Fig. 1
Left side shows typical denervation pattern in NPC neuropathy including the extensors and peroneal muscles visible due to the prolonged T2 time of the muscle tissue (arrows on left image). In cases of L5 denervation as shown on the right, the posterior tibial muscle, and the popliteal muscle (arrows on right image) are additionally affected
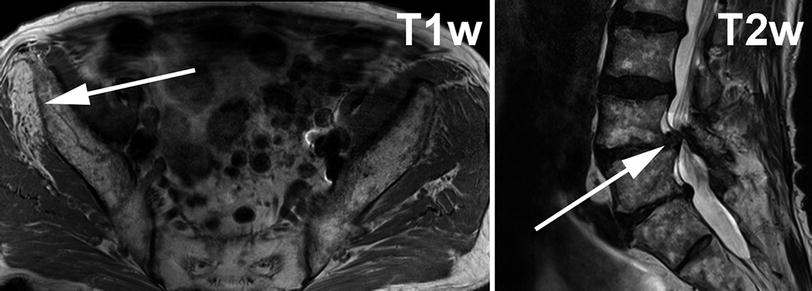
Fig. 2
Left image shows T1-weighted axial slice through gluteal region. On the right side of the patient (solid arrow on left) the gluteus minimus muscle shows strong T1w hyperintense signal alteration indicating fatty atrophy in the chronic stage of denervation. The gluteus minimus muscle receives its supply predominantly from L4 and L5. In this case right sided severe L5 radiculopathy was caused by disk sequestration at L4/L5 level on top of preexisting severe degenerative stenosis of the spinal canal (right image, solid arrow)
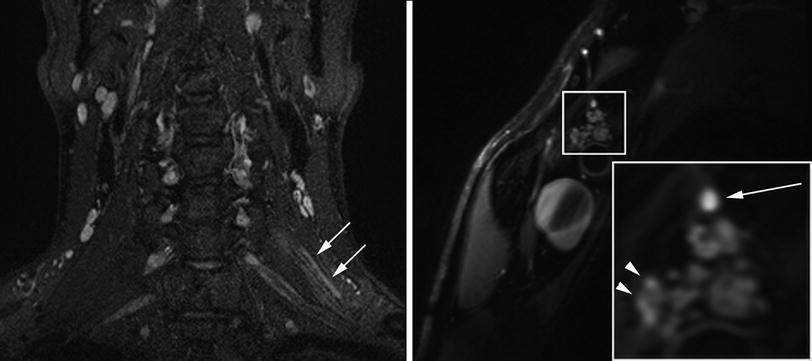
Fig. 3
Patient with inflammatory left radiculopathy of C5. Coronal SPACE (Sampling Perfection with Application optimized Contrasts using different flip angle Evolution) with fat-saturation by inversion-recovery reveals markedly hyperintense C5 spinal nerve (arrows). The sagittal-oblique T2-weighted fat-saturated image with high resolution through the plexus at a more distal level displays hyperintense nerve lesions in the posterior cord (arrow, right image) and the lateral cord (arrowheads, right image) in those fascicles emerging from C5 at more proximal level
4.2 Plexopathies
4.2.1 Anatomy of the Brachial Plexus
In order to understand and diagnose lesions to the brachial plexus adequately, knowledge of the anatomy of this complex region is necessary. The brachial plexus is formed by the ventral nerve roots of five spinal cord segments. The roots C5, C6, C7, C8, and Th1 always contribute to the formation of the upper extremity nerves. Furthermore, participation of the ventral nerve root C4 or the involvement of the nerve root Th2 can be observed. Frequencies of anatomical variations are different on the right or left side as well as in males and females (Uysal et al. 2003). Although diverse terminologies have been used, a contribution by C4 and absent or minimal contribution by Th2 has been most frequently defined as a “prefixed” condition, whereas a major contribution by Th2 and absent contribution by C4 has been described as “postfixed” brachial plexus [reviewed in (Pellerin et al. 2010)]. The “prefixed” condition can be encountered quite frequently [in up to 63 % (Kerr 1918)]. The postfixed condition is generally rather rare and has been observed in larger series in only up to 8 % (Kerr 1918; Loukas et al. 2007). A certain degree of Th2 contribution always occurs, either in the extrathoracic form (through the intercostobrachial nerve) or the intrathoracic form (through a communicating branch between Th2 and Th1 (Loukas et al. 2007). Clinically, Th2 participates in the sensory processing, mostly in the innervation sites of the medial and posterior antebrachial cutaneous nerves and thus is more of surgical than imaging interest. However, the detection and description of a postfixed brachial plexus are of clinical relevance, since it has been postulated to be more commonly associated with irregularities of the C7 transverse process and with cervical ribs.
In normal and pathological conditions MRI can be used to depict the integrity of the intradural portions of the ventral and posterior nerve rootlets using MR myelography. Since fibrous band like attachments between the epineurium of the spinal nerves C5, C6, and C7 and the cervical transverse processes protect these nerves from traction, they are less likely to be avulsed than C8 and Th1, which do not show these protective ligaments (Sunderland 1974).
