Fig. 1
Diagram of microvasculature (with permission from Ross et al. 2003)
3 Histology of Microvasculature
-
Capillaries: structurally the fundamental vascular units are simple endothelial tubes connecting terminal arterioles or precapillaries and venules. There is usually a semi fluid intercellular cement between the endothelial cells of the capillary, and only a delicate reticular network surrounds the endothelial tube. Outside their walls may be found fixed macrophages and fibroblasts. The average diameter of normal blood capillaries is about 8 microns; thus erythrocytes are permitted to pass in single file. The largest normal capillary has a diameter of about 12 microns. Capillaries form networks which may be flattened or spongy and vary in shape, conforming to the character of the region they supply. The closeness of the mesh generally varies directly with the intensity of the tissue metabolism in the region supplied. Lymphatic capillaries are somewhat broader than blood capillaries and not as uniform in caliber, showing dilatations and constrictions. Their walls are composed of endothelium alone, embedded in connective tissue. These capillaries form dense networks roughly paralleling networks of blood capillaries, and they are often close neighbors of blood capillaries. They are found in nearly all parts of the body. Near surfaces, such as the skin and internal membranes, they often begin as loops or as blind swollen tubules. There are certain other small blood channels resembling capillaries in some respects, but which can be distinguished from them. Prominent examples are sinusoids and the rete mirabile.
-
Sinusoids are special channels between larger vessels. Some of them connect arterioles with venules, as in the spleen, adrenal cortex, and bone marrow. Others connect venule with venule, as in the liver and in the anterior lobe of the pituitary gland. There are also lymphatic sinusoids which interconnect lymphatic vessels, as in the lymph nodes. Sinusoids are relatively wide, up to 30 microns, and are not uniform in caliber. They are lined by an incomplete layer of scattered fixed microphages that project into the lumen, by flat cells that are apparently potential macrophages and by cells in every transition between these two forms. These lining cells are separated from the surrounding parenchyma only by a network of reticular fibrils.
-
The rete mirabile is a capillary-like plexus inserted in the course of an arteriole or venule, with an afferent arteriole or venule carrying blood to it and efferent arteriole or venule draining it. An example is the renal glomerulus, and similar structures are the sinusoids of the anterior pituitary (venule-sinusoid-venule) and of the lymph nodes (lymphatic-sinusoid-lymphatic).
-
Precapillaries are vessels intermediate between capillaries and arterioles or venules. They are larger than capillaries but have incomplete accessory coats as compared with arterioles and venules. Arterial precapillaries are less than 40 microns in diameter. The smallest of them consist of an endothelial tube surrounded by scattered smooth muscle fibers; the largest are, in addition, surrounded discontinuously by connective tissue cells and fibers. Venous precapillaries are less than 200 microns in diameter; the smallest consist of endothelium surrounded by scattered connective tissue elements, and the larger ones are, in addition, surrounded discontinuously by smooth muscle fibers. With the smooth muscle in the precapillaries to act as sphincters, the control of vessel size and blood flow through the capillary beds becomes possible.
-
Arterioles: All blood vessels larger than precapillaries exhibit a common generally structural organization. However, each specific type of vessel shows characteristic adaptive variations in which certain features of the general plan may be emphasized, minimized, or omitted, or certain new features may be added. Every typical blood vessel larger than a precapillary is composed of three concentric coats of tunics, namely, the tunica intima or interna, the tunica media, and the tunica adventitia or externa. The tunica intima consists of an endothelium (simple squamous epithelium) bounding the lumen, a subendothelial coat of delicate fibroelastic tissue and a fenestrated internal elastic membrane, which is the outermost component of the intima. The tunica media consists primarily of smooth muscle circularly arranged, with variable amounts of elastic fibers, or circular elastic networks, or concentric elastic tubes in certain large vessels. The tunica adventitia, the outer coat, usually contains a concentration of elastic tissue as an elastic layer, and close to the tunica media there may be a definite external elastic membrane. The remainder of this coat consists of moderately compact fibroelastic tissue which merges gradually with areolar connective tissue. Blood vessels most than 1 mm in diameter contain nutrient vessels called vasa vasorum. In arteries these are usually limited to the tunica adventitia, but in veins they may extend through the tunica media. Lymphatic vessels are also present in the walls of the larger blood vessels. The arteries include arterioles, the smallest arteries (40–300 microns in diameter), which are predominantly muscular; small to medium–sized arteries, which are predominantly muscular; and large arteries, which are predominantly elastic. The transition from muscular to elastic composition is usually gradual, and there are intermediate mixed types. The size and composition of arteries are not always typically correlated. The arterioles have relatively thick walls and narrow lumens, the walls being thicker relative to the caliber of the lumen than in any other type of blood vessel. As compared with arterioles, venules have a large diameter (between 200 and 100 microns) but a relatively thin wall and broad lumen. The tunica intima consists only of an endothelial layer, the thin tunica media is almost exclusively smooth muscle, and the tunica adventitia is a relatively thick component of the very thin wall. An arterial lumen is always smaller than that of its companion vein of the combined lumens of its companion veins, and the arterial wall is thicker, more rigid, and more resistant to collapse.
