Fig. 1
Volume rendering computed tomography in false colors showing the macroscopical anatomy of the muscles of the abdominal wall, including the rectus abdominis muscle
Pennate muscles include triangular-shaped (e.g., adductor longus), unipennate or semipennate (e.g., flexor pollicis longus), bipennate (e.g., rectus femoris), multipennate (e.g., deltoid), and circumpennate (e.g., tibialis anterior) muscles.
Some muscles are bipennate and their fascicles join a single central tendon; multipennate muscles have more than one tendon coursing through the muscle substance. Fascicles may also present a curved spiral arrangement between the origin and the insertion (e.g., pectoralis major, supinator). Several muscles are composed of a single belly or may have a complex internal architecture made up of multiple heads with a different origin (e.g., two heads for the biceps brachii and the biceps femoris; three heads for the triceps brachii and the triceps surae) and join together to generate a distal tendon (Fig. 2a–d).
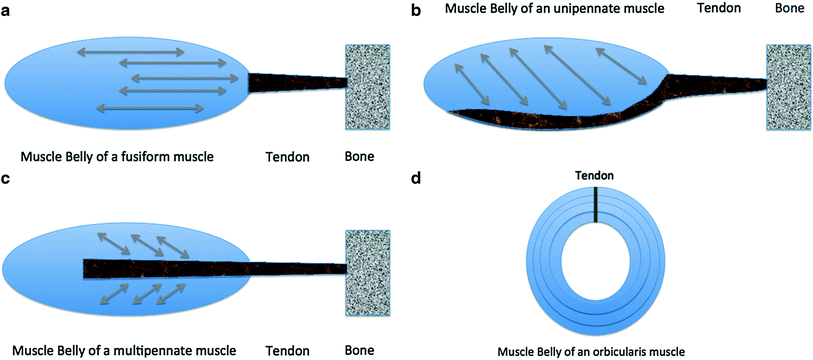
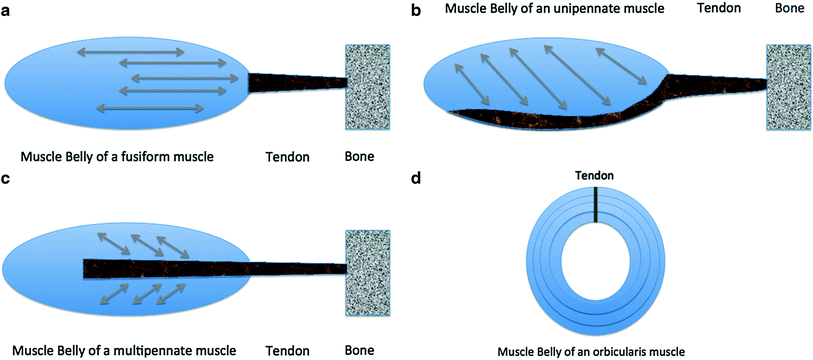
Fig. 2
Schematic drawing of common skeletal muscle anatomy. a Fusiform muscle. The fascicles are parallel to the mid-portion of the muscle belly (low strength but high velocity and range of movement). b Unipennate muscle. The fascicles insert on one side of the aponeurosis (great strength but low range of shortening). c Bipennate muscle. The fascicles insert on two sides of a central aponeurosis (great strength but low range of movement). d Orbicularis muscles. These muscles contract concentrically and may not have a real bony insertion but a strong tendinous insertion
From the biomechanical point of view, during muscle contraction, the force is transmitted to the skeleton by the tendon or aponeurosis and may or may not result in joint motion. Skeletal muscle contractions may be divided into three types: isometric, when the muscle contracts, but there is no change in its length; isotonic or concentric, when the muscle contracts and simultaneously shortens; and eccentric, when the muscle contracts and at the same time lengthens.
When a muscle is activated by the nervous system and it is required to lift a load which is less than the maximum tetanic tension the muscle can generate, the muscle is able to shorten. Contractions that permit the muscle to shorten are referred to as concentric or isotonic contractions. An example of a concentric contraction is the lifting of a weight during a biceps curl. In concentric contractions, the force generated by the muscle is always less than the muscle’s maximum force. As the load the muscle is required to lift decreases, contraction velocity increases. This occurs until the muscle finally reaches its maximum contraction velocity.