The ventral nerve roots can be easily identified on short-tau inversion recovery (STIR) or neurography T2-weighted sequences, as their slightly hyperintense signal gives contrast against to the dorsally located scalenus medius muscle. The T2-weighted pulse sequences used in MR Neurography are typically fat-saturated and provide submillimeter in-plane resolution. Nerve lesions of various origins seem to be detectable in robust manner over a range of echo- and relaxation times including T2-weighted and proton-density weighted contrast. As the characteristic topographic anatomy with the brachial plexus nerve roots travelling dorsally to the anterior scalenus muscle is encountered in only 32 % of cases bilaterally, anatomic variations are described to occur frequently in this region, and may be addressed, when reporting a plexus imaging study (Harry et al. 1997). In 15 % the C5 and C6 nerve roots are found to pierce through the scalenus anterior muscle. In 3 % a small slip of the scalenus medius muscle travels anterior to one or two of the brachial plexus roots (Harry et al. 1997).
The differentiation of different trunks and later forming divisions of the supraclavicular part of the brachial plexus by imaging is extremely challenging. The upper trunk is formed by the nerve roots C5 and C6 at the lateral border of the scalenus medius muscle, the middle trunk of ventral nerve root C7, and the lower trunk of the roots C8 and Th1 posterior to the anterior scalenus muscle (Johnson et al. 2010). Each trunk divides into a ventral and dorsal division. The posterior divisions will form the posterior cord, which is the smallest of the three cords. The anterior divisions of the superior and middle trunk unite on the lateral side of the axillary artery to form the lateral cord. The anterior division of the middle trunk gives rise to the medial cord. Due to the limited dimensions of the infraclavicular space, clear imaging differentiation of the divisions may not be possible. However, the cords can be easily identified by MRI due to their topographic relationship with the axillary artery. The posterior cord branches into the axillary and radial nerves. The lateral cord gives rise to the musculocutaneous nerve and lateral root of the median nerve and the medial cord evolves into the ulnar, cutaneous antebrachii, and brachii medialis nerves as well as the medial root of the median nerve.
4.2.2 Traumatic Plexopathies
Traumatic injuries of the brachial plexus are mainly caused by motor vehicle accidents, with the majority of them being motor cycle related. Less frequent causes for traumatic plexopathies are falls, iatrogenic injuries, sports injuries, penetrating, and gunshot wounds. More than two-thirds of patients are young male adults aged between 20 and 40 years. The injuries affect mostly the root and the trunk level of the brachial plexus. Isolated infraclavicular plexus injuries are a rarity (Barman et al. 2012).
Surgical posttraumatic plexus repair including nerve transfer aims to restore elbow flexion and shoulder abduction function (Yang et al. 2012). Preoperative imaging plays an important role in planning these procedures. Internationally, over 90 % of dedicated peripheral nerve surgeons consider (CT or MR) imaging as important diagnostic step in the workup of patients with traumatic brachial plexus injuries (Belzberg et al. 2004).
The imaging assessment of patients with traumatic plexopathies has to respect the diversity of injury mechanisms involved in traumatic plexus injuries. Traction injuries (axonotmesis and neurapraxia) to either the roots C5 and/or C6 as well as to the upper trunk are most frequently seen. Lower trunk injuries occasionally occur; however, middle trunk traction injuries are almost never encountered (Barman et al. 2012). The second type of injury mechanism is nerve rootlet avulsion—a “preganglionic” separation of the ventral (motor) and/or less frequently dorsal (sensory) rootlets from the spinal cord. This most severe form of injury mechanisms mainly involves the lower plexus nerve rootlets (C7, C8, and Th1) (Sunderland 1974) and is associated with no regenerative potential, as surgical repair of these rootlets is not successful. As third injury mechanism, rupture and complete discontinuity (neurotmesis) of extradural nerve roots or trunks can be sometimes observed (Fig. 4).
