermetabolic upper-pole parenchymal lesion of left kidney (blue arrow) is more conspicuous because the 2-h imaging delay allowed for clearance of excreted FDG from the renal calyceal system. Biopsy revealed renal oncocytoma
Renal PET Imaging: FDG-PET and Beyond
For evaluation of the patient with a suspicious renal mass prior to histological evaluation, data from multiple small studies suggest FDG-PET sensitivity is 85–90 % for malignancy [60–64]. Note that these reports were older studies using PET, not PET-CT, and usually did not employ diuretic administration or other procedural techniques that subsequently have been shown to clear excreted FDG that can obscure evaluation of the upper urinary tracts and renal parenchyma. The sensitivity of FDG-PET is probably lower for lesions that are predominantly cystic, whereas solid malignant renal masses have a high density of cells to produce a detectable signal on PET, malignant cells lining the periphery of a cystic renal lesion will produce a less-concentrated uptake signal less easily detected on PET imagery.
Focal accumulations of excreted tracer in the renal pelvicaliceal system can mimic hypermetabolic renal parenchymal tumors. Renal oncocytomas in multiple cases have demonstrated high FDG avidity on PET imagery, presenting another false-positive (for malignancy). (See Fig. 9.12)
A PET tracer highly selective for clear cell carcinoma has been studied, in clinical trials: the iodine124-labelled antibody chimeric G250 (124I-cG250) [65]. Interestingly, in a pilot study of 25 renal cancer patients, this PET radioantibody did not accumulate in nine non–clear cell carcinomas (including two papillary cell carcinomas), but accumulated in 15 of 16 clear cell carcinomas. A larger clinical study, using iodine131-labeled cG250 in renal cancer patients, supports the concept that the radioantibody is histology specific [66].
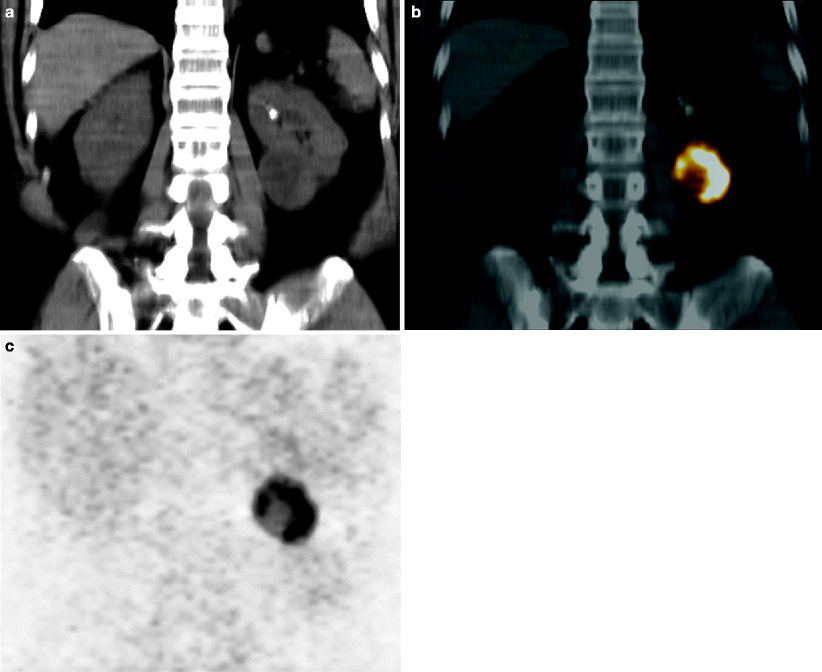
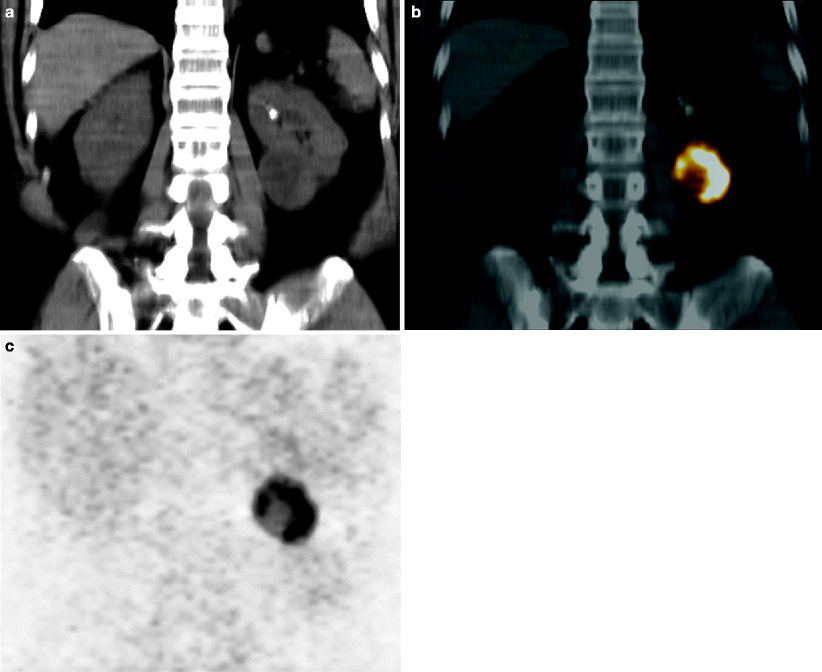
Fig. 9.13
124I-cG250 PET-CT of a 63-year-old man with a clear cell carcinoma of left kidney, shown on a coronal abdominal tomograph by CT (a), fusion (b), and PET (c). Note the utter lack of background PET activity and the intense tumor uptake, illustrating the potential of diagnostic PET imaging with radiolabeled antibodies
The Value of FDG-PET in Testicular Cancer