In eccentric contractions the muscle actively lengthens. During normal activity, muscles are often active while they are lengthening. Classic examples of this are walking, when the quadriceps (knee extensors) are active just after heel strike while the knee flexes, or setting an object down gently (the arm flexors must be active to control the fall of the object).
As the load on the muscle increases, it finally reaches a point where the external force on the muscle is greater than the force that the muscle can generate. Thus even though the muscle may be fully activated, it is forced to lengthen due to the high external load. This is referred to an eccentric contraction. There are two main features to remark regarding eccentric contractions. First, the absolute tensions achieved are very high relative to muscle’s maximum tetanic tension generating capacity (you can set down a much heavier object than you can lift). Second, the absolute tension is relatively independent of lengthening velocity. This suggests that skeletal muscles are very resistant to lengthening. The basic mechanics of eccentric contractions are still a source of debate, since the cross-bridge theory, which so nicely describes concentric contractions, is not as successful in describing eccentric contractions. Many muscle injuries and delayed soreness are often associated with eccentric contraction. Moreover, muscle strengthening may be greater using exercises that involve eccentric contractions. It has been recently demonstrated that eccentric contractions that normally determine an injury may stimulate the activation and proliferation of satellite stem cells to guide skeletal muscle regeneration. For this reason therapeutic strategies to combat disuse muscle atrophy may be implemented by the use of eccentric contractions in a rehabilitative setting (Valero et al. 2012). During isometric contraction the muscle is activated, but instead of being allowed to lengthen or shorten, it is held at a constant length. An example of an isometric contraction would be carrying an object in front of you. The weight of the object would be pulling downward, but your hands and arms would be opposing the motion with equal force going upwards. Since your arms are neither raising nor lowering, your biceps will be isometrically contracting. The force generated during an isometric contraction is dependent on the length of the muscle while contracting. Maximal isometric tension is produced at the muscle’s optimum length, where the length of the muscle’s sarcomeres is on the plateau of the length-tension curve.
