Best response
WHO change in the sum of products
RECIST change in sums longest diameters
CR (complete response)
Disappearance of all target lesions
Disappearance of all target lesions
PR (partial response)
50 % decrease
30 % decrease
SD (stable disease)
Small changes that do not meet above criteria
Small changes that do not meet above criteria
PD (progressive disease)
25 % increase
20 % increase
2.1 Response Evaluation Criteria in Solid Tumors (RECIST)
Measurable lesions are defined as lesions of 20 mm minimum size in nonspiral CT and 10 mm size in spiral CT data sets. These are attributed to target lesions (up to five per organ) which constitute total tumor burden in their linear summation. All nonmeasurable lesions are assigned to nontarget lesions and also documented. Bone metastases are regarded as nonmeasurable lesions in RECIST. Results are subsequently assigned to response-defined categories of complete response (CR), partial response (PR), stable disease (SD), and progressive disease (PD). PR is defined as more than 30 % decrease in the linear sum of target lesions (corresponding to an extrapolated 65 % decrease in volume, Fig. 2) and PD as more than 20 % increase (approximately 73 % volumetric increase). RECIST has become broadly accepted in clinical practice, by research groups and industry as an easy-to-execute, time-efficient, standardized procedure to assess tumor response to individual therapy (Park et al. 2003). RECIST place minimal added demand on clinical routine practice and at the same time provides comprehensible results and clearly defined target values.
However, there are some inherent methodological drawbacks, which have to be taken into account. First, neither linear or bidimensional measurements adequately address tumor heterogeneity and morphologic changes under therapy. Second, therapy response criteria based on morphology alone do not reflect metabolic changes within tumor tissue. Furthermore, reader-dependent inconsistencies in data interpretation may occur by the unattended use of rudimentary image-processing or measurement tools (e.g., the use of manually adjusted electronic calipers) with reported disagreements between readers ranging between 15 and 40 % (Belton et al. 2003). Also, measurement recommendations mainly assume sharply defined, high-contrast target lesions which are not necessarily routinely present. Therefore major variance can be expected in ill-defined, asymmetric, or diffuse tumor lesions. Finally, RECIST does not provide specific technical guidelines concerning examination settings in CT image acquisition (e.g., milli-ampere second/mAS, peak kilovolts/kVp) or pulse sequence-specific properties in MRI, which can have substantial influence on image quality and signal behavior.
2.2 Revised RECIST 1.1
RECIST has been used and validated since 2000 in multiple prospective trials. Based on this large experience with RECIST, these criteria have been revised (called RECIST 1.1) in 2009 (Eisenhauer et al. 2009). Mainly the assessment of the tumor response has been simplified by reducing the number of target lesions from ten to five and from five to two per organ, maximum. Further on, lymph nodes with size of their short axis above 15 mm are considered as pathological and assessable as target lesions. The short axis measurement should be included in the sum of lesions for response assessment. Shrinkage of pathologically lymph nodes below 10 mm is considered as CR in RECIST 1.1. The response as assessed with RECIST 1.1 has not to be confirmed in randomized trials any more. Also the definition of PD has changed. In addition to the previous criteria in RECIST, in particular a increase of <20 % in the sum of diameters, an absolute 5 mm increase is now required. Further on, “unequivocal progression” of nontarget lesions is in RECIST 1.1 defined more precisely: the increase in size of nontarget lesions has to be representative of overall disease and must not be based on a single lesion. In contrast to RECIST the revised RECIST 1.1 give detailed specifications for standard anatomical radiological imaging.
3 Multimodal Imaging for Evaluation of Therapy Response
The principal role of imaging procedures after RE one hand is to display metastatic burden of the liver to assess local response. Therefore, size, number, and localization of lesions need to be accurately displayed and discriminated from potential benign liver lesions. On the other hand it is important to reliably assess potential tumor progression through presence of extrahepatic tumor manifestations, such as lymph node, lung, bone, abdominal, or cerebral metastases. For this purpose a variety of imaging procedures are available, either based on a stepwise, multimodal imaging approach or, alternatively, as integrated, total body imaging techniques.