4 Physiology of Microcirculation
The microcirculatory apparatus is of special importance in any consideration of damage and recovery of organs because of its intimate and vital supportive relationship to the metabolism of tissues and tissue components. The fine blood vessels, and usually the fine lymphatic vessels also, enter into the composition of most organs. The one-way microcirculatory flow carries nutrients, oxygen, hormones, and blood cells to all parts of the body, and collects metabolic wastes from the tissues and transports them to the kidneys or, in the case of carbon dioxide, to the lungs.
Capillaries are the most important of all vessels functionally, since they are in a close relationship with the various tissue elements at the site of cellular metabolism, where they deliver nutrients and oxygen and receive wastes, their thin walls and slow blood flow favor diffusive interchanges. Capillary blood flow varies in amount and extent with tissue activity, being intermittent in resting or relatively inactive tissues and voluminous and extensive in active tissues. Although capillaries are closed tubes they are normally semi permeable. Water, gases, salts, and nutrients in simple form are among those substances which pass through their walls, but most colloids and all microscopically visible particles do not normally pass through. However, the degree of permeability of capillary walls varies regionally and also with local changes in condition. Leukocytes occasionally pass through the endothelium under normal conditions, and when the endothelium is altered, as in inflammatory conditions, the passage of leukocytes may be greatly increased; even erythrocytes may escape from the blood through the altered endothelium. True endothelial cells are not normally phagocytic, as are the cells lining the sinusoids.
The muscular arteries can regulate the blood flow to any part of the body according to its changing needs by means of nervous control of the muscular layer of the vessels. The arterioles, with their relatively thick walls and narrow lumens, are the chief controllers of systemic blood pressure and local blood flow. Most of the decline in blood pressure in the mascular system occurs within the arterioles, so that the delicate capillaries normally receive blood gently in a slow stream.
Endothelial cells normally divide from time to time with moderate frequency to replace those cells that die and are lost. They are capable of much more rapid proliferation under pathologic conditions, e.g., during recovery from local injuries to the vasculature and during neovascular formation in the healing of wounds or lesions. In neovascular formation, the endothelium from capillaries forms solid sprouts which enter injured and regenerating areas. The sprouts become hollow, fibroblasts are at hand, myoblasts soon differentiate, and accessory coats are formed around the endothelial tubes as needed.
The aging arteries seem to be a physiologic process that passes gradually into arteriosclerosis. Each type of artery normally shows a characteristic pattern and schedule of change with increasing time.
5 Pathogenesis
A discussion of the radiation effects on endothelial cells should be preceded by some review of the complex physiology of the endothelium. Aside from those activities common to all cells, certain specialized functions are characteristic of Endothelial cells (EC):
-
EC are the most radiosensitive among the fixed elements of the mesenchyme. Depending on dose, ionizing radiation can produce lethal or sublethal injury to EC. The latter may alter considerably the complex physiology of the endothelium. Some functions are inhibited or abolished: fibronolysis, synthesis of various enzymes and cytokines, attachment of EC to the basal lamina, angiogenesis, etc. Other functions are enhanced, including permeability, soluble coagulation, platelet adhesion, and aggregation. There is also upregulation of adhesion molecules for leukocytes. Endothelial cells are heterogeneous; accordingly, radiation effects vary in quality and severity from one site to another, and from one animal species to another.
-
Coagulation: EC participate in the soluble coagulation system by producing both procoagulants (e.g., tissue factor and Factor V) and anticoagulants (e.g., plasminogen activators and thrombomodulin.). In addition, EC regulate the adhesion and aggregation of platelets through von Willebrand Factor (vWill F), nitric oxide, etc.