Sometimes, a fourth type of muscle “contraction,” known as passive stretch, is described. As the name implies, the muscle is being lengthened in a passive state (i.e., not being stimulated to contract) (Fig. 3a–d). An example of this would be the pull one feels in their hamstrings while touching their toes. The usefulness of stretching is still a controversial topic. Several studies investigated optimal stretching techniques causing confusion among researchers and clinicians. Further investigations may be useful to determine how passive and active muscular stretch may improve muscular function as a whole and eventually prevent musculoskeletal derangements (Williams et al. 2013). Examples of muscular contractions on ultrasound (US) examinations are demonstrated in three video clips (VIDEO 1–3) which can be accessed and downloaded via www.extras.springer.com. Representative snapshots of the video clip for isometric contraction are given in Fig. 4.
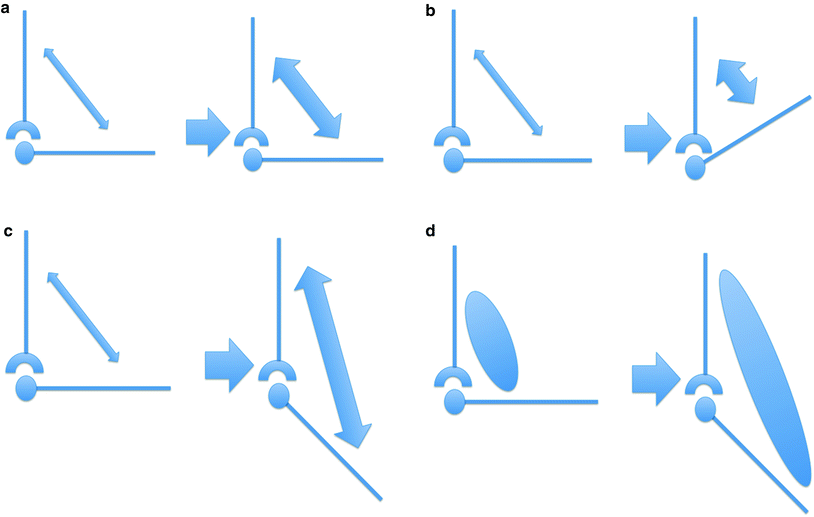
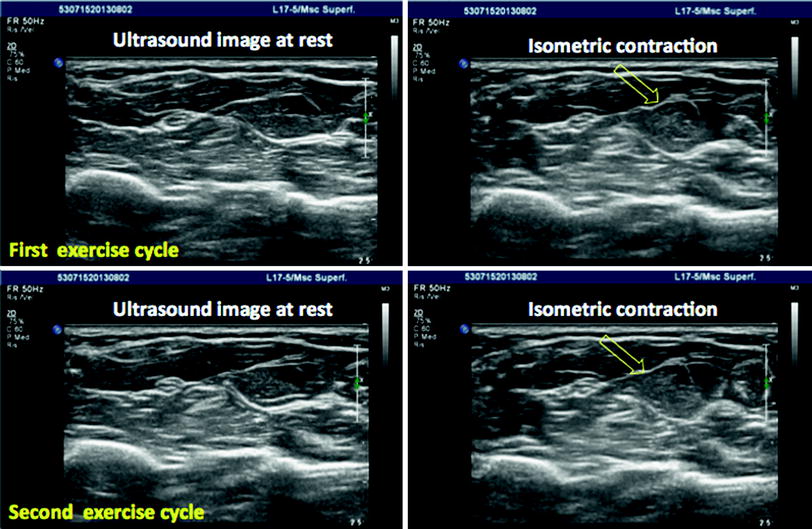
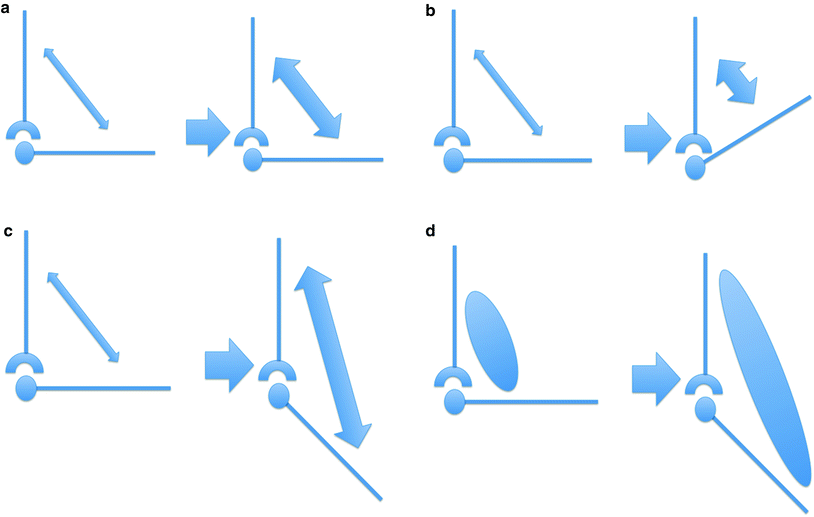
Fig. 3
a Isometric contraction. The length of the muscle does not change during contraction. b Isotonic contraction. The muscle shortens as it contracts. A typical example of isotonic contraction is the flexion of the arm on the forearm. c Eccentric contraction. The muscle lengthens during contraction. d Passive stretching. The muscle is lengthened in a passive state without active contraction
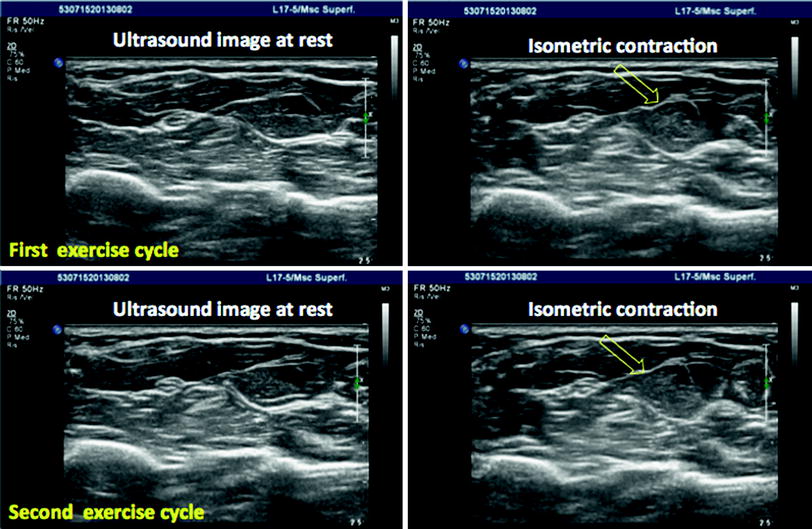
Fig. 4
Examples of different forms of muscular contractions on real-time ultrasound examinations are demonstrated in the three video clips (isometric contraction, isotonic contraction, and passive stretching) which can be accessed and downloaded via www.extras.springer.com. Representative snapshots of the video clips are given for isometric contraction on two subsequent exercise cycles to show the potential of ultrasound to show muscular motion in real-time. The open arrows show the time point of contraction
5 Physiological Notes on Muscular Contraction
From the physiological point of view the musculoskeletal system moves the different segments with respect to each other following the principle of levers. Each muscle is able to provide stability and to produce movement of the levers (bone) around the fulcrum (joint).
