Introduction
Neuroradiological imaging of the pediatric patient has undergone tremendous change since the late 2000s. Although the use of magnetic resonance imaging (MRI) is on the rise, in part because of concerns over exposing children to medical radiation, there is still an important role for pediatric head computed tomography (CT), which provides fast imaging, often without the need for sedation. CT therefore remains an important tool for evaluating patients in the emergency department, especially in cases of acute trauma and critically ill inpatients who may be too unstable to undergo a lengthier MRI examination ( ). MRI also carries the additional risk of sedation and anesthesia for young children who have limited or no verbal skills and have difficulty remaining still in the magnetic resonance (MR) scanner long enough for the technologist to produce diagnostically useful images. At our institution, CT can be performed portably, making it an accessible test for children who cannot be transported to the radiology department. In addition, for patients who cannot undergo MRI secondary to implanted devices or external monitoring hardware, CT serves as the only available cross-sectional imaging modality.
The aim of this chapter is to provide an approach for interpreting pediatric head CT scans with a focus on recognizing intracranial structures in the developing brain while highlighting common disease entities seen in children. We will not, however, describe all potential neurological diseases of childhood that may be detected on head CT. As with the other chapters in this book, our goal is to present key findings that will enable the busy clinician to arrive at an appropriate or probable differential diagnosis by using a systematic approach to interpreting head CTs that will aid in clinical decision making.
Computed Tomography Techniques and Radiation Awareness
As CT use continues to grow among all age groups, the risks associated with exposure to ionizing radiation must be disclosed, as well as the fact that children experience disproportionate sensitivity to the effects of radiation. CT now accounts for the largest contribution to medical radiation dose in the United States. Children are at a greater risk for both short- and long-term effects of radiation, in part because their smaller bodies and organs are more susceptible to damage from higher doses and in part because their developing tissues and rapidly dividing cells are more radiosensitive than those of adults. These factors, combined with longer life expectancy, place children at higher risk for eventually developing cancers as a result of exposure to ionizing radiation, especially when CT must be repeated over months or years.
As a medical community, we can all play a role in minimizing radiation exposure through a variety of techniques. For example, shielding tissues outside of the imaging field can reduce the dose delivered to adjacent organs. Proper protocols should be selected for pediatric patients that take into account smaller body size with adjustments in the milliampere (mA) and peak kilovoltage (kVp). Protocols can also be further tailored depending on the clinical indication. Often a lower-dose scan can yield sufficient diagnostic information to answer a specific clinical question. At our institution, we select different scanning parameters for patients who are being followed for hydrocephalus where visualization of fine anatomic detail at the expense of a higher radiation dose does not add value to the clinical decision-making process ( ; ; ). Single-phase contrast examinations should be part of the routine standard of care because additional information is rarely captured from the noncontrast or delayed phases to justify the added radiation dose. The scanning parameters for children must also be adjusted to image only the region of interest, with care taken to minimize imaging of any collateral tissues or organs.
With the promotion of the Image Gently campaign, numerous resources are now available to help guide the selection of appropriate CT parameters for clinical practice ( ). At our institution, CT imaging protocols are continually reviewed by a team of radiologists, medical physicists, and radiology technologists to ensure scans are being optimized to achieve images of the highest diagnostic quality at the lowest possible levels of radiation exposure ( ). The parameters selected for our head CT protocol are presented in Table 25.1 . Modern multidetector scanners enable the acquisition of submillimeter (mm) thin-section images, which can then be reformatted into multiple viewing planes, but with no additional radiation dose delivered to the patient. Three-dimensional (3D) rotating models can also be generated from the axial data. These 3D models can both aid the radiologist in detecting pathology and provide surgeons and clinicians with a helpful visual aid for future disease management.