-
Permeability: transport of certain molecules across the EC cytoplasm.
-
Inflammation and immune response: EC express multiple antigens, including MHCs I and II, and ABO. Several cytokines are produced in EC, such as IL1 and GM-CSF. Depending on activation state, lymphocytes, granulocytes, and macrophages adhere to specific EC receptors.
-
Synthesis of stromal components: EC produce their own basement membrane (mainly collagens IV, V, and laminin), as well as various collagens for the surrounding tissue matrix.
-
Vascular tone regulation: through angiotensin converting enzyme and endothelin, EC contract smooth muscle while nitric oxide relaxes it.
-
Angiogenesis: this, the formation of microvessels in the fully developed vertebrate, is the most dynamic function of the endothelium; it occurs in response to a large number of agonists and antagonists. It is either physiologic (e.g., in wound healing and cyclical endometrial growth) or pathologic (e.g., in neoplasia and many inflammatory disease).
The above, and other endothelial cell functions, are regulated by numerous genes, many of which have been characterized. EC vary greatly from tissue to tissue and from one animal species to another. This heterogeneity is evident morphologically, functionally, and in response to injury. Figure 2 outlines diagrammatically the most important of the functions characteristic of EC (compare with Fig. 3) (Fig. 2) (Fajardo 1989).
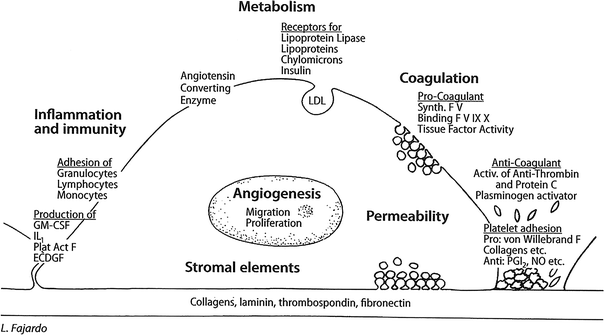
Fig. 2
Physiology of normal endothelial cell a normal endothelial cell attached to its basal lamina (bottom), which is partially denuded on the right. The main functions described here are indicated in bold uppercase letters, with examples in lowercase. ABO, blood antigens; ACE, angiotensin converting enzyme; GM-CSF, granulocyte–monocyte colony stimulating factor; LDL, low density lipoproteins; MHC I & II, major histocompatibility complexes; NO, nitric oxide; PGI 2,prostacyclin (Reproduced , with permission, from (Fajardo 1989))
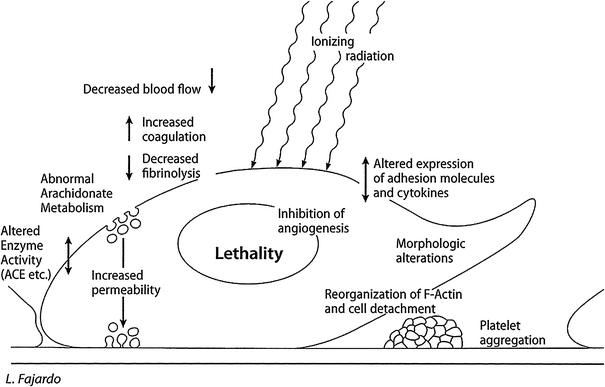
Fig. 3
Radiation effects of radiation on endothelial = F3.2 P21 CURED I LENT; general effects of radiation on endothelial cells. This is a diagrammatical summary of the most important , lethal, and sublethal effects of ionizing radiation on endothelial cells. It combines in vitro and in vivo data and is based on multiple sources of information (Reproduced, with permission, from (Fajardo 1998))
The impact of ionizing radiation on the endothelium has been studied in vivo and in vitro, the latter using various EC lines, including human umbilical vein cells (HUVEC), bovine aortic cells (BAEC), or capillary EC (e.g., HDMEC). The doses varied between < 1 Gy and as much as 60 Gy, with various fractionation schemes. For the in vitro studies, the doses were often 5 Gy or less. From a review of various in vitro experiments (too many to list here), it appears that radiation becomes lethal to endothelial cells when it reaches Do values of the order of 100–200 cGy in the clonogenic survival curves (higher values are required in vivo).
EC may undergo mitotic death or apoptosis, the latter through a pathway that probably involves the formation of ceramide.
Sublethal doses of radiation affect the morphology and various functions of EC.
