Fig. 10.1
Simplified diagram of a striatal dopaminergic synapse. On the presynaptic side, potential markers for imaging of the integrity of dopaminergic neurons in humans are shown. [18F]FDOPA and [18F]FMT PET provide measures of the structural and biochemical integrity of the dopaminergic neurons. [11C]DTBZ is a commonly used radiopharmaceutical for the vesicular monoaminergic transporter. Substituted (nor)phenyltropanes ([11C]CFT, [11C]PE2I, [123I]β-CIT, [123I]FP-CIT and [99mTc]TRODAT) are frequently used PET and SPECT radioligands for imaging of the DAT in humans. On the postsynaptic sides, [11C]NNC112 and [11C]SCH23390 are commonly used antagonist radiopharmaceuticals for the dopamine D1 receptor. Dopamine D2 receptors are predominantly expressed on the postsynaptic side than on the presynaptic side of the dopaminergic synapse. [11C]NPA and [11C]PHNO are agonist radioligands for dopamine D2/3 receptors. Commonly used antagonist radioligands for D2/3 receptors are substituted benzamides ([11C]raclopride, [11C]FLB 457, [18F]fallypride and [123I]IBZM). DAT dopamine transporter, VMAT-2 vesicular monoaminergic transporter 2
As an alternative to [18F]FDOPA, the tyrosine derivative [18F]FMT has been developed. This radiotracer is similar to [18F]FDOPA in that both radiotracers are substrates of AADC. However, unlike [18F]FDOPA, [18F]FMT is not a substrate for catechol-O-methyl-transferase (COMT), an enzyme essential in the breakdown of dopamine. Therefore, there are no radioactive O-methyl-metabolites which contribute to the non-specific radioactivity in the brain, which enables the use of simplified kinetic modelling approaches (Dejesus et al. 2001).
The enzyme AADC plays an important role in the synthesis of dopamine, in that it converts L-DOPA to dopamine. It is important to consider that AADC also plays a role in the synthesis of other monoamine transmitters, e.g. in the conversion of 5-hydroxytryptophan to serotonin (5-HT). Since both [18F]FDOPA and [18F]FMT are substrates of AADC, these tracers are not only converted in dopaminergic neurons but also in serotonergic and noradrenergic neurons (Brown et al. 1999; Moore et al. 2003). The striatal accumulation of these tracers does however predominantly reflect conversion in nigrostriatal dopaminergic neurons (Pavese et al. 2012).
Initial PET studies applying [18F]FDOPA or [18F]FMT focused on evaluation of the nigrostriatal pathway, by studying the distribution of these tracers in the striatum (Antonini et al. 1995). Due to the better performance characteristics of the PET systems, as well as the improved PET data analysis methods, also extrastriatal radioactivity concentrations can nowadays be quantified (e.g. cortical areas). However, and as mentioned before, due to the expression of AADC in all monoaminergic neurons, accumulation in the raphe nuclei and locus coeruleus likely reflects predominantly serotonergic and noradrenergic activity, respectively, instead of accumulation in dopaminergic neurons (Ito et al. 2008; Lewis et al. 2012; Pavese et al. 2012). An important consideration when studying extrastriatal brain regions is that the reliability of measurements in extrastriatal regions is lower than in the striatum (Egerton et al. 2010).
Neuropsychiatric diseases like Parkinson’s disease and dementia with Lewy bodies are characterized by severe loss of dopaminergic nigrostriatal neurons. Consequently, many PET studies have utilized [18F]FDOPA and [18F]FMT to demonstrate the ability to detect loss of dopaminergic neurons in cohorts of these patients (Brooks et al. 1990; Antonini et al. 1995; Hu et al. 2000; Pavese et al. 2012). In addition, several [18F]FDOPA PET studies have been performed in patients suffering from psychosis, with the majority of them demonstrating an elevated striatal [18F]FDOPA uptake, which suggests an increased synthesis as well as dopamine turnover in these patients (Kumakura et al. 2007; Howes and Kapur 2009). [18F]FDOPA has also been applied to evaluate the role of dopamine in the human reward system (Dreher et al. 2008; Schlagenhauf et al. 2013). Finally, in healthy controls [18F]FDOPA and [18F]FMT PET have been used to assess ageing effects and cognitive functions (Braskie et al. 2008; Dreher et al. 2008; Kumakura et al. 2010).