FDG-PET has been found sensitive both for seminoma [67] and nonseminomatous germ cell tumors (NSGCT) in general [68], with some researchers suggesting histology-specific variations in tumor FDG avidity [69–72]. FDG is the preeminent radiopharmaceutical for imaging of seminoma in current practice [73, 74]. For stage IIC-III seminoma patients with normal serum markers, current NCCN guidelines recommend FDG-PET as the preferred test for evaluating a residual mass when present following primary chemotherapy and for identifying whether viable cancer is present within the mass. The use of FDG-PET is also recommended, when no residual mass is present, during follow-up “as clinically indicated” [73]. Additionally, the NCCN suggests FDG-PET can be considered for stage IIB-III seminoma patients with elevated but stable β-human chorionic gonadotropin levels. FDG-PET is not suggested for the evaluation of nonseminomatous germ cell tumors (NSGCT) patients nor for initial staging of seminoma patients, per guidelines of the NCCN and the European Germ Cell Cancer Consensus group (EGCCC) [73, 74].
Guidelines suggest that FDG-PET can be used “as clinically indicated” for follow-up of stage IIC-III seminoma patients [73]. Yet FDG-PET is not considered of proven clinical benefit in the follow-up of NSGCT patients [73, 74]. For example, in one study, a negative FDG-PET/CT did not have a statistical impact upon relapse risk in stage I NSGCT patients at high-risk for recurrence [75], even though FDG-PET/CT identifies metastatic disease in up to 21 % of stage I NSGCT patients with serum tumor markers and CT showing no evidence of disease [75, 76].
Guidelines suggest that FDG-PET cannot be recommended for initial staging of GCT patients [73, 74]. The EGCCC suggested that available FDG-PET research in GCT staging was not conclusive as of 2008. None of the research articles cited offered the same conclusion. In fact, the EGCCC statements cite multiple articles which reported that FDG-PET was more sensitive for tumor detection than CT [70, 75, 76], offering improved staging of GCT patients compared to CT alone [70, 76, 77]. These guidelines did not clearly distinguish between FDG-PET and FDG-PET/CT technology and sometimes cited certain older research that used outmoded PET methodology [78, 79].