Common morphologic changes include hypertrophy of EC associated with reorganization of F-actin filaments, and detachments from the basement membrane. The in vivo changes include vascular constrictions, thromboses, and rupture of microvascular walls with resulting hypoperfusion. Most studies show an increase in permeability for various molecules (however, serotonin transport is decreased). There is hypercoagulation, and platelet aggregation due to enhanced release of vWill F, causing an increased tendency to thrombosis. In addition, ineffective fibrinolysis results from a decrease in plasminogen activators. The eicosanoid metabolism is altered, with early decrease and late increase in PGI-2. There is enhanced chemoattraction for leukocytes and upregulation of adhesion molecules (e.g., ELAM-1). EC show decrease in endothelial enzyme activity (e.g., angiotensin converting enzyme, alkaline phosphatase). Radiation inhibits angiogenesis: the magnitude of this effect depends in part on the sequence of angiogenic stimulus versus radiation. Various data suggest that the inhibition of angiogenesis is greater when the radiation exposure occurs prior to the angiogenic stimulus instead of following it. This information may be important when designing the sequence of radiation therapy versus surgery (the angiogenic stimulus, and depends on the timing of radiation with regard to the time interval for surgery.
Like other normal cells, EC have some innate protection from ionizing radiation. For instance, glutathione and superoxide dismutase provide some defense of EC from reactive oxygen species (e.g., hydroxyl radical and superoxide respectively). Nevertheless, it appears that endothelial cells are the most radiosensitive elements in the vessel wall. They may even be the most sensitive among the fixed cells of the mesenchyme. Many of the studies suggest that EC are more radioresponsive in vitro than in vivo. Sublethal endothelial radiation injury not only contributes to the very early, acute effects, but also accounts for many of the delayed effects, such as stromal fibrinous exudate and ischemia.
6 Capillaries and Arterioles
There are differences in radiation response between small and large vessels. The endothelial tissue of the small blood vessels seems to be moderately sensitive to ionizing radiation. Its radiosensitivity seems to be intermediate between the highly radiosensitive parenchymal tissues containing vegetative and differentiating intermitotic cells of high mitotic frequency and the radioresistant tissues composed of reverting and fixed postmitotic cells. However, the endothelium of large blood vessels does not seem to be quite as responsive to radiation as that of smaller blood vessels. This difference in itself suggests either an enhanced conditional sensitivity of endothelium of small vessels or an influence of indirect mechanisms of alteration at the intertissue level, or perhaps even more remote indirect mechanisms contributing to the total radiation effect observed in the fine vasculature after moderate doses of radiation.
The reasons for this difference in radiosensitivity between the endothelium of small vessels and that of large vessels are not yet entirely clear. One of the possible reasons may be related to the fact that greater degrees of endothelial change are seen in the small vessels of irradiated organs containing radiosensitive parenchymal cells and showing greater degrees of inflammatory response involving the vessels (congestion, edema) than in the small vessels of irradiated organs containing radioresistant parenchymal cells and showing lesser degrees of such inflammatory response. This suggests that some of the observed radiation-induced changes in the endothelium of the fine vasculature may depend on the degree of such inflammatory response, which in turn depends on the degree of destruction of parenchymal cells, which in turn depends on the relative radiosensitivity of the parenchymal cells in the organs under consideration.
Another possible reason for the difference in observed degree of endothelial effect between large and small vessels may be related to differences in the size of the vascular lumens. The lumens of fine blood vessels are much more easily occluded by various effects of radiation on endothelium than those of large blood vessels, and the integrity of the walls of small blood vessels is thereby likely to be much more jeopardized by small degrees of change in the endothelial lining. The production of similar degrees of initial radiation effect on the endothelium of large vessels with their large lumens involves much less immediate pathologic consequence to the vessels themselves.
Blood sinusoids, which are lined not by typical endothelial cells but by phagocytes, are also less sensitive to radiation than are the small blood vessels lined by typical endothelium. The phagocytic cells are considerably more radioresistant than the endothelial cells, and the lumens of sinusoids are usually considerably larger than those of the fine vasculature line by endothelium.