10.2.2 Imaging of the VMAT-2
The vesicular monoamine transporter type 2 (VMAT-2; Fig. 10.1) is located in presynaptic dopaminergic neurons. Initially, carbon-11-labelled tetrabenazine derivatives have been developed successfully for PET imaging to visualize and quantify the VMAT-2 in humans (for a review, see Kilbourn 1997). Recently, also fluorine-18-labelled radioligands for the VMAT-2 have been developed successfully (Lin et al. 2010), which affords the opportunity to use these radioligands also in hospitals not equipped with a cyclotron.
VMAT-2 is not exclusively present in dopaminergic neurons, but also in other monoaminergic neurons, including serotonergic (Guillot and Miller 2009), although the vast majority of the striatal radioactivity concentration represents binding in dopaminergic nerve terminals (Wang et al. 2010). In this regard, it is of interest that it has been suggested that VMAT-2 radioligands can also be used to visualize loss of pancreatic beta-cell mass in type 1 diabetes (Goland et al. 2009), since beta-cells express VMAT-2. However, the specific binding of VMAT-2 PET radioligands binding to beta-cell mass has recently been disputed (Virostko et al. 2011).
Like [18F]FDOPA and [18F]FMT use in PET studies, VMAT-2 studies in humans have demonstrated that VMAT-2 imaging is a sensitive technique to detect reductions of nigrostriatal dopaminergic terminals in patients suffering from Parkinson’s disease or dementia with Lewy bodies (Frey et al. 1996; Okamura et al. 2010; Burke et al. 2011a; Villemagne et al. 2011). In addition, VMAT-2 binding has been evaluated in relation to natural ageing effects (Bohnen et al. 2006). Until now, SPECT radioligands have not been developed successfully for imaging of the VMAT-2.
10.2.3 Imaging of the Dopamine Transporter
The dopamine transporter (DAT) is mainly located in the membrane of terminals of presynaptic dopaminergic neurons (Fig. 10.1), but is also expressed at much lower levels in cell bodies in the substantia nigra (Ma et al. 1999). Radioligands for the DAT have been developed successfully for both PET and SPECT. Regarding SPECT radioligands, radiopharmaceuticals derived from cocaine, e.g. [99mTc]TRODAT-1, [123I]β-CIT and [123I]FP-CIT, are non-selective DAT radioligands, although binding in the striatum predominantly reflects binding to DAT (Laruelle et al. 1993; Booij et al. 1997a, 2007; Dresel et al. 1998; Ziebell et al. 2010; Koopman et al. 2012). Indeed, [123I]FP-CIT SPECT studies have shown that extrastriatal binding (in the diencephalon and midbrain) can be blocked by a selective serotonin reuptake inhibitor, indicating predominantly binding to serotonin transporters in these brain areas (Booij et al. 2007; Ziebell et al. 2010). In addition, also promising and DAT-selective SPECT ligands, like [123I]PE2I, have been developed and used in human subjects (Kuikka et al 1998; Ziebell et al. 2010).
PET radioligands for imaging of the DAT have been developed numerously over the last two decades (see for reviews Varrone and Halldin 2012a, b). Initially, non-selective DAT radioligands (e.g. [11C/18F]CFT, Rinne et al. 2002; Nurmi et al. 2003; [18F]FP-CIT; Oh et al. 2012) were applied in human studies, but more recently reported radioligands have an improved DAT selectivity (e.g. [11C]PE2I (Hirvonen et al. 2008) (Fig. 10.2) or [18F]FE-PE2I (Varrone et al. 2009; Sasaki et al 2012)). The developed PET radioligands also afford the opportunity to assess extrastriatal DAT binding, i.e. binding in the midbrain area and the orbitofrontal area (Jucaite et al. 2005; Hirvonen et al. 2008; Yagi et al. 2010), while with SPECT only DAT binding in the striatum can be assessed accurately.