3.1 Ultrasound
Due to its broad availability and cost-effectiveness, ultrasound is regarded as a first-line imaging modality used in patients with known or suspected liver tumors. One of the major advantages of ultrasound is the “dynamic” nature of the technique allowing spontaneous analysis of suspected lesions in any spatial orientation and providing additional information through assessment of lesion mobility, echogenicity, and even elasticity. The B-mode gray scale display is highly useful for the differentiation of malignant lesions from common benign foci, such as benign cysts or haemangiomas. Reported sensitivities for the detection of malignant liver lesions range between 58 and 70 % for B-mode gray scale scanning and can potentially be further enhanced by technical innovations such as “tissue harmonic imaging” (THI), 3D-scanning and cross beam techniques (Bartolozzi et al. 1996; Harvey and Albrecht 2001). Especially, THI allows for enhanced display and lesion delineation by recording echoes with double frequency (Tanaka et al. 2000). Also, color-coded duplex and power Doppler imaging can provide additional information to further categorize focal liver lesions based on their perfusion characteristics. Furthermore, accompanying pathologies of liver vasculature (e.g., portal vein thrombosis, arterial stenosis) can be assessed (Reinhold et al. 1995). Yet, it has to be taken into account that an important limitation of ultrasound examinations is the examiner-dependent variability of imaging results, conditional to the experience, and skills of the operator. Furthermore, image quality may be impaired by several physical influences, such as present ascites or liver cirrhosis.
The introduction of contrast-enhanced ultrasound (CEUS) with the use of microbubbles with a diameter of 2–6 μm has significantly expanded the diagnostic potential of sonography (Fig. 1) (Quaia 2007). As a major advantage CEUS allows the real-time assessment of focal liver lesion perfusion during low transmit power insonation and it is not limited by motion and blooming artifacts encountered in color- and power Doppler ultrasound. Microbubbles are comprised of biocompatible materials, including proteins, lipids, and a filling gas, for example nitrogen (Levovist, Bayer Schering Pharma, Berlin, Germany). Microbubbles work by resonating in a US beam, rapidly contracting and expanding in resonance to the pressure changes of the sound wave. If the transmitted acoustic pressure is weak, microbubbles are vibrating symmetrically and there is a conventional linear response, but, if the transmitted acoustic pressure is strong, microbubbles are vibrating asymmetrically, and there is a harmonic nonlinear response. Further developments of micro bubble properties have led to high-molecular weight and low solubility gases with an increased vapor concentration inside the bubbles relative to surrounding tissue and increased stability in the peripheral circulation (Quaia 2007). The two approved agents of these second generation particles are Optison (GE Healthcare, Princeton, US) and SonoVue (Bracco Imaging, Milan, Italy). CEUS shows a high level of concordance with CT and MR imaging in depicting the contrast enhancement pattern of focal liver lesions during the arterial phase. Concordance in the portal venous phase is generally lower, reflecting the tendency of CT and MR contrast agents, unlike microbubbles, to diffuse into the tumoral interstitium in cases in which CEUS shows a washout (Burns and Wilson 2007). First trials have indicated an increase of liver lesion conspicuity by the use of contrast agents from 63 % for b-mode imaging alone to 91 % for combined B-mode/contrast-enhanced ultrasound (Albrecht et al. 2001). Especially, lesion characterization is ameliorated from 65 % to 92 % by the use of CEUS, as it has been reported in a different study (Reinhold et al. 1995). Yet, current data has shown, that detection rate is still restricted for liver lesions smaller than 2.5 cm and that lesions smaller than 1 cm cannot reliably be characterized (Rettenbacher et al. 2005). Furthermore, a major limitation of CEUS in comparison to multiphase CT- and MR imaging is the fact that only one liver tumor can be scanned at a time as the transducer has to be kept still during the examination and further micro bubble injections are often necessary to characterize additional liver tumors. Also, the technique requires specific training of the examiner and therefore at present is considered an important add-on rather than a substitute to established cross-section imaging. CEUS may especially provide an added diagnostic value in those incidental focal liver lesions in which contrast-enhanced CT or MR imaging are not conclusive, especially on single-phase scans or scans performed by an incorrect delay time.