The system of levers is divided into three types on the basis of the organization and the distance between the fulcrum to the point of effort and to the load point. We can find all three classes of levers in body muscles.
First Class: The fulcrum lies between the effort and the load. In the human body, a lever of the first class can be found at the level of the head, for example. The weight of the face and of the head may be considered the resistance. The contraction of the posterior neck muscles may be considered the effort to lift the weight. A lever of the first class has a twofold purpose: to increase the speed of movement and to overcome the resistance. In this case the resistance (load) is moved in the opposite direction.
Second Class: The load lies between the fulcrum and the effort. The classical example reported in several textbooks is the principle of the wheelbarrow. A small upward force applied to the handles can overcome a much larger force (weight) acting downwards in the barrow. Similarly, a relatively small muscular effort is required to raise the body weight. In the human body, a lever of the second class can be found in our feet when we stand on our toes and lift our heels off the ground. The resistance (load) is the weight of our body resting on the arch of the foot. The effort is brought about by the contraction of the calf muscle attached to the heel. This leverage allows us to walk. The main purpose of a lever of the second class is to overcome the resistance.
Third Class: The effort lies between the fulcrum and the load. In the human body, a typical example of a lever of the third class is when the biceps contracts, allowing us to lift an object. The elbow may be considered the fulcrum, the object is the resistance (or load), and the biceps muscles contraction is the effort. The load can be moved rapidly over a large distance, while the point of application moves over a relatively short distance. The main purpose of this type of lever is to obtain rapid movement. Human skeletal muscles are shaped also according to their action as levers (Fig. 5a–c).
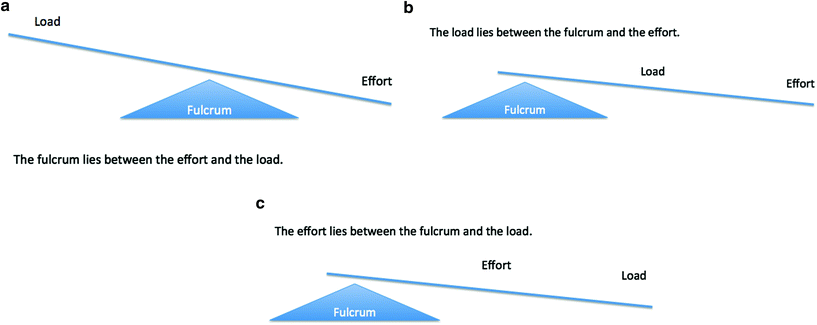
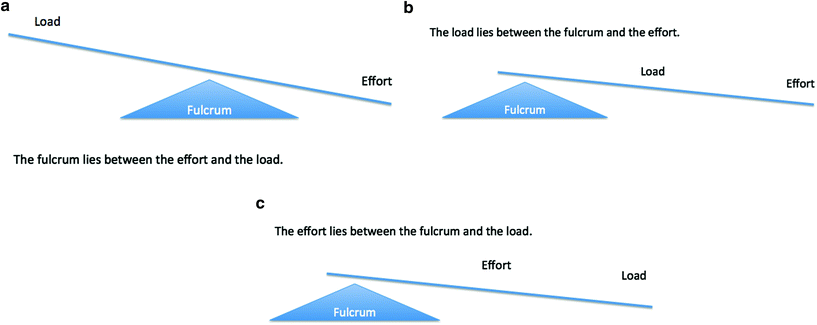
Fig. 5
Principle of levers. a First class lever. b Second class lever. c Third class lever
6 Tendons
Tendons are non-contractile collagenous structures with the function of connecting the muscle to its bony insertion. Tendons are important to transmit forces generated by muscle contraction, to facilitate and to maintain the posture. Several skeletal muscles have both a tendinous insertion and a tendinous origin. Tendons are round or oval in cross-section and are made mainly of type I collagen fibers. These collagen fibers have the task to transmit the force with the minimum area, therefore the fibers are orientated parallel to the long axis of the tendon. Vessels and nerves course within loose connective tissue between the tendon fascicles. Some tendons are surrounded by a synovial sheath, especially when the corresponding muscle has the function to move bony segments for long distances (for example at the level of the fingers). Tendons attach to the periosteum of the bone through fasciculi that continue directly into the cortex of the bone. A plate of fibrocartilage at the site of bony attachment serves to cushion and reinforce the site of attachment. In general, tendons have a variable