AGE | Display Field of View (DFOV) (cm) | KILOVOLTAGE (kVp) | MILLIAMPERE (mA) |
---|---|---|---|
Newborn to 6 months | 20 | 120 | 155 |
7 months to 2 years | 25 | 120 | 155 |
3 years to adult | 25 | 120 | 215 |
|
Special Computed Tomography Examination Considerations
As in adults, CT angiography (CTA) is also used for specific indications in the work-up of the pediatric patient. CTA is used to evaluate children with acute, nontraumatic parenchymal or subarachnoid hemorrhage; to evaluate for intracranial aneurysms, vascular malformations, or intracranial arterial dissections; or when concern exists for acute stroke where intravascular thrombolysis is being considered. At our institution, a single-phase, arterial-phase CT is performed that omits the noncontrast phase of the examination often performed in adult patients. CT venography is performed at some institutions for the evaluation of venous sinus thrombosis when MRI is either unavailable or contraindicated ( ).
Occasionally contrast-enhanced CT may also be performed for the work-up of a mass, for assessing metastatic spread of disease, or for evaluating infectious processes. In pediatric patients with headache in the setting of sinusitis or mastoiditis, contrast-enhanced CT may be used to evaluate for intracranial complications. In most instances, MRI is preferred for these indications; however, as mentioned previously, in patients for whom MRI is not indicated, contrast-enhanced CT can play a vital role in reaching a diagnosis. Under these circumstances, the noncontrast portion of the examination is generally omitted because postprocessing techniques can evaluate cases where there is a question of enhancement in the presence of hemorrhage or mineralization.
Key Imaging Questions
In order to systematically approach pediatric head CTs we have organized this chapter around key imaging questions and finding encountered in clinical practice.
Are the Vascular Structures Hyperdense?
Sinovenous Thrombosis
The causes of cerebral sinovenous thrombosis differ significantly between children and adults. In children and young adults the causes include conditions that result in narrowing of the sinuses and invasion of the sinus walls and may predispose to stasis and coagulation. Trauma with skull fractures extending to the venous sinuses can cause occlusion or compression of the sinus, thereby altering flow and predisposing to thrombosis. Neoplastic and infectious processes may directly invade the sinus walls resulting in sinus occlusion secondary to the infiltrate and associated narrowing. In the pediatric population a prothrombotic state may be caused by inherited disorders of coagulation, dehydration, certain medications, and various systemic diseases, including malignancies, anemias, chronic renal disease, and autoimmune disorders, such as systemic lupus erythematous. In the neonate, additional maternal and obstetric factors predispose to sinovenous thrombosis, including maternal infection and diabetes, traumatic delivery, and placental abnormalities including abruption ( ).
Familiarity with the cerebral venous anatomy is necessary to evaluate for cerebral sinovenous thrombosis. Cortical veins are superficially located along the brain surface and drain centrally to the dural venous sinuses. Deep cerebral veins, including the internal cerebral, medullary, and subependymal veins, drain blood from the deep brain structures to the vein of Galen, which, in turn, joins with the inferior sagittal sinus and together drain to the straight sinus. The major dural venous sinuses receiving blood from these superficial and deep systems include the torcula, sigmoid sinuses, transverse sinuses, and superior sagittal sinuses. The cavernous sinuses are also part of the deep venous system receiving blood from the ophthalmic veins and sphenoparietal sinuses. Each of these structures must be evaluated for abnormalities on CT. Thrombosis within any of these structures is collectively referred to as cerebral venous thrombosis.
Findings suggestive of sinovenous thrombosis on noncontrast head CT include increased attenuation and distension of the sinuses with possible extension of the hyperdensity into adjacent cortical veins known as the cord sign ( Fig. 25.1A–C ). Direct detection of the thrombus is seen in only approximately 20% of patients, however, so looking for secondary signs is important ( ). In the presence of adjacent infection such as otomastoiditis or orbital cellulitis, there is also the possibility of spread to the adjacent venous structures. With fractures, it is important to evaluate for extension of the fracture to the sinus, as well as for osseous displacement into the sinus with resultant compression and narrowing. Thrombosis can result in areas of parenchymal infarct and hemorrhage. Although infarcts typically present with loss of gray/white differentiation and edema, they do not characteristically correspond to a typical arterial distribution but may be associated with hemorrhage and proximal to the involved sinus or vein ( Fig. 25.1B–D ). When the thrombus involves the deep venous system, hyperdensity within the internal cerebral veins is usually seen. It is also important to look for subtle changes in low density in both thalami, specifically with blurring of the margins between the thalami and the internal capsule occurring secondary to venous congestion and edema.