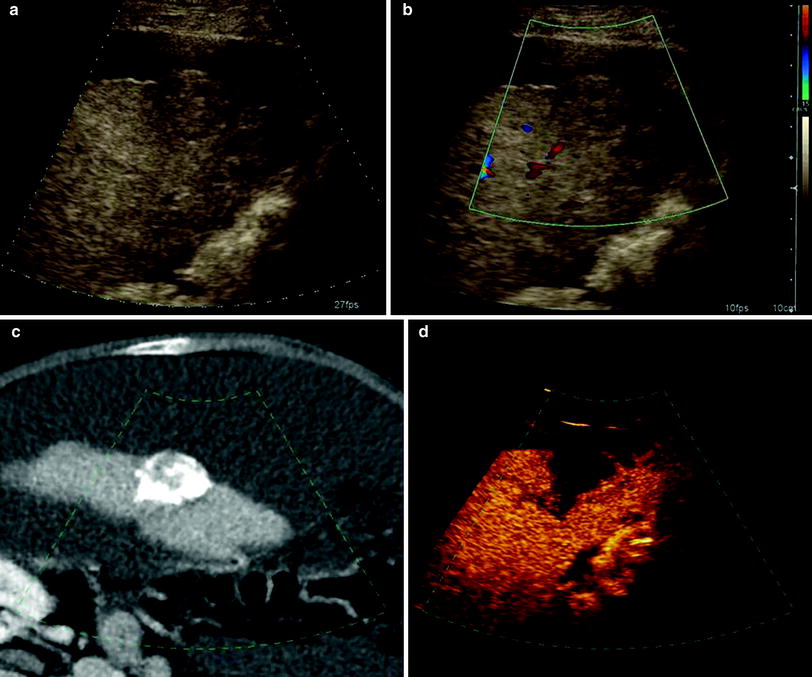
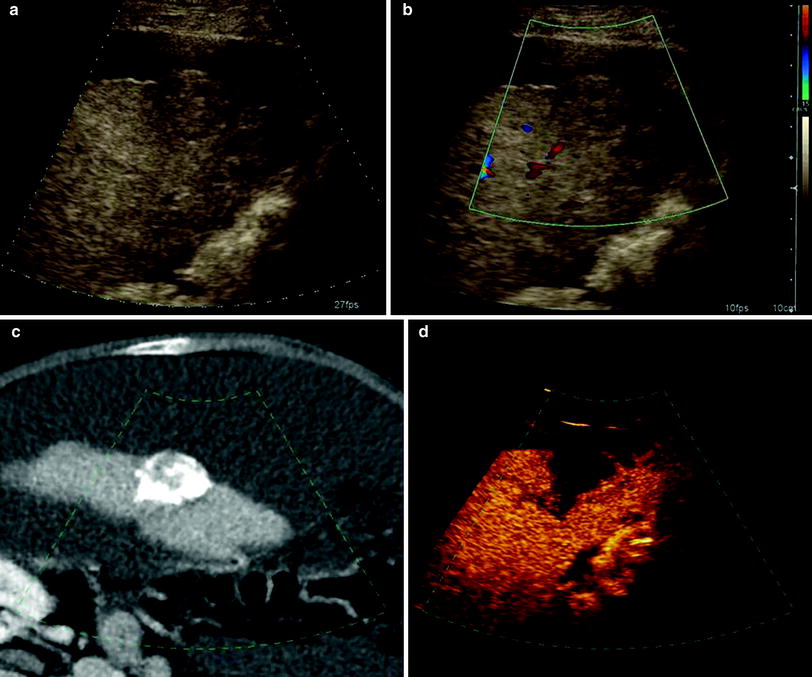
Fig. 1
Patient with focal liver metastasis under RE therapy. a, b Unenhanced ultrasound examination in B-mode and Doppler-mode shows a 3.5 cm large hypo- to isointense solid liver mass with capsular bulging consistent with metastasis. Postembolization CT shows accumulation of toxic agent within the target lesion. d Corresponding contrast-enhanced ultrasound indicates therapeutic success by showing hypovascularization of the embolized metastasis. (Courtesy of PD Dr. h.c. Dirk Clevert, Department of Radiology, LMU Munich)
3.2 Magnetic Resonance Imaging
MRI has been a useful modality in abdominal imaging for more than two decades and various technical improvements in sequence design and contrast media application have been made to enhance its diagnostic accuracy. Especially the development of fast gradient echo- or single-shot sequences, 3D acquisition techniques, and respiratory triggering have markedly ameliorated image quality and significantly decreased motion and respiratory artifacts. Furthermore, the development of parallel image acquisition acceleration techniques (PAT) as well as the introduction of high-field scanners has led to an additional gain in image quality (Zech et al. 2004). An inherent advantage of MRI over CT certainly is its high contrast of liver parenchyma and at the same time its ability to discriminate different tissue properties, such as fat, blood, water, and even cellular components such as hepatocytes, Kupffer cells or bile ducts based on its T1- and T2-weighted signal behavior and its reaction to tissue-specific contrast agents.