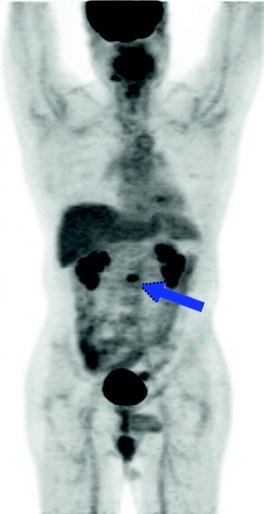
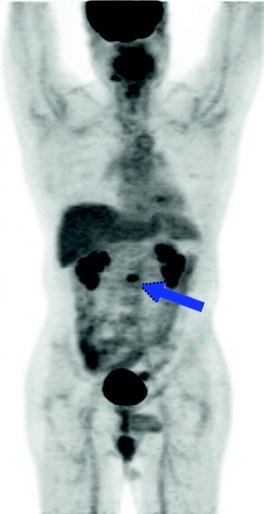
Fig. 9.14
A 44-year-old male with seminoma postorchiectomy referred for FDG-PET to rule out relapse during surveillance. Hypermetabolic aortocaval lymph node is visualized (blue arrow), consistent with metastatic disease
Adenopathy Detected by FDG-PET
In the seminoma patient with a residual mass, postchemotherapy, FDG-PET is efficacious for noninvasively identifying the presence of viable tumor cells [67, 68, 71, 80, 81]. FDG-PET