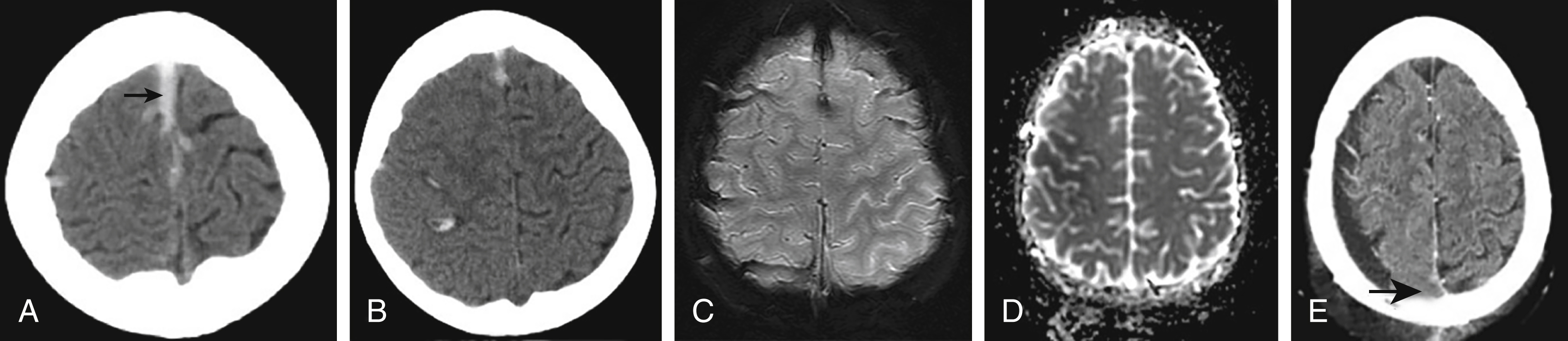
Identifying sinovenous thrombosis is particularly challenging in the neonate where hemoconcentration results in diffuse, increased attenuation in the sinuses on unenhanced head CT, especially in contrast with the low-density, unmyelinated brain ( ). In hemoconcentration, all of the dural venous sinuses will have a homogeneously hyperdense appearance. Although secondary parenchymal signs or complications of thrombosis may suggest underlying hemoconcentration, it is also important to determine whether the sinuses just appear relatively hyperdense secondary to the presence of diffuse cerebral edema. An additional challenge in identifying hemoconcentration in the newborn is the presence of birth-related, extraaxial hemorrhage that is often present posteriorly and adjacent to the sinuses. Parturitional subdural hemorrhage is a very common finding in both vaginal and cesarean deliveries and is seen with increased frequency in vacuum- or forceps-assisted deliveries. Up to 46% of neonates have small, parturitional subdural hemorrhages, which can be both supratentorial and infratentorial in location, and may occur with concurrent subgaleal and cephalohematomas within the scalp. Establishing the location of hyperdensity is therefore critical because birth-related hemorrhages are most often subdural, and thus will be located deep to the dural venous sinuses and separated by the overlying dura. These small hemorrhages typically track along the subdural space at a distance from the dural sinuses, offering an additional clue as to their location.
Overall, noncontrast CT is insensitive for the diagnosis of sinovenous thrombosis, with positive results in only roughly 30% of cases, even when considering all of the findings. If there is high clinical suspicion, however, or if noncontrast CT shows any of the suggestive findings described earlier, further imaging with a contrast-enhanced CT venogram or MRI venogram is recommended. On contrast CT venography, the empty delta sign, which represents the nonenhancing thrombus surrounded by the enhancing dura, is generally noted ( Fig. 25.1E ) ( ).