Extracellular gadolinium-based contrast agents still play a major role in liver MR imaging for the assessment of liver pathologies, especially for the detection and monitoring of malignant liver lesions. The reported sensitivities for lesion detection are ranging between 54 and 81 % (del Frate et al. 2002; Matsuo et al. 2001). In direct comparison, gadolinium-enhanced MRI has been reported superior to biphasic spiral CT regarding both lesion detection and characterization with a fundamental impact on patient management (Semelka et al. 2001). In the context of response assessment, dynamic contrast-enhanced multiphasic MRI enables to depict hypervascularity and alterations in tumor perfusion following radioembolization (Fig. 2). Yet, at this time there is no clear evidence that this modality is more effective or more accurate than conventional triphasic CT for this purpose (Ibrahim et al. 2009; Atassi et al. 2008).
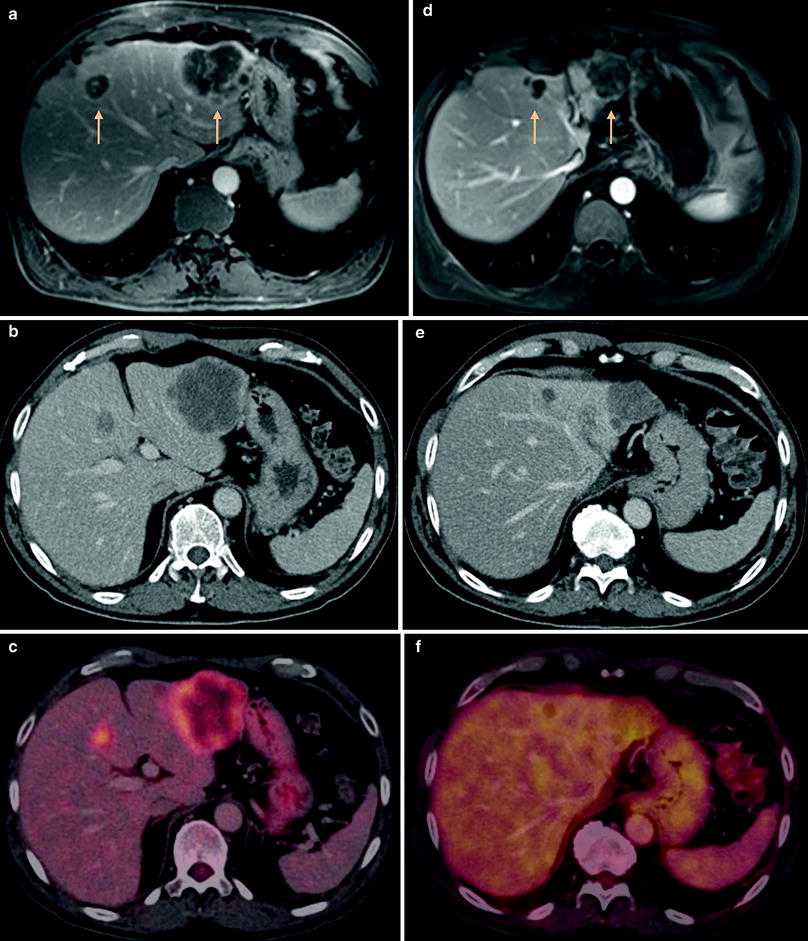
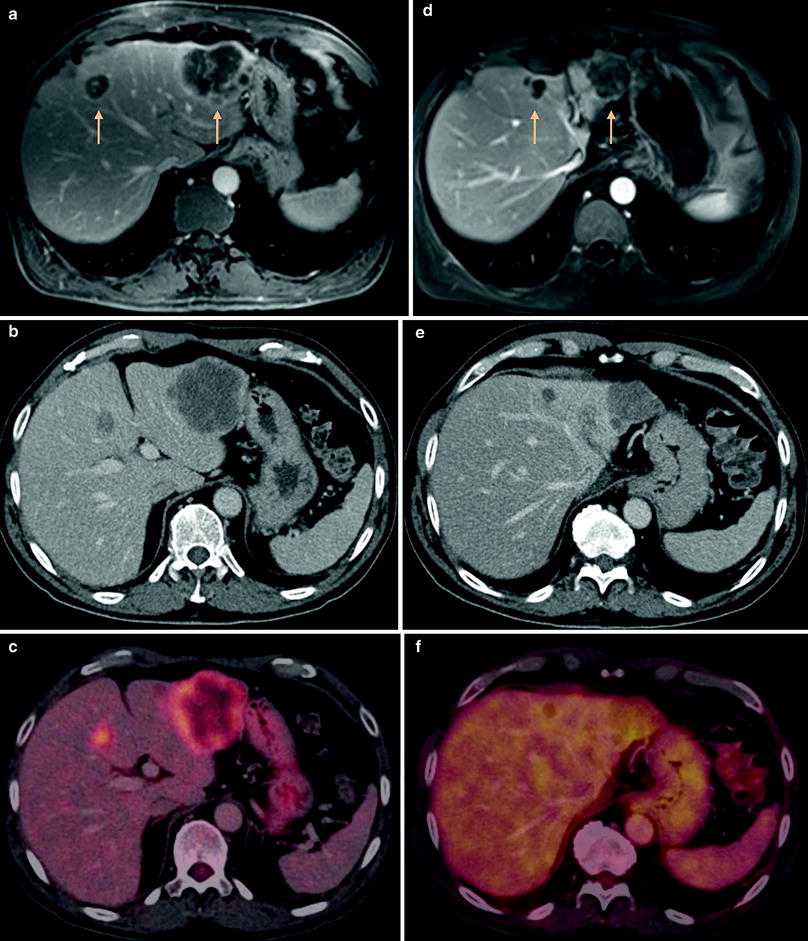
Fig. 2
Patient with cancer of the sigmoid under RE therapy. a Contrast-enhanced 3D-GRE-MRI shows multifocal liver metastases with a large tumor in liver segment 2 and another lesion in segment 4A. Both lesions show marked peripheral enhancement of contrast agent. b, c Corresponding FDG-PET-CT confirms the lesions with an increased peripheral tracer uptake (SUVmax 10,0). d Follow-up MRI 3 months after RE shows necrotic transformation of the lesions with significant reduction in size (>30 %, PR) and reduced contrast enhancement. e, f Response is confirmed by PET-CT showing no pathologic tracer uptake above physiological background tracer accumulation
Liver-specific contrast agents enable a significant increase in the detection rate of suspected liver metastases. These agents are divided into two basic groups: on one hand there are iron oxide particles (SPIO, superparamagnetic particles of iron oxide), targeted to the reticulo-endothelial system (especially Kupffer cells). On the other hand there are hepatobiliary contrast agents, directed to the hepatocytes and subsequently excreted with the bile.
The basic principle of SPIO is based on the fact that there are usually no Kupffer cells in malignant liver lesions. SPIO-based agents, like Resovist (Bayer Schering Pharma, Berlin, Germany), cause a homogeneous signal decrease in healthy liver parenchyma in T2/T2*-weighted sequences by inducing local inhomogeneities of the magnetic field, consequently leading to a positive, hyperintense contrast of the malignant focus. Although SPIO-based agents are not suitable to evaluate the perfusion and vascularity of focal liver lesions they nevertheless can contribute to lesion characterization. With SPIO-based contrast agents sensitivity ranges between 83 and 97 %, as reported in literature (del Frate et al. 2002; Lencioni et al. 1998). Several studies have confirmed the superior performance of SPIO-based liver MRI compared to biphasic spiral CT (Atassi et al. 2008; Reimer et al. 2000; Namkung et al. 2007).