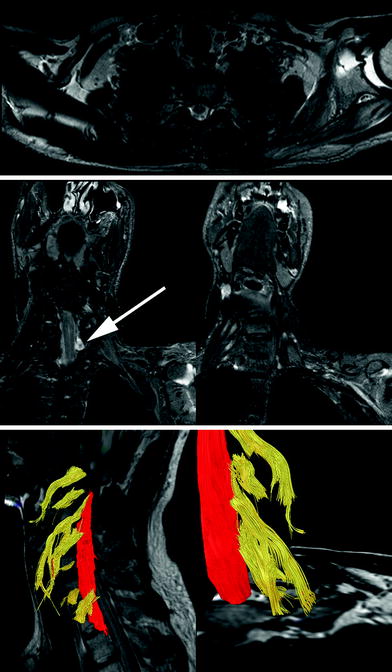
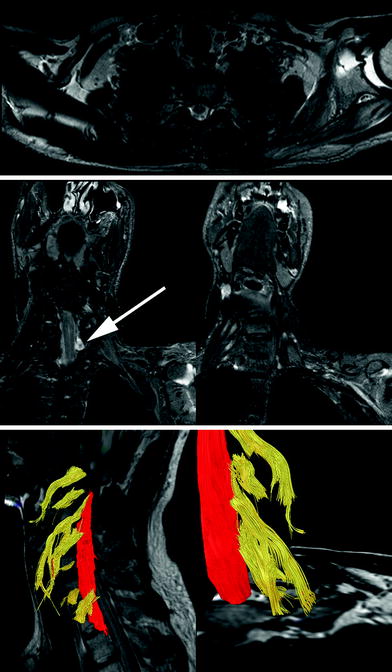
Fig. 4
Axial T2-weighted (upper row), paracoronal STIR (short tau inversion recovery) (middle row), and tractography images in a patient with a complete posttraumatic (fall from great height) brachial plexus paresis: In addition to the posttraumatic effusion of the glenohumeral joint, signal alterations of left sided subscapular and infraspinatus muscle are detected. The pattern of these signal alterations follows a characteristic distribution, typical for muscle denervation after brachial plexus injuries. The paracoronal STIR sequences show denervation edema of the middle and posterior scalenus muscles as well as the retracted distal nerve segments of the ruptured brachial plexus nerves. This finding is visualized in 3D by tractography (lower row). Moreover, diffusion tensor imaging (DTI) could not visualize the distal segments of the avulsed nerve root C7 and C8 (arrow points to pseudomeningocele), due to loss of anisotropy and Wallerian degeneration
Besides excluding or confirming posttraumatic injuries to the bones and joints of the shoulder girdle, the spinal cord, vascular, and soft tissue structures, the main objective in imaging of traumatic brachial plexus injuries is the imaging differentiation of the described injury mechanisms. The identification of an avulsion (preganglionic) injury is of immense clinical importance. As electrophysiological examinations are frequently equivocal in differentiating pre- and postganglionic injuries (Barman et al. 2012), imaging offers important presurgical information (Martinoli et al. 2010).
Pseudomeningoceles are tears of the dural recessus within the neuroforamen and can be easily identified by their bright signal on T2-weighted sequences (Fig. 4). The presence of a pseudomeningocele is a quite sensitive indicator of nerve root avulsion (preganglionic injury). However, 20 % of nerve root avulsion injuries are not associated with pseudomeningoceles (Yoshikawa et al. 2006; Martinoli et al. 2010). Thus, direct visualization of the rootlets is necessary to confidently rule out or confirm a preganglionic nerve root injury. For a long time the invasive technique of CT myelography, was the only method to accomplish this task. Recent studies indicate equal sensitivity and specificity of MR myelography techniques, in particular 3D heavily T2-weighted sequences (3D CISS, TrueFISP, FIESTA, and the DRIVE) and CT myelography in detecting nerve root avulsions (Doi et al. 2002). Furthermore, the inability to identify intact anisotropic nerve structures distal to the avulsed nerve root by diffusion tensor imaging (DTI) and tractography may help to even further improve the capabilities of MRI in the differentiation of pre- and postganglionic injuries (Fig. 4).
Traction injuries manifest themselves as neuromas in continuity, with thickening of the affected nerve. In future, new techniques in peripheral nerve MR imaging, such as DTI and tractography may be able to assess the severity of the traction injury and moreover the presence or absence of intact fascicles within the lesioned nerve segments.
Complete rupture of a brachial plexus nerve is characterized by an abrupt ending of the disrupted nerve and retraction of the distal nerve segments. Using tractography no continuity with the distal nerve segments can be detected (Fig. 4).
Finally, the assessment of the brachial plexus is complete, if the pattern of nerve lesions is in congruency with the pattern of acute or chronic denervation signs of the depicted muscles.
Typically, the field of view of a brachial plexus examination (if a flexible body-array coil is used), includes the muscles listed in Table 1. Denervation of paraspinal (dorsal branch of cervical nerves) and scalenus (ventral branch of cervical nerves/root) muscles occurs in cases of very proximal spinal nerve lesions (Fig. 4). In nerve avulsion injuries, characteristically the multifidus (= deepest of paraspinal muscles) and the levator scapulae muscle show denervation changes. Thus, denervation edema or volume loss of these specific muscle groups are helpful indicators and reassuring signs for avulsion injuries (Uetani et al. 1997; Hayashi et al. 2002). Knowledge about the specific innervations of depicted muscles is essential in differentiating between muscle signal changes due to denervation and those caused by posttraumatic changes.