Acute Arterial Stroke
Although pediatric arterial stroke occurs only rarely in children, with an incidence of approximately 1.6 per 100,000 per year, it still represents a major public health burden because of the long-term morbidity associated with significant neurological deficits that typically result from this event. The incidence of arterial stroke is highest in children younger than 1 year. Unfortunately, the diagnosis is often delayed secondary to the absence of risk factors typically associated with adult stroke (e.g., clinical history of heart disease, hypertension, obesity, nicotine use) and variability of clinical presentation. The primary causes for stroke in children include cardiac shunts, sickle cell disease, and inherited coagulation disorders, which account for most of the underlying causes. In children with multiple strokes, occlusive vasculopathy including moyamoya disease, radiation therapy, trisomy 21, and neurofibromatosis type 1 should be considered as possible causes ( ).
The dense middle cerebral artery (MCA) sign refers to the hyperattenuating appearance of the M1 segment of the MCA secondary to thrombosis of the vessel. It is an important early indicator of ischemic stroke on unenhanced CT. Although this sign is very specific, it is insensitive and present in only 30% of patients with a proximal MCA occlusion ( ). Caution should be exercised when subjectively assessing the density of the MCA, however, because apparent, increased vessel attenuation can also be seen in cases of elevated hematocrit such as in polycythemia and in herpes encephalitis secondary to edema in the subjacent brain parenchyma. An absolute attenuation cutoff of 43 Hounsfield units and a ratio of the attenuation of the contralateral MCA above 1.2 have been shown to reliably exclude false-negative cases ( ; ). The hyperdense vessel sign can also be applied to the other intracranial vessels, including the carotid and basilar arteries ( Fig. 25.2 ), although this is seen less commonly than in the MCA.
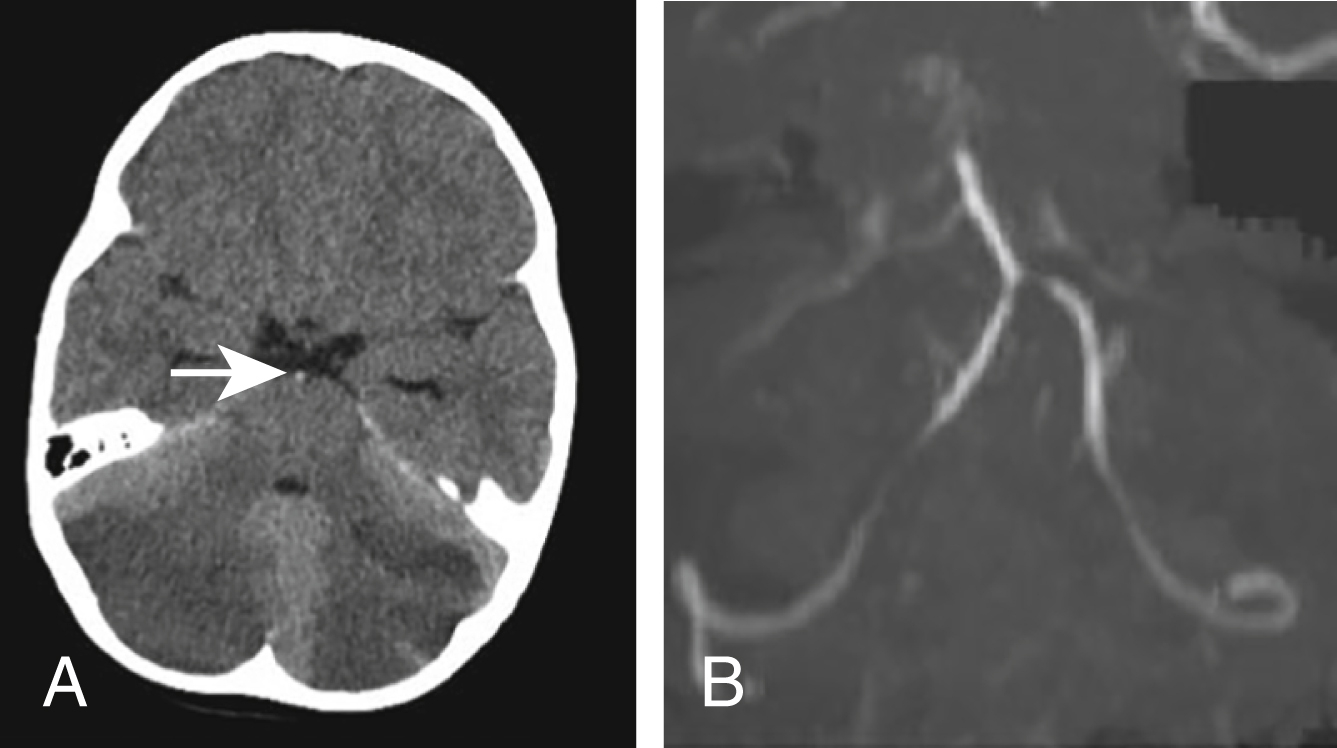
To achieve optimal therapeutic management, children with acute ischemic stroke must be identified as quickly as possible because thrombolysis and antithrombotic therapy are increasingly being used at pediatric stroke centers and are key to recovery and rehabilitation ( ). In addition to the hyperdense MCA sign, other signs seen in the setting of early acute MCA territory stroke include edema, loss of gray/white differentiation, and effacement of the sulci within the region supplied by the MCA, including the ipsilateral insula, frontal, temporal, and parietal lobes, as well as the deep gray structures.