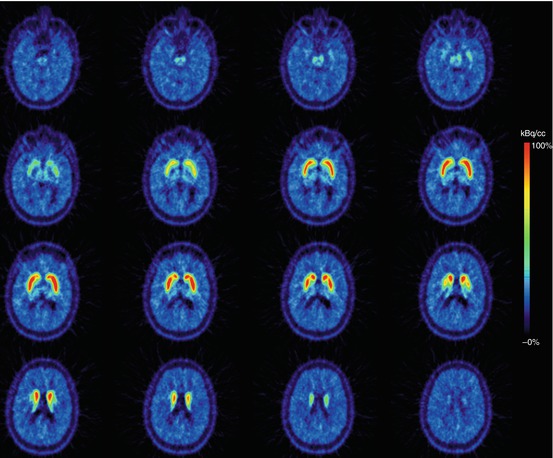
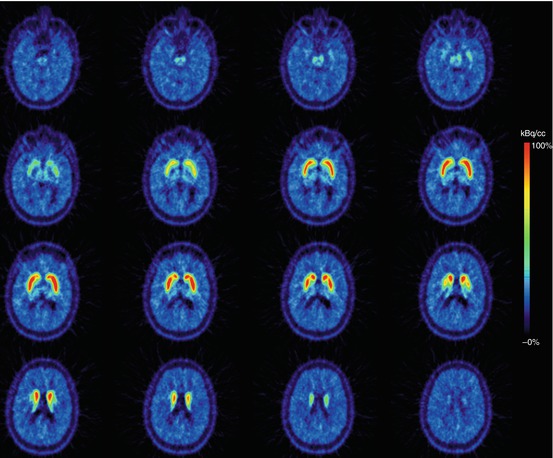
Fig. 10.2
Transversal planes of a mean PET summation image (6–93 min) obtained after i.v. injection of [11C]PE2I (selective radiotracer for the dopamine transporter) into a healthy male control subject. Intense specific binding is visible in the caudate nucleus and putamen and lower specific binding in extrastriatal brain areas (thalamus/midbrain). This study has been acquired on a brain-dedicated PET system (HRRT) (Images courtesy of Dr. Andrea Varrone and Professor Christer Halldin, Karolinska Institutet)
The DAT plays an important role in regulating dopaminergic neurotransmission. In this regard, appropriate regulation of the DAT expression is critical. Indeed, the DAT expression undergoes dynamic control by cellular protein kinases and phosphatases (Ramamoorthy et al. 2011). So, the DAT is not only expressed on the plasma membrane of dopaminergic neurons, as internalization of the DAT has been reported (Eriksen et al. 2009; Chen et al. 2010). Until now, it is not clear whether the abovementioned DAT radioligands label only plasma membrane-bound transporters or also internalized transporters when applied in PET/SPECT studies evaluating in vivo conditions (Earley et al. 2011). The radioligand binding characteristics to internalized transporters may differ from those transporters that are not internalized. Interestingly, Guo and co-workers have recently demonstrated that radioligands binding the dopamine D2/3 receptors have a lower affinity to internalized than to non-internalized receptors (Guo et al. 2010). Future studies are necessary to evaluate whether DAT internalization is a relevant consideration for in vivo imaging of DAT.
Numerous PET and SPECT studies evaluating DAT binding have shown the possibility to detect in vivo degeneration of nigrostriatal cells in disorders like Parkinson’s disease and dementia with Lewy bodies (Booij et al. 1997b; Varrone et al. 2001; McKeith et al. 2007; Yagi et al. 2010; Oh et al. 2012). Also, DAT binding has been examined in relation to ageing and gender effects in healthy controls, as well as the effects of polymorphism of the DAT gene (Lavalaye et al. 2000; van Dyck et al. 2005; van de Giessen et al. 2009; Troiano et al. 2010; Burke et al. 2011b). Generally speaking, brain SPECT imaging of neuroreceptors/transporters is cheaper than PET imaging, and consequently DAT imaging with SPECT is commonly used in routine clinical studies to exclude or detect loss of nigrostriatal neurons in individual patients (Booij et al. 2001; Løkkegaard et al. 2002; Catafau et al. 2004; Ziebell et al. 2012).
10.3 Imaging of the Postsynaptic Dopaminergic System
10.3.1 Imaging of Dopamine D1 Receptors
Dopamine exerts its pharmacological action through G-protein-coupled receptors. These transmembrane receptors can be divided into two subfamilies based on their pharmacological properties: the D1– (D1, D5) and D2-like receptor subfamily (D2, D3, D4; Stoof and Kebabian 1981; Strange 1993; Beaulieu and Gainetdinov 2011).