Table 1
Plexus and segmental innervation of shoulder girdle and proximal upper extremity muscles, which is usually visualized by MRI of the brachial plexus
Muscle | Nerve | Cord | Trunk | Root |
---|---|---|---|---|
Deltoid | Axillary | Posterior | Upper | C5, C6 |
Subscapular | Subscapular | Posterior | Upper, middle | C5, C6, C7 |
Supraspinatus | Suprascapular | – | Upper | (C4), C5, C6 |
Infraspinatus | Suprascapular | – | Upper | (C4), C5, C6 |
Teres minor | Axillary | Posterior | Upper | C5, C6 |
Teres major | Subscapular, thoracodorsal | Posterior | Upper, middle | C5, C6, C7 |
Pectoralis major | Medial pectoral | Medial | Upper, middle, lower | C6, C7, C8, T1 |
Pectoralis minor | Lateral pectoral | Lateral | Upper, middle | C5, C6, C7 |
Subclavius | Subclavian | – | Upper | C5, C6 |
Serratus | Long thoracic | – | Upper, middle, lower | C5, C6, C7, C8 |
Scalenus anterior, medius, posterior | Ventral branches, cervical nerves | – | – | C4, C5, C6 |
Paraspinal muscles: multifidus, semispinalis cervicis and capitis, splenius capitis and cervicis | Dorsal branches, cervical nerves | – | – | – |
Trapezius, sternocleidomastoideus | Accessory nerve | – | – | – |
4.2.3 Perinatal Brachial Plexus Lesions
Although obstetric management of shoulder dystocia has significantly improved and the overall rate of cesarean sections has increased, the incidence of brachial plexus birth palsies lies still between 0.4 and 4.6 per 1,000 live births (Hoeksma et al. 2000; Foad et al. 2008). Thus, birth related brachial plexus injuries remain an epidemiologically significant burden. Generally, most perinatal plexus lesions are associated with transient functional deficits. However, a minority of patients will have to live with a sometimes remarkable degree of disability. The early clinical assessment of these cases is essential to provide timely surgical treatment in order to prevent major neurological deficits of the affected upper extremity. Since most treatment concepts favor early surgery in cases without clinical improvement up to the age of 6 months (O’Brien et al. 2006; Hale et al. 2010), the clinical examination of the injured neonates and infants remains the basis for further decision making. At this early time point, the role of electrodiagnostic testing and imaging is currently unclear. However, higher field strength and new developments in peripheral nerve MR imaging may add valuable morphologic information. Thus, future radiologists will be more frequently involved in the management of birth related plexus injuries.
The imaging approach in these patients should aim to solve the following tasks: (1) Detection of injuries to the spinal cord and of a preganglionic (=avulsion) injury of C5–Th2, (2) Visualization of the extent of nerve traction injuries and neuromas at the level of nerve roots, trunks, divisions, or cords, (3) Visualization of the degree and specific pattern of muscle denervation and consecutively, (4) The identification of early reactive bone/cartilage remodeling of the shoulder joint leading to glenohumeral deformities.
The assessment of nerve root avulsions remains challenging in the neonatal period. Although the presence of a pseudomeningocele (on MRI, CT, or myelography) shows a high sensitivity for nerve root avulsions, it is a rather nonspecific sign (Vanderhave et al. 2012). In some centers CT myelography continues to be the gold standard for the assessment of preganglionic injuries (Steens et al. 2011). However, in future the wide distribution of 3 T MR units and the use of noninvasive MR myelography sequences should allow a more specific evaluation of the preganglionic segments in these cases (Medina et al. 2006).
High-resolution STIR sequences in the orientation of the nerve roots and trunks are sensitively able to depict the involvement and extent of nerve lesions due to traction injuries and neuroma in continuity (Smith et al. 2008).
Moreover, atrophy patterns of the shoulder girdle muscles have to be evaluated. The subscapular and deltoid muscles are most frequently (in up to 87 and 89 % of patients) involved (Hogendoorn et al. 2010). Since the subscapular nerve arises from C5 to C7, subscapular muscle denervation in particular is characteristic for upper brachial plexus injuries. In addition the infraspinatus (in 71 %) and supraspinatus (in 74 %) muscles show signs of chronic denervation (Fig. 5) (Hogendoorn et al. 2010; Poyhia et al. 2005).
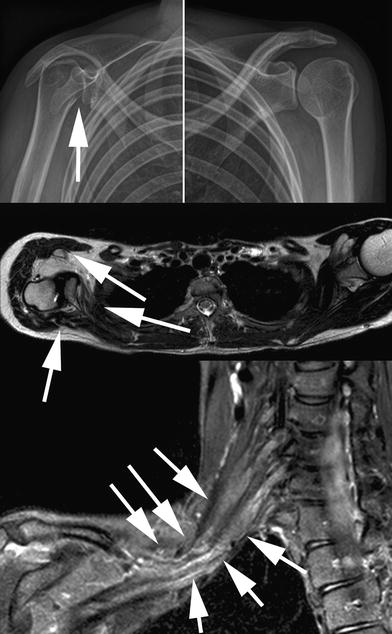
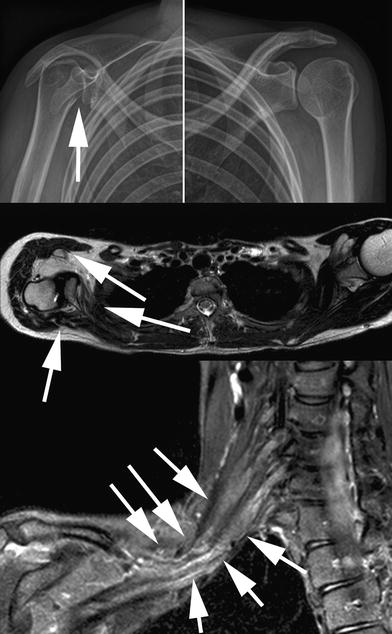
Fig. 5
Radiographs (upper row), axial T2-weighted, and paracoronal STIR (short tau inversion recovery) sequences in a 23-year-old patient with perinatal brachial plexus injury. There is subluxation of the humeral head (solid arrow in upper image) and remodeling of the right shoulder, typical for a glenohumeral deformity. Please note the significant atrophy of the subscapular and infraspinatus as well as right sided deltoid muscles (solid arrows in middle image). The paracoronal STIR image depicts extensive traction neuromas of the upper and middle trunk (solid arrows in lower image)
An imbalance in muscle strength may ultimately lead to an internal rotation contracture and progressive glenohumeral joint incongruence (Poyhia et al. 2005). In some cases, the glenohumeral deformity is early encountered and causes a significant osseous limitation in the range of motion of the shoulder joint. Thus, the radiological identification of this process is crucial for further physical therapy. Due to the optimal soft tissue contrast, MRI can depict remodeling processes in the nonossified humeral head early in the course of the disease (Fig. 5).