Is There Cerebral Edema?
Identifying the presence of edema in children is generally more challenging than in adults because the findings may appear more subtle and may be easily overlooked if the clinician is accustomed to interpreting adult head CTs. The normal pediatric brain has little space between the sulci and fissures, and ventricle size may be small. However, in the presence of edema, whether focal or diffuse, the brain volume enlarges compressing the cerebrospinal fluid (CSF) spaces. This loss of sulcation is frequently difficult to detect, so evaluating areas of the brain where sulci are normally seen (e.g., at the vertex) can be helpful ( Fig. 25.3 ). Although the ventricles in pediatric patients are normally smaller than those of adults, they should be visible and fairly symmetrical in size. Any asymmetries of the ventricles suggesting ventricular effacement or a slitlike appearance can be found in the setting of edema. Similarly, the sylvian fissures and basilar cisterns should normally be visible and symmetrical in the normal pediatric brain. It is important therefore to visualize the suprasellar, quadrigeminal plate, and perimesencephalic cisterns for the presence of edema.
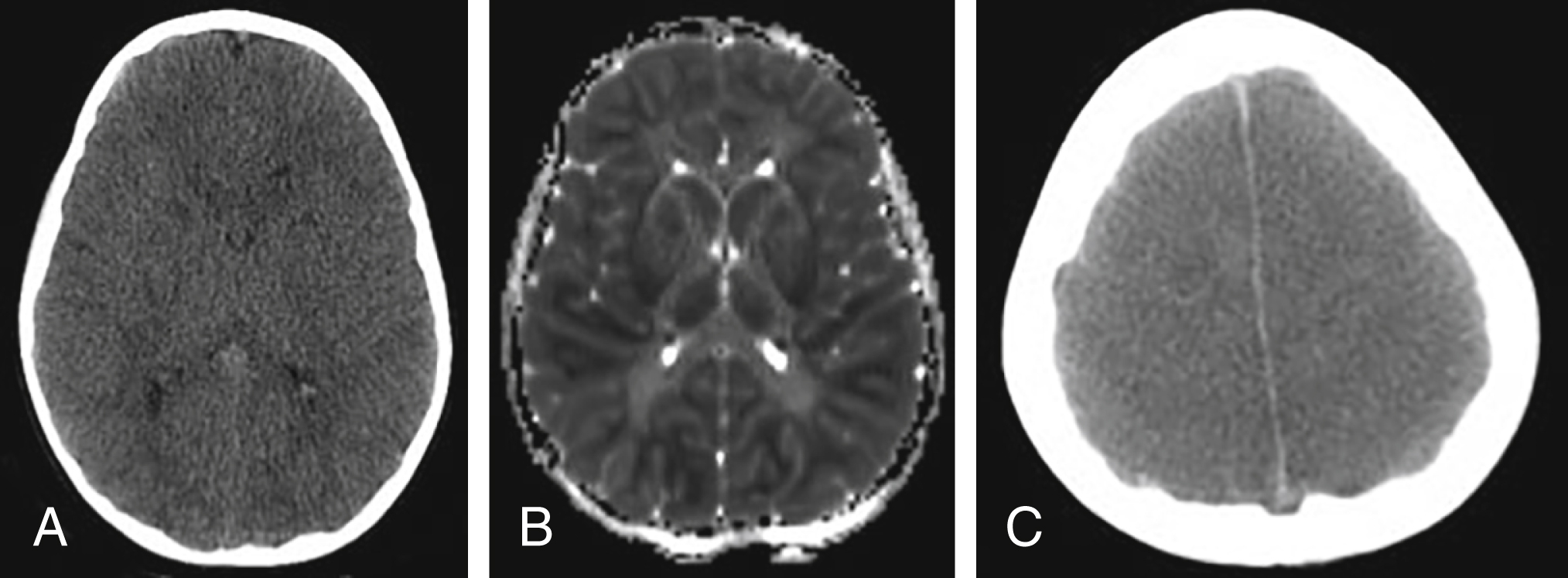
As edema progresses, there is loss of the gray/white differentiation (see Fig. 25.3A, C ). In children, it is important to evaluate the deep gray structures and ensure that the basal ganglia and thalami can be seen as distinct from the adjacent white matter (WM) structures, including the internal and external capsules and periventricular WM (see Fig. 25.3A–B ). Any blurring of the gray/white differentiation or diffuse haziness of the brain parenchyma should raise the possibility of cerebral edema.
Diffuse cerebral edema may arise from a number of causes in pediatric patients ( Box 25.1 ).
- •
Diabetic ketoacidosis
- •
Global anoxia: drowning/near drowning, arrest, hanging
- •
Hepatic or uremic encephalopathy
- •
Infection: sepsis, encephalomyelitis, meningitis
- •
Status epilepticus
- •
Trauma: diffuse axonal injury
- •
Toxin ingestion
The detection of diffuse edema can be challenging, however, because the brain may appear symmetrical. Important clues are blurring within the deep gray structures, effacement of the basilar cisterns, and nonvisualization of the sulci at the vertex. In addition, in the presence of diffuse cerebral edema, the cerebellum may appear hyperdense secondary to changes in low density within the edematous supratentorial brain known as the “white cerebellar” or “reversal” sign ( ).
