Fig. 2.1
Diagram representing fluorescence. The red arrow represents the absorption of light. The blue arrow represents vibrational relaxation from singlet excited state, S2 to S1. This process is a non-radiative relaxation in which the excitation energy is dispersed as vibrations or heat to the solvent, and no photon is emitted. The green arrow represents fluorescence to the singlet ground state, So
Application of Fluorescence in Medicine
By understanding what fluorescence consists on, we can now think on the uses that can be given in medicine. Fluorescence in the life sciences is used generally as a non-destructive way of tracking or analysis of biological molecules by means of the fluorescent emission at a specific frequency where there is no background from the excitation light. As relatively few cellular components are naturally fluorescent (autofluorescence), in fact, a protein or other component can be “labeled” with an extrinsic fluorophore, a fluorescent dye that can be a small molecule, protein, or quantum dot. These are the bases for most of the applications of fluorescence in medicine. The quantification of a dye is done with a spectrofluorometer and finds additional applications.
Some of the uses of fluorescence in medicine include:
Microscopy: when scanning the fluorescent intensity across a plane one has fluorescence microscopy of tissues, cells, or subcellular structures, which is accomplished by labeling an antibody with a fluorophore and allowing the antibody to find its target antigen within the sample. Labeling multiple antibodies with different fluorophores allows visualization of multiple targets within a single image. DNA microarrays are a variant of this.
Immunology: an antibody is first prepared by having a fluorescent chemical group attached, and the sites (e.g., on a microscopic specimen) where the antibody has bound can be seen, and even quantified, by the fluorescence.
Automated sequencing of DNA by the chain termination method; each of four different chain terminating bases has its own specific fluorescent tag. As the labeled DNA molecules are separated, the fluorescent label is excited by a UV source, and the identity of the base terminating the molecule is identified by the wavelength of the emitted light.
DNA detection: the compound ethidium bromide, in aqueous solution, has very little fluorescence, as it is quenched by water. Ethidium bromide’s fluorescence is greatly enhanced after it binds to DNA, so this compound is very useful in visualizing the location of DNA fragments in agarose gel electrophoresis. Intercalated ethidium is in a hydrophobic environment when it is between the base pairs of the DNA, protected from quenching by water, which is excluded from the local environment of the intercalated ethidium.
Fluorescence image-guided surgery (FIGS) is a medical imaging technique that uses fluorescence to detect properly labeled structures during surgery. Its purpose is to guide the surgical procedure and provide the surgeon of real-time visualization of the operating field. When compared to other medical imaging modalities, FIGS is cheaper and superior in terms of resolution and number of molecules detectable. As a drawback, penetration depth is usually very poor (100 μm) in the visible wavelengths, but it can reach up to 1–2 cm when excitation wavelengths in the near infrared are used.
FIGS is performed using imaging devices with the purpose of providing real-time simultaneous information from color reflectance images (bright field) and fluorescence emission. One or more light sources are used to excite and illuminate the sample. Light is collected using optical filters that match the emission spectrum of the fluorophore. Imaging lenses and digital cameras are used to produce the final image.
Fluorescence excitation is accomplished using various kinds of light sources. Halogen lamps have the advantage of delivering high power for a relatively low cost. Using different band-pass filters, the same source can be used to produce several excitation channels from the UV to the near infrared.
During open surgery, handheld devices are usually preferred for their easy of use and mobility. A stand or arm can be used to maintain the system on top of the operating field. The disadvantage of these devices is that other lights in the operating room interfere in the fluorescence emission, which is simply solved by switching off the other lights. FIGS can also be performed using minimally invasive devices such as laparoscopes or endoscopes. In this case, a system of filters, lenses and cameras is attached to the end of the probe. Unlike open surgery, the background from external light sources is reduced.
The major limitation in FIGS is the availability of clinically approved fluorescent dyes. Indocyanine Green has been widely used as a non-specific agent to detect sentinel lymph nodes during surgery. Methylene Blue can also be used for the same purpose. First clinical applications using tumor-specific agents that detect deposits of ovarian cancer during surgery have been carried out.
History of Fluorescence
Bernardino de Sahagún and Nicolás Monardes described an early observation of fluorescence in the infusion known as lignum nephriticum (Latin for “kidney wood”) in 1560 and 1565 respectively. It was derived from the wood of two tree species, Pterocarpus indicus and Eysenhardtia polystachya. The chemical compound responsible for this fluorescence is matlaline, which is the oxidation product of one of the flavonoids found in this wood.
In 1819, Edward D. Clarke, an English mineralogist and traveler who amassed valuable collections of minerals; and in 1822 René Just Haüy, a French mineralogist and one of the founders of the science of crystallography, described fluorescence in fluorites. Sir David Brewster described the phenomenon for chlorophyll in 1833 and Sir John Herschel did the same for quinine in 1845.
George Gabriel Stokes was a British physicist and mathematician noted for his studies of the behavior of viscous fluids, particularly for his law of viscosity, which describes the motion of a solid sphere in a fluid, and for Stokes’s theorem, a basic theorem of vector analysis. In his 1852 paper on the “Refrangibility” (wavelength change) of light, he described the ability of fluorspar and uranium glass to change invisible light beyond the violet end of the visible spectrum into blue light. He named this phenomenon “fluorescence.” The name was derived from the mineral fluorite (calcium difluoride), some examples of which contain traces of divalent europium, which serves as the fluorescent activator to emit blue light. In a key experiment he used a prism to isolate ultraviolet radiation from sunlight and observed blue light emitted by an ethanol solution of quinine exposed by it.