Hepatobiliary contrast agents work through a direct uptake into the hepatocytes and subsequently cause a signal increase of normal liver parenchyma in T1-weighted sequences by shortening the T1-relaxation time. In contrast, malignant lesions appear hypointense against the hyperintense physiological background. Of this agent group, Gadolinium-based substances such as Primovist (Bayer Schering Pharma, Berlin, Germany) and Multihance (Bracco, Milan, Italy) have been developed. Their important advantage is that they can be administered as a bolus, and therefore both an early dynamic phase and a liver-specific phase can be acquired within one scan after one single injection, which represents a substantial advantage over SPIO’s. The sensitivity for the detection of liver metastases with hepatobiliary contrast agents ranges between 70 and 90 % (Bartolozzi et al. 2004; Bluemke et al. 2005). Especially, the combined analysis of early dynamic phase images and delayed phase images obtained from liver-specific agents yielded the highest detection rate (Kettritz et al. 1996). Furthermore, there are reports that the use of hepatobiliary contrast agents may also lead to a higher sensitivity for the depiction of liver metastases under current therapy, compared to contrast-enhanced CT (Beziat et al. 2004).
Findings of diffusion-weighted MRI (DWI) may potentially serve as an early biomarker, in analogy to information on metabolic changes derived in PET, and represents a promising technique for noninvasive assessment of tumor response after radioembolization (Deng et al. 2006). Studies with both transarterial chemoembolization (TACE) and Y90 radioembolization have shown that DW-MRI may represent a sensitive tool in the early follow-up period to assess therapy response. By evaluating neoplastic tissue water mobility, DW-MRI has been reported to detect tumor response within 42 days of radioembolization, being able to differentiate neoplastic tissue from reactive edema (Atassi et al. 2008; Reimer et al. 2000). Another recent study has described a reproducible, significant decrease of ADC values in responding liver lesions after RE in as early as 2 days following treatment in comparison to nonresponders (Dudeck et al. 2010). Early detection of such alterations in tumor microstructure therefore suggests a sentinel role for DW-MRI in selected patients (Kalva et al. 2008).
3.3 Multislice Computed Tomography
CT has developed dramatically in the last decade. Scanners with 64 or more rows enable high spatial and temporal resolution imaging, which allows the integration of bi or triphasic examinations of the liver with a thoracic scan. The short acquisition time of multidetector CT scanners in combination with their reliable high resolution allowing whole-body assessment make them the backbone of oncologic therapy assessment. Of course the imaging technique has to be adapted to the underlying tumor entity, but in most cases a late-arterial and a portal venous phase abdominal CT can be regarded as standard. In selected cases such as hepatocellular carcinomas, hepatic metastases of neuroendocrine tumors or the mapping of the arterial vessels for planning of transarterial therapies an early arterial phase can be included. Therapy monitoring based on CT has shown to be predictive for survival in various tumors and treatments (Eisenhauer et al. 2009).
Several studies have proven its predictive value in patients treated with 90Y-microspheres as well. Jakobs et al. have analyzed the response rates and survival in 41 patients with colorectal cancer liver metastases treated with radioembolization. Their results indicate that therapy response based on CT is correlated with a significantly improved survival of patients (Jakobs et al. 2008 b). The same seems to be true for patients with breast cancer liver metastases. Women with a PR had a survival of 23.6 months as compared to 5.7 months in women not responding to the treatment (Jakobs et al. 2008 b). Also in neuroendocrine tumor liver metastases response as assessed on CT scanning is able to predict survival of patients after radioembolization (Saxena et al. 2010). However, some pitfalls for response assessment have to be considered. After radioembolization hepatic perfusion is often inhomogeneous making exact tumor delineation sometimes difficult. Further on, in primary liver tumors such as cholangio-cellular carcinomas and in particular in hepatocellular carcinomas response assessment solely based on size may not accurately reflect the prognosis of the patients after locoregional treatment. There is evidence that in these cases response assessment based on enhancement modes such as the EASL criteria more accurately helps to predict long-term survival (Shim et al. 2012).
4 Whole-Body Imaging
Whole-body PET using [18F]-fluoro-2-deoxy-d-glucose (FDG) is an imaging modality that can detect cancerous disease by tracing increased FDG uptake in tumor lesions. The introduction of combined PET-CT scanners has made a new modality available for whole-body imaging which combines the functional data of PET with the anatomical information of CT scanners in a single examination. Various study results indicate, that a fusion of both modalities improves diagnostic accuracy as well as lesion localization and report promising results for the staging of various oncologic diseases compared to PET and CT alone (Pelosi et al. 2004; Cohade et al. 2003; Lardinois et al. 2003).