Focal edema in the pediatric patient is attributable to a variety of causes ( Box 25.2 ). It may be easier to detect than the diffuse pattern, however, because asymmetry often exists in focal edema, with the contralateral side suggesting an abnormality. Certain diseases have a predilection for localizing to specific brain regions; thus familiarity with the common focal edema patterns is crucial to making a firm diagnosis. For example, herpes simplex encephalitis (HSE) should be considered when there is focal edema involving one or both medial temporal lobes, inferior frontal lobes, and/or insula, because this is a devastating, rapidly progressive, and potentially fatal infection. In the presence of edema involving the parietooccipital, watershed regions, cerebellum, and posterior frontal regions, especially in the clinical setting of hypertension or immunosuppressive drug use, a diagnosis of posterior reversible encephalopathy syndrome should be considered ( ; ). Edema confined to the cerebellum in a child may suggest cerebellitis. Cerebellitis can be seen with viral infections such as varicella zoster (after vaccinations) and in toxic encephalopathy in the setting of methadone overdose.
- •
Stroke
- •
Posterior reversible encephalopathy syndrome
- •
Seizure
- •
Metabolic conditions presenting acutely
- •
Demyelinating disease: acute disseminated encephalomyelitis, multiple sclerosis
- •
Infection: cerebellitis, encephalitis, abscess
- •
Mass: neoplasm, arteriovenous malformation, cavernoma with hemorrhage
Is There Mass Effect/Midline Shift or Herniation?
Once edema is identified as focal or diffuse, determining the displacement and/or distortion of brain structures locally and remotely is crucial to understanding the extent of the condition. Moreover, identifying the presence or absence of mass effect plays a greater role in management decisions than determining the specific cause of the edema. In addition, the cause of the mass effect may not be readily apparent on unenhanced head CT, and any displacement of adjacent structures may be the only clue to the presence of underlying pathology.