First Approaches of Fluorescence in Medicine
The first approaches of fluorescence remote to 1953, Lay and Randall, used a fluorescent staining for detection of cancer cells in vaginal smears. They used the affinity of the basic fluorochrome dyes (berberine sulfate, acid fuchsin, and acridine) for acid nucleic acids. Because neoplasic cells have shown an increase in the total nucleic acids and ribonucleic acids, showing an increase in protein synthesis, the cancerous cells were evidently seen. They used a fluorescent microscope to evidence this finding [1, 2].
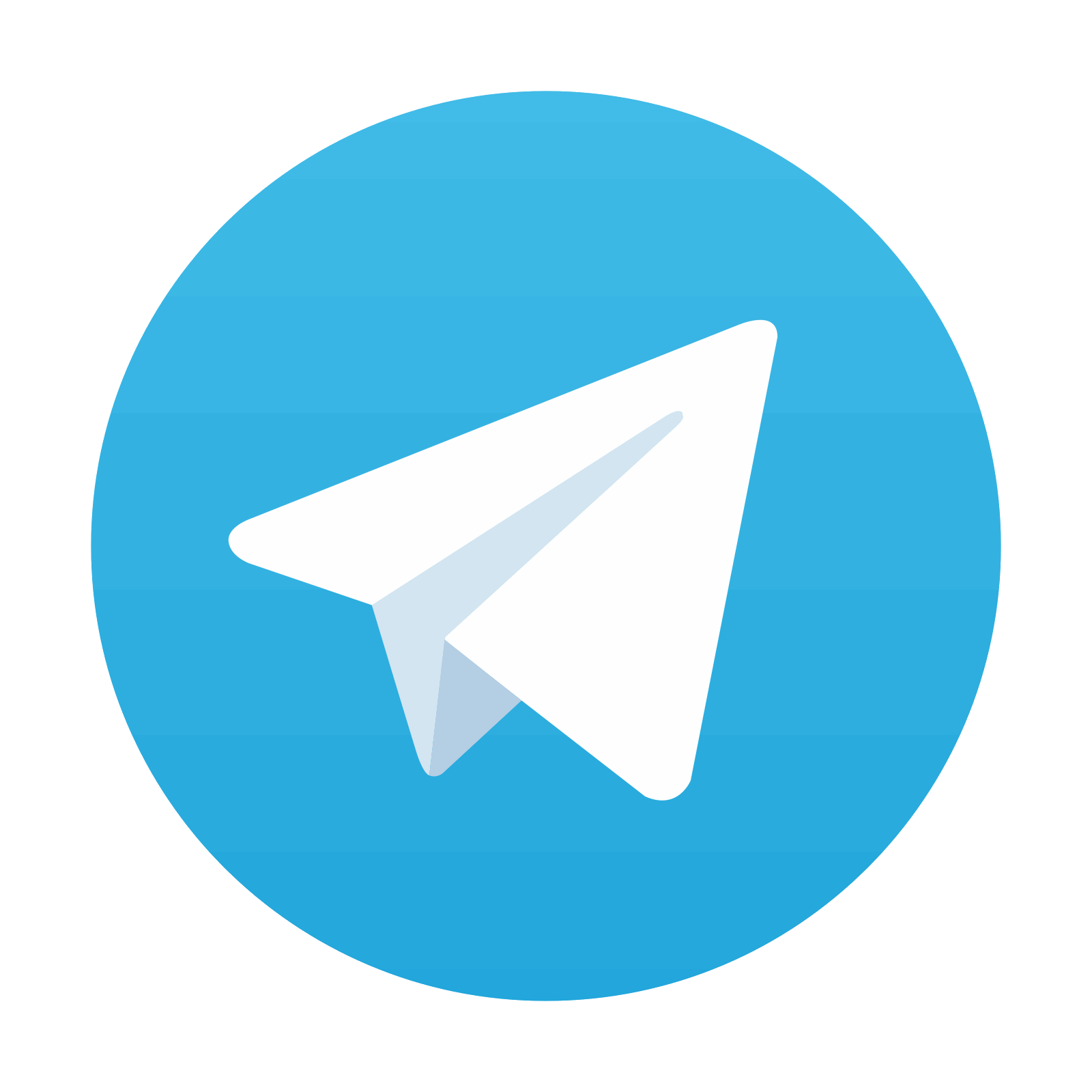
Stay updated, free articles. Join our Telegram channel

Full access? Get Clinical Tree
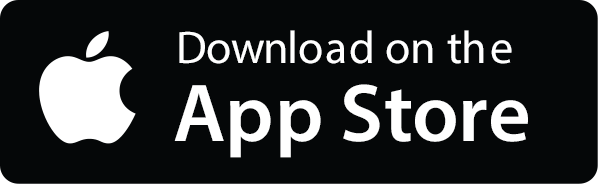
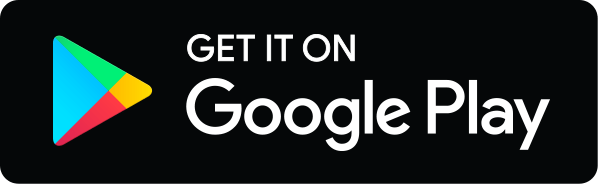