MRI with its lack of ionizing radiation, high soft tissue contrast, and spatial resolution is a useful application for tumor detection and staging of malignancies. In recent years, whole-body MRI (WB-MRI) has fundamentally changed diagnostic concepts for oncologic imaging as an alternative to standard multimodality imaging strategies and is now increasingly applied in clinical routine for integrated imaging of various neoplasms. However, the crucial problem for implementing WB-MRI in the past has been to integrate substantially different requirements in hardware setup, contrast media application, slice positioning, and sequence design into one single comprehensive scan. Significant improvements in hardware, from pioneering approaches using a rolling platform system mounted on top of a conventional MRI scanner to the essential introduction of multireceiver channel scanners with automated free table movement, have cleared the way for clinically feasible and efficient total body imaging concepts (Barkhausen et al. 2001; Schmidt et al. 2005). Furthermore, important innovations in sequence design and image acquisition, such as parallel acquisition techniques (PAT), have helped to significantly reduce overall examination times without compromising spatial resolution and have increased patient comfort and acceptance. Now, a dedicated assessment of various organ systems by sequences with adequate soft tissue contrast, image orientation, spatial resolution, and contrast media dynamics can be combined with whole-body anatomic coverage.
Especially in the field of oncologic imaging various useful applications have emerged for an integrated diagnostic approach to cancer as a systemic disease, indicating its use as a radiation-free alternative to competing modalities such as multislice-CT (MS-CT) or FDG-PET-CT (Clark et al. 2005; Schlemmer et al. 2005).
4.1 PET and PET-CT
PET using 18F-fluoro-2-deoxy-glucose (FDG) enables imaging of tumor metabolism. FDG as a glucose analogue is taken up via the glucose transporters located on the cell surface. As the glucose consumption is increased in most cancer cells, FDG uptake is increased as well. After phosphorylation FDG does not undergo further metabolism and cannot be transported outside the cell again, and therefore is trapped inside.
PET/CT using FDG enables the combination of anatomic and functional information and thereby overcomes the limitations of the separate imaging modalities. The technical developments in the recent years have shortened the examination time dramatically. With PET/CT scanners of the newest generation the examination time of a typical whole-body scan is as short as 15 min. Mainly due to this shortening of the scan time, PET/CT scanning can be offered the majority of oncologic patients.
The current standard of monitoring tumor response is measuring tumor shrinkage on CT. Despite several revisions and refinements in recent years CT-based response assessment still has fundamental limitations. Because of difficulties in delineating tumor tissue from secondary changes in the surrounding tissue the inter-observer variability in tumor size measurements is still high (Erasmus et al. 2003). Further on, anatomic imaging has drawbacks in differentiating residual viable tumor tissue from treatment-induced scarring. This may even be more important in locoregional therapies of liver tumors, because these tumors often show an increasing necrosis without any significant change of size (Miller et al. 2007). In many studies the potential of metabolic imaging in the assessment of therapy response has been shown. Consistently, these studies proved the higher accuracy of FDG PET to differentiate viable tumor from treatment-induced necrosis. Further on, a decline in the metabolic activity of the tumor usually precedes the decline in size indicating therapy response, offering the opportunity of early prediction of patient outcome (Wahl et al. 2009). It has also been shown for different cancer entities that FDG PET response is predictive for survival after therapy (Weber 2009). Therefore, FDG PET/CT seems to be well suited for therapy monitoring of patients after radioembolization. Consequently, more patients show response to hepatic radioembolization on FDG PET as on CT. In a study including 44 women with hepatic metastases from breast cancer response after radioembolization was compared on CT and FDG PET. While on CT 47 % of the women showed a PR, FDG PET indicated therapy response in 95 % (Coldwell et al. 2007). Similar results have been presented in hepatic metastases from colorectal cancer. Up to 95 % of patients had a response on FDG PET (Gulec et al. 2012). Also, the course of the tumor marker CEA is correlation significantly with the metabolic response, but not with the anatomical based response in these patients, indication superiority of FDG PET to CT (Wong et al. 2002). However, none of these studies showed the prognostic value of the metabolic response for the survival of the patients.