4.3 Trunk Neuropathies
In the following section MRI appearances of the most common nerve lesion patterns of the upper extremity will be described. However, this overview cannot cover the complexity of all upper extremity nerve lesions in every detail. For more detailed descriptions, the reader is referred to the cited reference articles.
4.3.1 Musculocutaneous Nerve Neuropathies
Isolated lesions to the musculocutaneous nerve are rare. They are mainly posttraumatic or exercise related, occasionally entrapment of the nerve is observed in the region, where the nerve penetrates the coracobrachial muscle.
In particular, MRI at 3 T promises to be a valuable tool in the identification and characterization of isolated musculocutaneous nerve lesions, as the nerve itself can be successfully visualized (Chhabra et al. 2012). The musculocutaneous nerve arises from the lateral cord and its motor innervations comprise the coracobrachial, biceps, and brachial muscles. However, denervation associated MR signal changes of the brachial muscle is frequently incomplete, since in the majority of cases [67–100 %, reviewed in (Puffer et al. 2011)] its inferolateral portion is supplied by the radial nerve.
4.3.2 Radial Nerve Neuropathies
The most frequent site of radial nerve injury is the spiral groove of the humerus. In this region, the nerve is mainly injured by two mechanisms: (1) Due to its close anatomical proximity to the humerus, upper extremity trauma causing fractures, frequently leads to radial nerve lesions. (2) Because of its attachment to the surrounding soft tissue at its penetration site through the lateral intermuscular septum, the radial nerve is particularly vulnerable to compression/entrapment injuries (“Saturday night palsy”) in this region (Fig. 6).
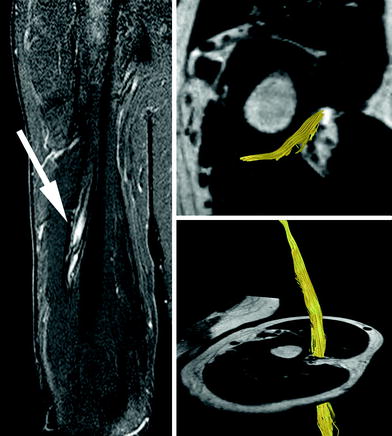
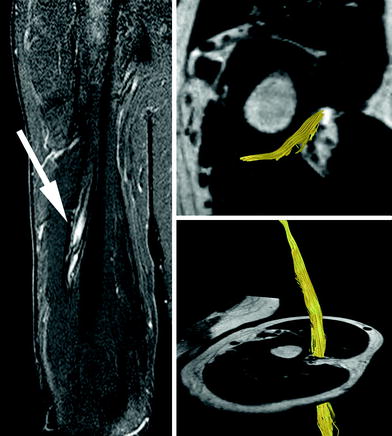
Fig. 6
Coronal STIR (short tau inversion recovery) sequence (left) and 3D tractography analysis of the radial nerve in a patient with Saturday night palsy (right). Please note the signal increase of the entrapped radial nerve at the spiral groove of the humerus (solid white arrow, left). However, tractography detects intact trajectories ruling out severe internal injury of radial nerve or true neuroma formation
Lesions of the radial nerve at this level lead to denervation of the hand extensor muscles. However, since its innervation occurs proximal to the spiral groove, the triceps muscle is generally not denervated in the typical upper arm radial nerve lesions.
The neurogenic “radial tunnel syndrome” affects the purely motor posterior interosseous branch of the radial nerve. Over a distance of 5 cm this radial nerve branch is crossed by potentially compressing fibrous bands, branches of the radial artery, and the edges of the supinator muscle (Rosenbaum 1999). The proximal edge of the supinator muscle is found to be tendinous in half of all individuals (Arcade of Frohse), and may represent a potential compression site (Spinner 1968). The isolated neurogenic posterior interosseous nerve (PIN) compression manifests clinically as radial deviation of the hand, weakness in the extension of the wrist and of all digits (in a complete lesion), and weak extension of fourth and fifth digits (in a partial lesion) without any sensory loss. The pure PIN compression has to be differentiated from the clinical presentation of the “disputed” radial tunnel syndrome, which is dominated by pain in the region of the lateral epicondyle and is observed in patients with recurrent epicondylitis (Werner 1979; Rosenbaum 1999). However, in both conditions denervation signs of the PIN innervated muscles occur and can be detected by MRI in more than 50 % of cases (Ferdinand et al. 2006) (Fig. 7). The most frequently affected muscle showing denervation edema in this condition is the supinator muscle (Ferdinand et al. 2006) (Fig. 7).