length according to the muscle of origin. Some tendons may have an intramuscular component or a superficial component resulting in a variable length of the myotendinous junction. Type I collagen fibers of the tendon are accompanied by a few amount of elastic fibers. These elastic fibers are important to increase the resilience and resistance of the tendon. The elastic properties of the tendons allow an elongation up to 6 % during maximum loads preventing damages during movements. Concerning the vascular supply of the tendons it is well known that they have a limited blood supply. This anatomical feature predisposes certain tendons to some typical injuries (e.g., the supraspinatus and the Achilles tendon). Each tendon receives its vascular supply from segmental vessels arising from the surrounding peritendon or, from folds of the mesotendon for those tendons with synovial sheaths (http://www.wheelessonline.com).
7 Aponeuroses
Aponeuroses are connective structures that may be considered flattened tendons. Aponeuroses may be considered separate structures emerging from or within a muscle belly or its surface (for example the tendons of latissimus dorsi and pectoralis major or the aponeurosis of the quadriceps femoris muscle).
8 Muscle–Tendon Junction
The muscle–tendon junction (musculotendinous or myotendinous junction) is the anatomical site where the muscular tissue becomes tendon. This site may be considered a weak area since the majority of traumatic lesions occur at this site. From the microscopical point of view, at this level tendon fibrils insert into deep recesses between finger-like processes of skeletal muscle cells covered by a thick layer of basement membrane. The tendon fibrils attach to the basement membrane of the skeletal muscle cells. Myocytes and tendons fibrils are linked by means of interdigitation to have an increased contact area able to distribute the tensile forces during contraction. The muscle–tendon junction has negligible elastic content and for this reason it has a lower resistance to loads during movement.
9 Imaging
Diagnostic imaging commonly available in clinical practice is able to study the muscles, the tendons, and the bone from a macroscopical point of view in normal and pathological conditions. US, MRI, and CT are widely used with different purposes to visualize the normal anatomy of the musculoskeletal system. Moreover, in recent years, new and technologically advanced imaging techniques have been introduced to study the musculoskeletal anatomy from a physiological and microscopical point of view. For example, elastography has been introduced to assess the elastic properties of tendons and muscles. Using MRI, diffusion tensor imaging (DTI) has been introduced to study the architecture of the skeletal muscle (see also “Correlation of Skeletal Muscle Anatomy to MRI and US Findings” of this book). The DTI technique is based on the measurement of the apparent diffusion of water in a (biological) tissue (Van Donkelaar et al. 1999). It is concluded that DTI fiber directions resemble fascicle directions visible in high-resolution images very well. Moreover, on DTI images it is possible to observe and study specific features of the skeletal muscle such as the pennate insertion on the aponeuroses and the pennation angle. DTI has the potential to be introduced in biomechanical research on skeletal muscle function.