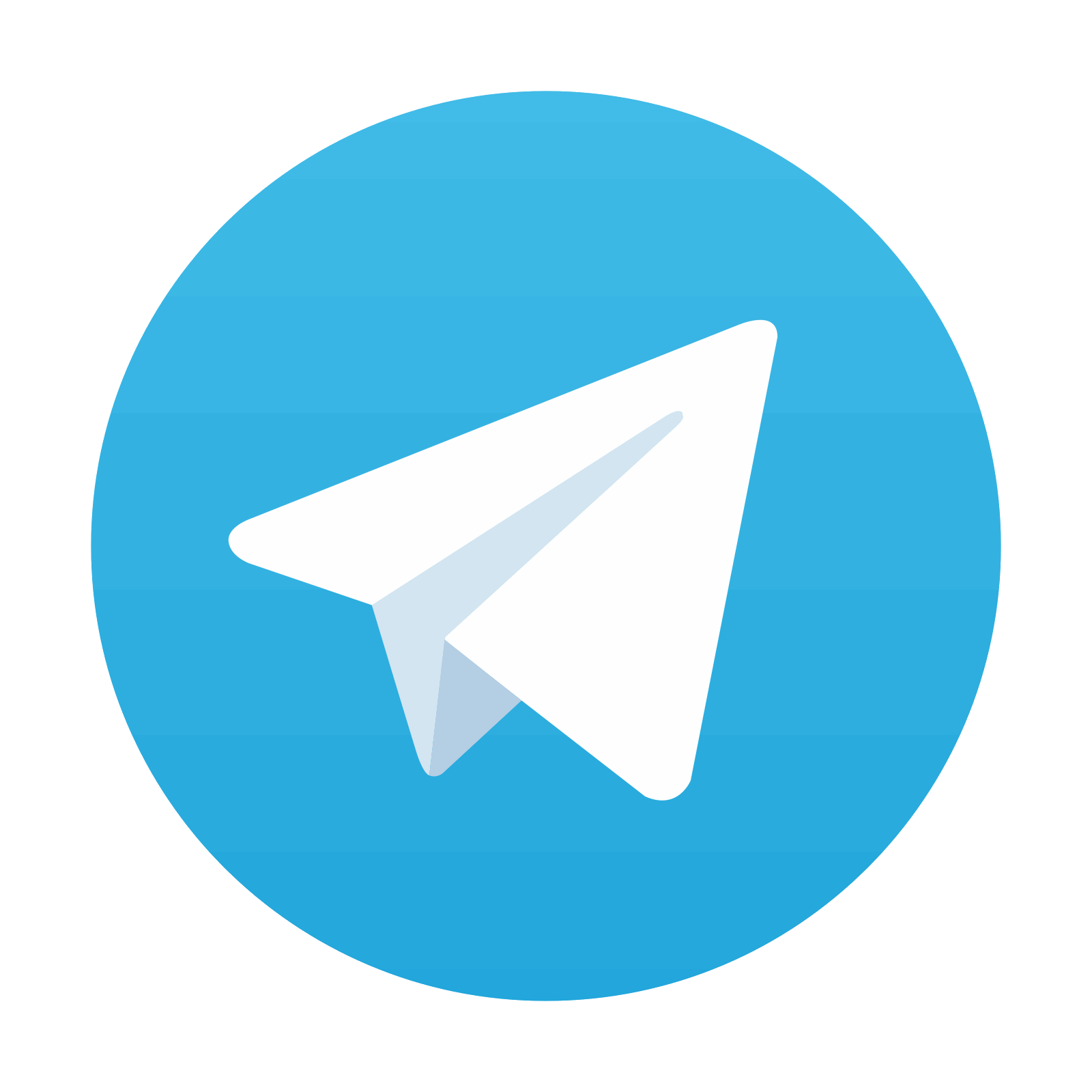
Stay updated, free articles. Join our Telegram channel

Full access? Get Clinical Tree
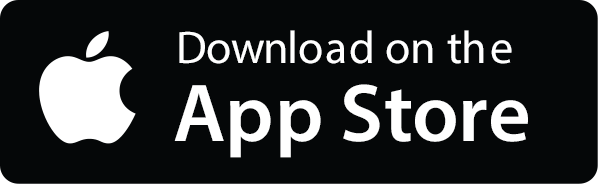
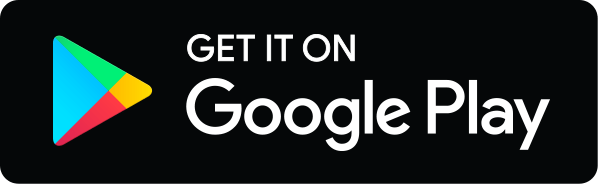