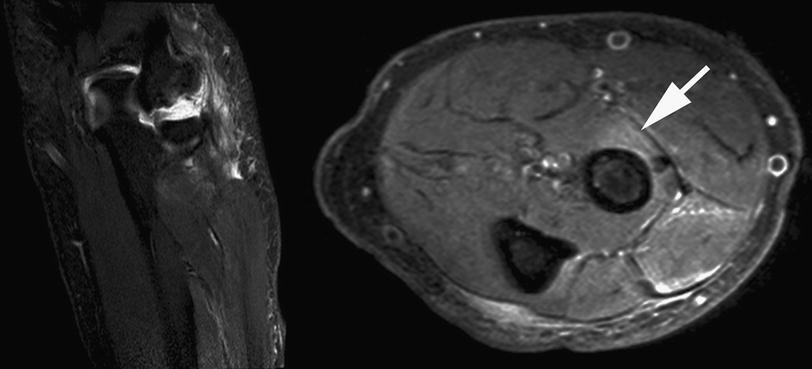
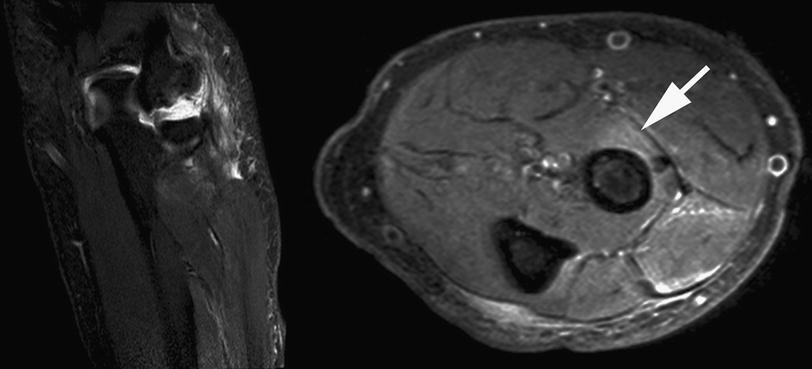
Fig. 7
Coronal STIR (short tau inversion recovery) and axial Proton Density sequences of the proximal forearm in a patient with lateral epicondylitis. Please note the extent of signal changes, affecting the proximal wrist extensor muscles and the supinator muscle (arrow). Moreover, increased signal intensity at the site where the posterior interosseous nerve pierces the supinator muscle (white solid arrow, Frohse Arcade, a fibrous arch over the posterior interosseus nerve and the most superior part of the superficial layer of the supinator muscle) is identified. These findings confirm the presence of an additional posterior interosseous nerve syndrome
4.3.3 Ulnar Nerve Neuropathies
The most common site of ulnar nerve injury is the cubital tunnel. After the carpal tunnel syndrome, the cubital tunnel syndrome (CUS) is the second most common nerve entrapment syndrome. As the ulnar nerve passes the intramuscular medial septum and the medial head of the triceps muscle it may be compressed by a locally thickened ligament (sometimes also referred to as the Struther′s arcade) (Wehrli and Oberlin 2005). The cubital tunnel per se is an osteofibrous canal, which is formed by the bony retrocondylar sulcus of the medial epicondyle proximally. Then it is defined by the course of the nerve underneath a retinaculum proximally and further distally underneath the arcuate ligament of Osborne and finally it is covered by the deep aponeurosis of forearm flexor muscles (Assmus et al. 2009). In 10–23 % of cases muscle fibers support the retinaculum—referred to as epitrochleoanconeus muscle (Campbell et al. 1991; Husarik et al. 2009). The most distal point of potential entrapment of the ulnar nerve is the Loge de Guyon at the wrist. Recent MRI data at 3 T could impressively demonstrate the high sensitivity of MRI in detecting signal changes of the deep motor branch of the ulnar nerve in Guyon’s-canal-syndrome (Kollmer et al. 2012).
However, the CUS is by far more common than the distal entrapment neuropathy of the ulnar nerve. The ulnar nerve physiologically shows de- and remyelinating changes as response to the exposure to significant mechanical forces, which are most accentuated in the region of the cubital tunnel (Bozentka 1998). Therefore, a clear distinction between subclinical CUS and changes within the normal spectrum of nerve lesions is difficult. This is also reflected by the controversial data on ulnar nerve signal changes in this region. A high accuracy in the definition of CUS was reached in the so far largest study published on the use of MRI in CUS by measuring the T2-weighted signal intensity of the ulnar nerve (Baumer et al. 2011) (Fig. 8). Moreover, signal changes of the ulnar nerve did also correspond to the clinical severity of CUS (Baumer et al. 2011). As increases in ulnar nerve signal intensity may occur in the cubital tunnel of asymptomatic subjects (Husarik et al. 2009), the radiological assessment of ulnar nerve signal changes has to respect the clinical context and further imaging findings. In severe cases of CUS, STIR sequences may depict denervation edema of the flexor carpi ulnar and ulnar bulk of the deep forearm flexor muscles (Fig. 9). Moreover, reversal of these signal changes indicates reinnervation and treatment success (Fig. 9) (Viddeleer et al. 2012). In cases, where decompression surgery does not lead to improvement of clinical symptoms, the ulnar nerve may show widespread signal abnormalities, which are not confined to the cubital tunnel itself and may indicate a noncompressive etiology (Baumer et al. 2012). Finally, DTI and tractography may add further topographical information on the exact site of ulnar nerve compression and help to tailor the surgical approach (Fig. 9).