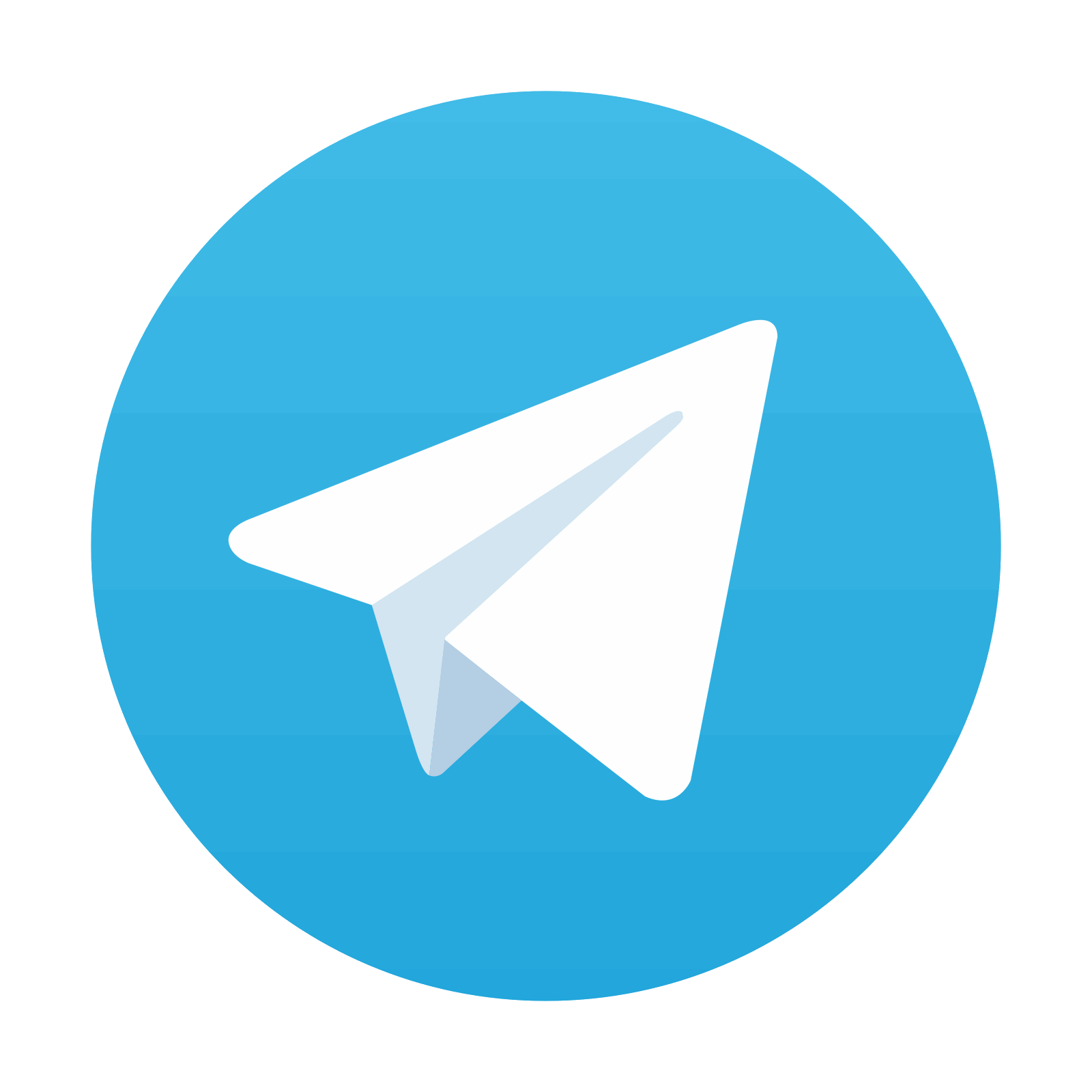
Stay updated, free articles. Join our Telegram channel

Full access? Get Clinical Tree
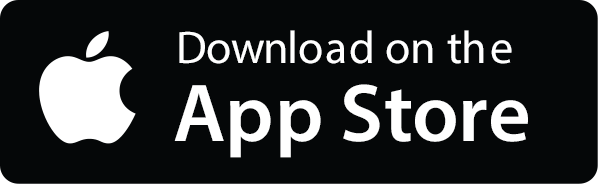
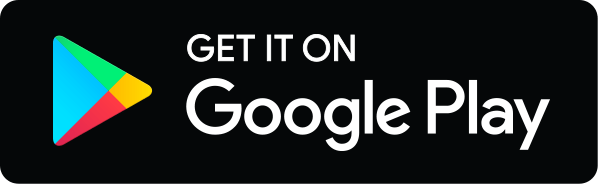