should be performed 6 weeks after the end of chemotherapy, as false-positive PET findings are more frequent in the early postchemotherapy period—probably due to a greater presence of FDG-avid inflammatory/phagocytic cells within the mass during that time [82–84
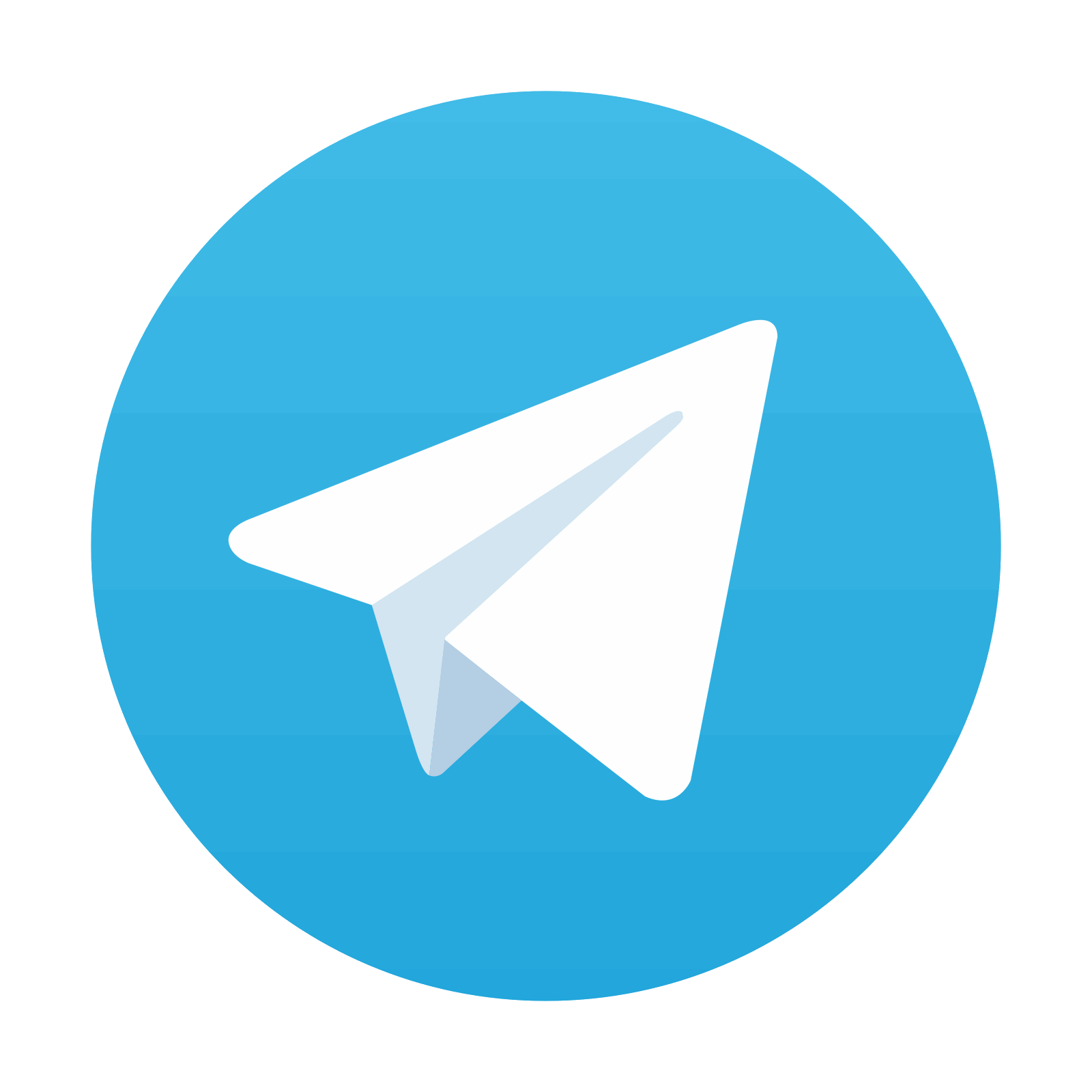
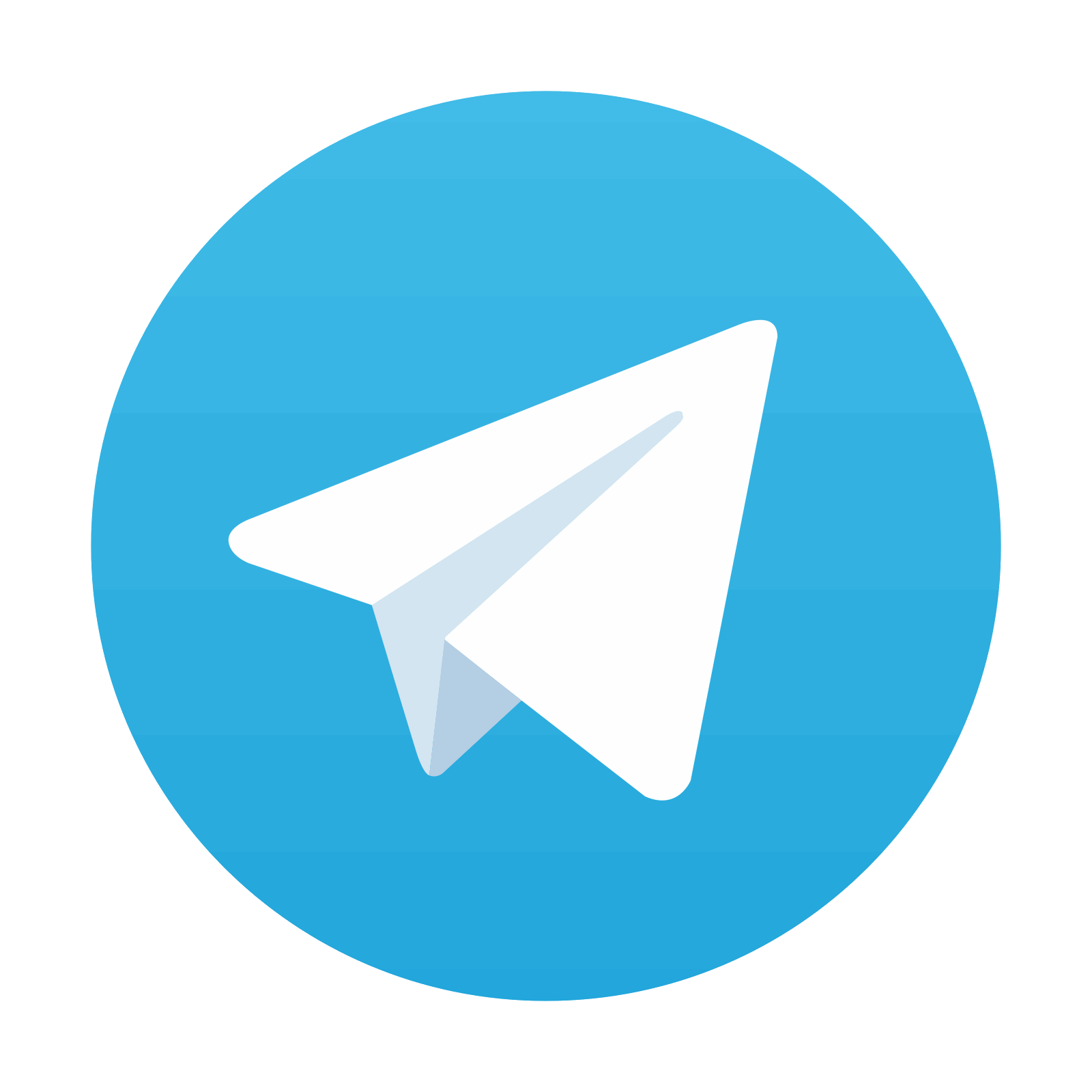
Stay updated, free articles. Join our Telegram channel

Full access? Get Clinical Tree
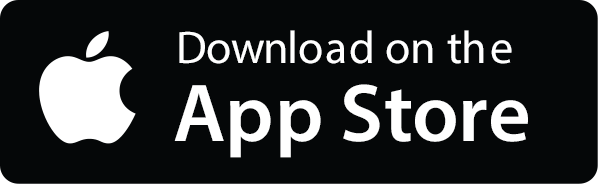
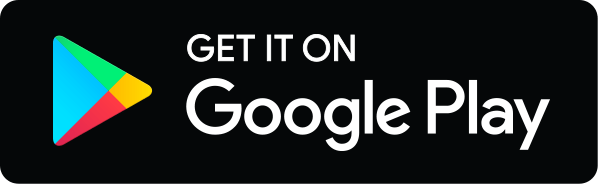