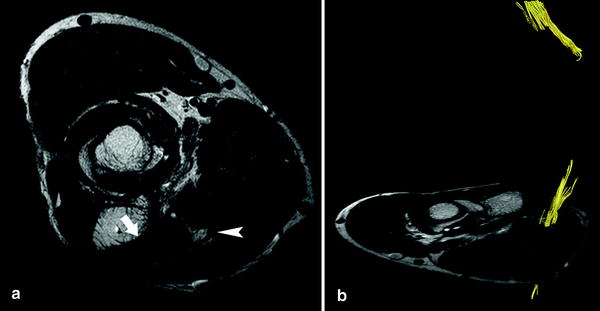
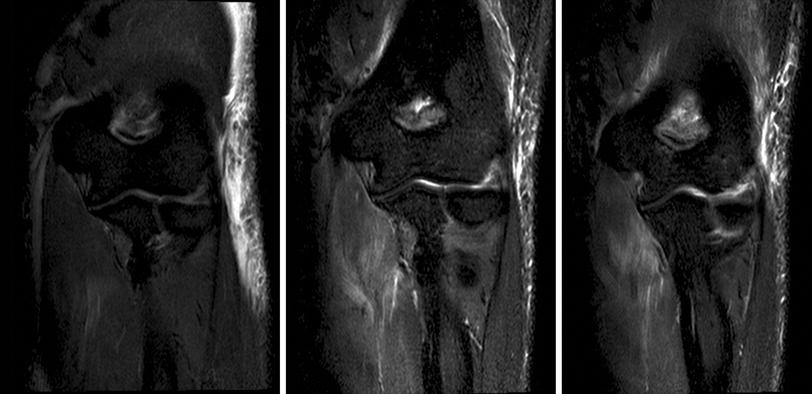
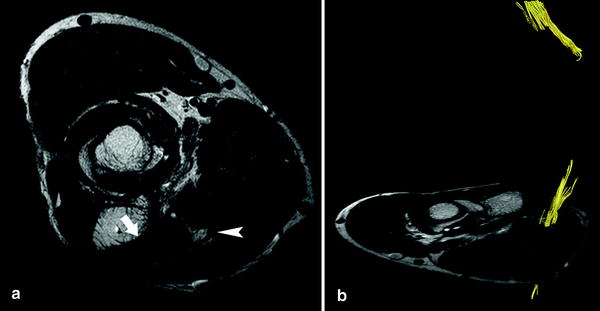
Fig. 8
Axial T2-weighted a sequence in a patient with severe cubital tunnel syndrome: please note the denervation edema of the flexor digitorum profundus muscle (arrow) and the increase in signal intensity of the ulnar nerve (arrowhead). Tractography b is unable to track the axonal trajectories of the ulnar nerve in the region of the cubital tunnel
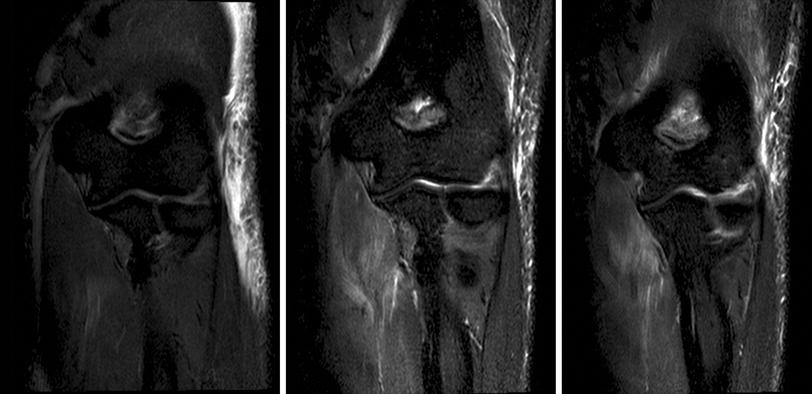
Fig. 9
Sequential coronal STIR (short tau inversion recovery) sequences 2 days after complete iatrogenic neurotmesis of the ulnar nerve during an arthroscopy procedure (left). The middle image shows a STIR sequence of the same patient 4 weeks after surgical repair, and 5 weeks after injury. The right image shows the follow up results 4 months after the lesion. Please note the maximum signal intensity changes of the denervated flexor digitorum profundus muscle to be present 5 weeks after denervation (middle image). Normalization/reduction of denervation associated signal changes (right image) correlated well with clinical improvement after surgical repair and reinnervation
4.3.4 Median Nerve Neuropathies
Entrapment of the median nerve within the carpal tunnel constitutes the most common entrapment neuropathy. In most cases the clinical and electrophysiological diagnosis of the carpal tunnel syndrome (CTS) is not challenging. Imaging mainly serves to identify or rule out mass lesions such as lipoma, synovitis, neuroma, hamartoma, or deformities of the wrist, which negatively impact surgical outcome [reviewed in (Wilson and Allen 2012) and (Kim et al. 2007)]. Surgical complications after carpal tunnel release are reported to occur in the range of 3–19 % (Stutz et al. 2006). In these cases reevaluation by MRI is helpful, since scarring and postoperative fibrosis of the median nerve may be optimally objectified and extensive reexploration can be avoided (Campagna et al. 2009).