The dopamine D1 receptor is a highly prevalent dopamine receptor in the striatum and neocortex and is located postsynaptically (Cortés et al. 1989; Volkow et al. 1996). The radiopharmaceuticals [11C]NNC 112 and [11C]SCH 23390 have successfully been developed to image dopamine D1 receptors in the living human brain using PET (Fig. 10.3). Both radioligands are high-affinity dopamine D1 receptor antagonists, although they also have been reported to bind to serotonin 2A (5-HT2A) receptors, estimated as approximately 25 % of cortical binding being due to binding to 5-HT2A receptors (Ekelund et al. 2007). To circumvent this limitation, a recent study proposed the use of the 5-HT2A receptor antagonist ketanserin. After ketanserin administration, the 5-HT2A receptor binding is inhibited, and cortical D1 receptor binding can be accurately assessed using [11C]NNC 112 in humans (Catafau et al. 2010).
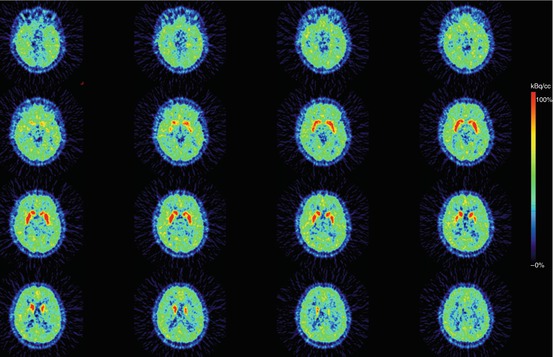
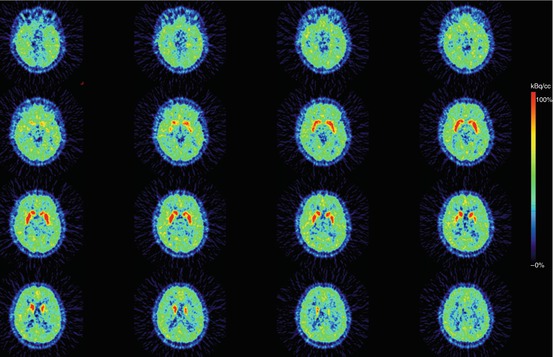
Fig. 10.3
Transversal plane of a mean PET summation image (12–63 min) obtained after i.v. injection of [11C]SCH 23390 (radiotracer for the dopamine D1 receptor) into a healthy male control subject. Intense specific binding is visible in the caudate and putamen, and lower specific binding in the extrastriatal brain areas (cortex). This study has been acquired on a brain-dedicated PET system (ECAT EXACT HR) (Images are courtesy of Per Stenkrona and Professor Christer Halldin, Karolinska Institutet)
Although [11C]NNC 112 and [11C]SCH 23390 bind to both dopamine D1 and 5-HT2A receptors, the affinity to dopamine D2-like receptors is substantially lower (Andersen et al. 1992) although binding to dopamine D5 receptors cannot be excluded (Sunahara et al. 1991; Chou et al. 2006). While dopamine D1 receptors are expressed at high levels in the striatum and cortical areas, the expression of dopamine D5 receptors in the brain is low (Beaulieu and Gainetdinov 2011). Consequently, in vivo binding to dopamine D5 receptors, as compared to D1 receptors, with PET radioligands like [11C]NNC 112 is likely negligible.
Dopamine receptors have been demonstrated to exist in two affinity states in vitro. The two receptor states are convertible and consist of a state of high and low affinity for the endogenous agonist dopamine (or exogenous agonists) (Sibley et al. 1982; Chio et al. 1994). The high-affinity state represents the active form of the receptors that are coupled to G proteins (Zahniser and Molinoff 1978). Regarding dopamine D1 receptors, results of in vitro experiments suggest that 20–40 % of these receptors are in the high-affinity state (Richfield et al. 1989; Mamelak et al. 1993; McCauley et al. 1995). Importantly, while antagonist radioligands label both the high- and low-affinity state of the receptors, agonist radioligands may label receptors only in its high-affinity state. So far, the available radioligands [11C]NNC 112 and [11C]SCH 23390 are both antagonists for the dopamine D1 receptor. Developments of agonist radioligands for the dopamine D1 receptor are ongoing and will enable to examine in future studies whether the high-affinity state of the dopamine D1 receptor exists in living humans (Palner et al. 2010). Also, dopamine agonist radioligands may be more sensitive to detect changes in dopamine concentrations in the synapse, e.g. changes induced by dopamine releasers like amphetamines (see below).