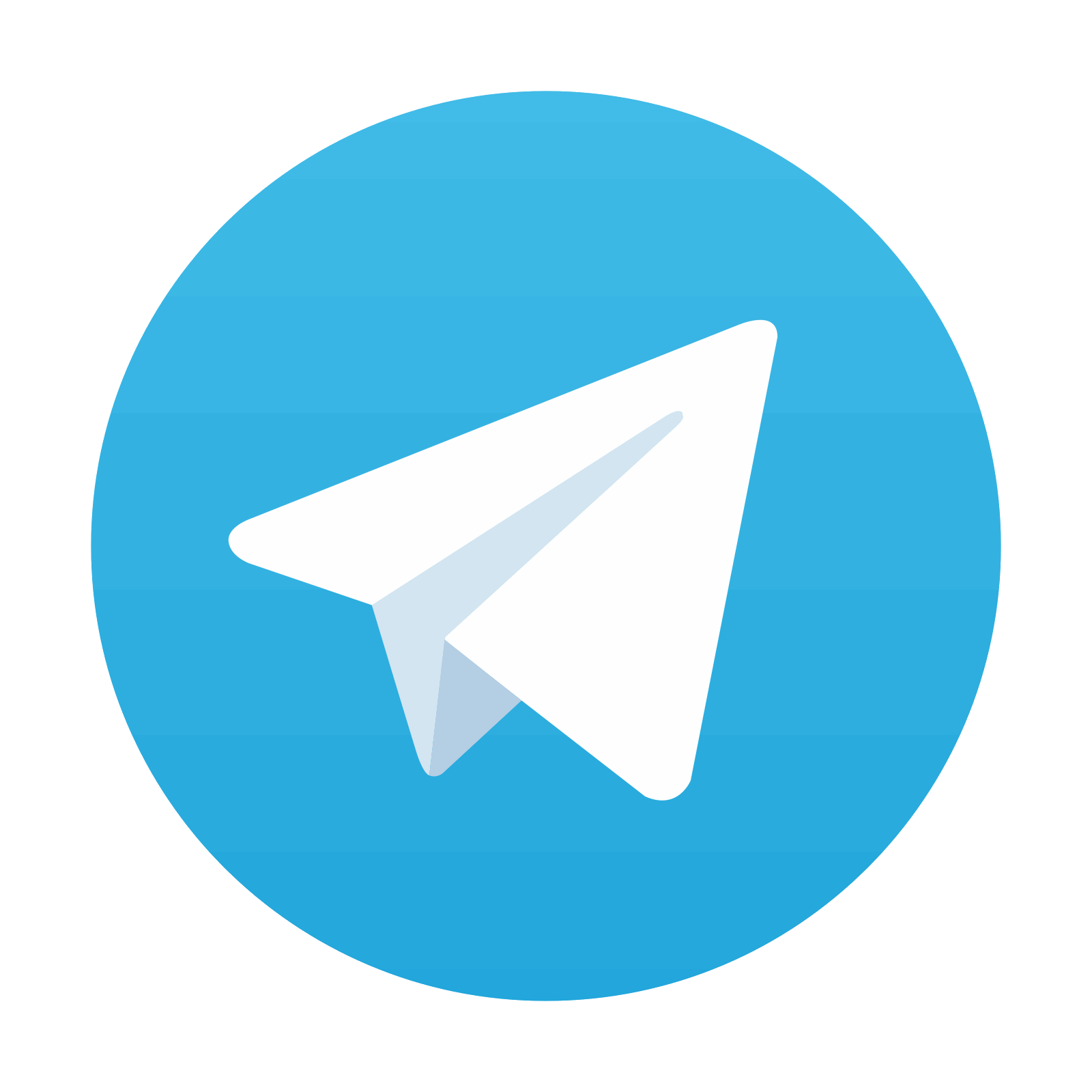
Stay updated, free articles. Join our Telegram channel

Full access? Get Clinical Tree
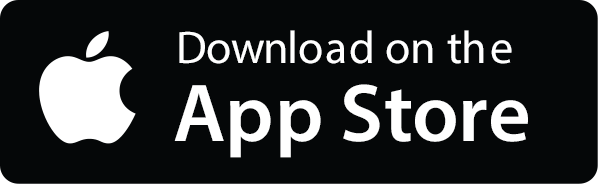
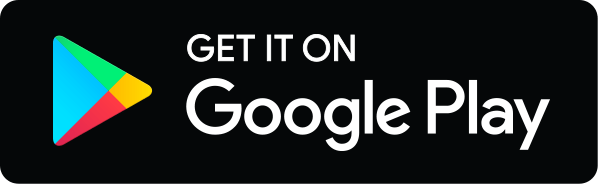