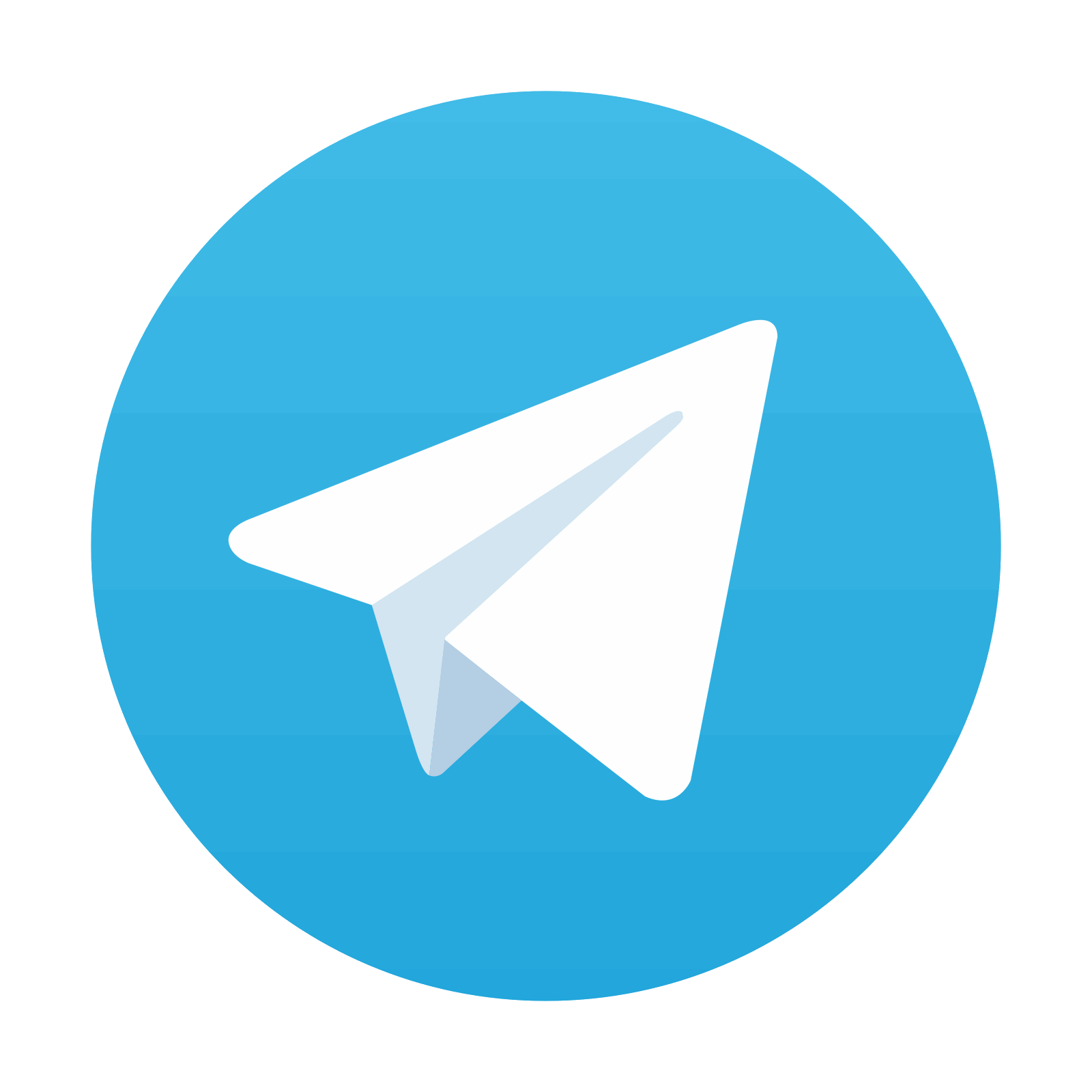
Stay updated, free articles. Join our Telegram channel

Full access? Get Clinical Tree
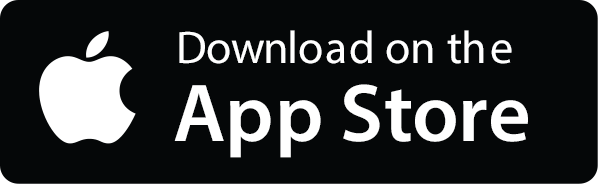
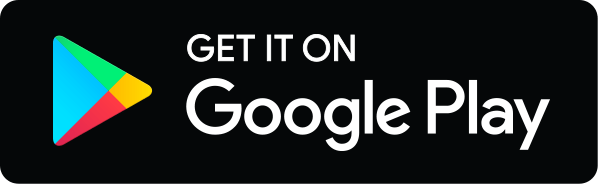