The brain has inherent symmetry, which should be considered when evaluating for the presence of mass effect. It is important first to identify the midline and, second, to ensure that key midline structures, such as the anterior and posterior falx, septum pellucidum, and pineal gland, are in fact at midline. Although the lateral ventricles may be slightly asymmetrical, they should maintain a smooth contour, and neither side should be effaced, compressed, nor shifted.
In addition to midline shift, it is also important to identify and report any herniation patterns ( Box 25.3 ) because specific types of herniations predispose the patient to additional complications secondary to the mass effect and may require immediate intervention. These herniation patterns are described later.
- •
Subfalcine
- •
Uncal
- •
Descending transtentorial
- •
Ascending transtentorial
- •
Tonsillar
Subfalcine herniation, the most common type of herniation pattern, results when the paramedian frontal lobe, typically the cingulate gyrus, is displaced to the opposite side of the falx cerebri. To identify this, a line should be drawn from the anterior falx posteriorly to the posterior falx to assess whether the paramedian frontal lobe is deviated across this line ( Fig. 25.4 ). As a result of subfalcine herniation, the anterior cerebral artery branches, which run within the interhemispheric fissure and cingulate sulci, can be compressed against, or be pulled under, the falx and secondarily occluded leading to infarcts within the anterior cerebral artery vascular territory. Also as a result of subfalcine herniation are trapping of the ventricles and hydrocephalus. As the frontal lobe and corpus callosum are pushed across to the opposite side, they may compress and obstruct the contralateral foramen of Monro, resulting in contralateral hydrocephalus (see Fig. 25.4 ).
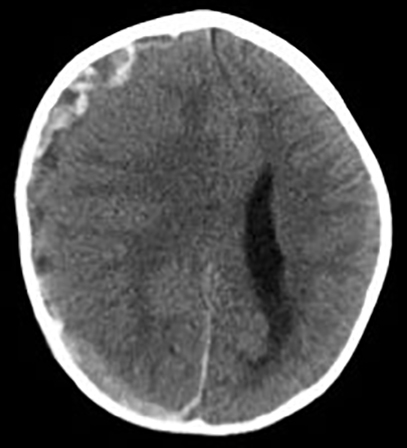
A second pattern of herniation requires identifying the uncus, the most medial part of the temporal lobe. Uncal herniation, a common type of descending transtentorial herniation, results when the uncus is displaced medially and inferiorly into the suprasellar cistern. As the herniation progresses, the hippocampus also herniates medially and effaces the quadrigeminal cistern. Eventually both uncus and hippocampus extend below the tentorial incisura, resulting in unilateral descending transtentorial herniation that compresses the midbrain. Uncal herniation may also compress the oculomotor nerve within the suprasellar cistern, resulting in pupillary dilation. It may also compress the ipsilateral posterior cerebral artery against the tentorium, giving rise to infarct in the posterior cerebral artery vascular territory.
With severe supratentorial mass effect, both medial temporal lobes may herniate medially and inferiorly push the midbrain and pons downward, reducing the angle between the midbrain and pons. When this occurs, it is termed central or bilateral descending transtentorial herniation .
When the mass effect occurs in the infratentorial brain, two types of herniation are seen. Typically the cerebellar tonsils will displace inferiorly through the foramen magnum, resulting in anterior displacement of the cervicomedullary junction and effacement of the fourth ventricle. This is most easily recognized on sagittal reformatted images. This type of herniation can occur with Chiari type I malformation acquired in patients with posterior fossa masses or posterior fossa edema ( Fig. 25.5 ), or may present with intracranial hypotension. Less commonly, or when the mass effect is severe, the cerebellum can displace upward through the tentorium. This displacement in turn effaces the quadrigeminal plate cistern, compresses the tectum, and possibly obliterates the cerebral aqueduct, resulting in hydrocephalus. This type of herniation pattern is termed ascending transtentorial herniation .