Since dopamine D1 receptors have been implicated in cognitive performance, dopamine D1 PET studies in healthy volunteers have been used to examine changes in working memory (McNab et al. 2009), amygdala response to affective stimuli (Takahashi et al. 2010) and natural ageing (Jucaite et al. 2010) and to study the effects of genes involved in the dopaminergic tone (Slifstein et al. 2008). Dopamine D1 receptor imaging in neuropsychiatric disorders has so far focused on schizophrenia (and the mechanism of action of antipsychotics) and cocaine addiction (Nordström et al. 1995; Karlsson et al. 2002; Hirvonen et al. 2006; Martinez et al. 2009). Until now, the development of SPECT radioligands for the dopamine D1 receptor has not been successful.
10.3.2 Imaging of Dopamine D2-Like Receptors
10.3.2.1 Dopamine D2/3 Receptor Imaging
In the past three decades, PET as well as SPECT radioligands have been developed successfully to image and quantify dopamine D2/3 receptors in the human brain.
Regarding SPECT imaging, the commonly used benzamide [123I]IBZM is an antagonist radioligand which binds with high affinity to dopamine D2/3 receptors (i.e. in the low nanomolar range) (Verhoeff et al. 1993; Videbaek et al. 2000; de Haan et al. 2003; Boot et al. 2008; Visser et al. 2008). With this radioligand striatal dopamine D2/3 receptors can be assessed accurately, and extrastriatal distribution may be visualized, particularly when using brain-dedicated SPECT systems (Fig. 10.4). The radioligand [123I]epidepride is a dopamine D2/3 receptors antagonist with an enhanced affinity (picomolar range) for the D2/3 receptors (Kessler et al. 1991). This ultrahigh-affinity SPECT radioligand allows assessing D2/3 receptors availability in extrastriatal brain areas, such as the midbrain, diencephalon and cortical areas (Kornhuber et al. 1995; Varrone et al. 2000; Glenthoj et al. 2006; Tuppurainen et al. 2010).
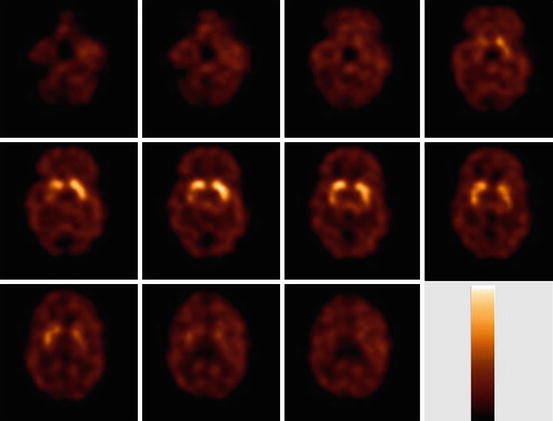
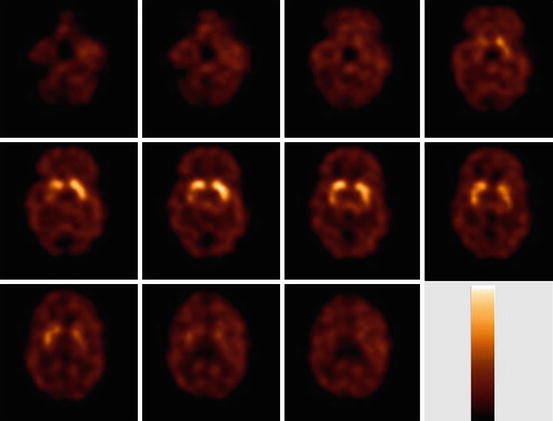
Fig. 10.4
Transversal [123I]IBZM slices obtained in a healthy control, 2 h after start of bolus/constant infusion. Intense specific binding is visible in the striatum, and much lower specific binding in the extrastriatal brain areas (thalamus/midbrain). The used colour scale represents a maximum intensity scale. This study has been acquired on a brain-dedicated SPECT system (Neurofocus)
Regarding PET imaging, the benzamide [11C]raclopride is an antagonist radioligand that binds with high affinity to dopamine D2/3 receptors (in the low nanomolar range; Farde et al. 1986) and is commonly used in human studies (Ito et al. 2011; Martinez et al. 2011). The radioligand [11C]N-methylspiperone binds also with high affinity to dopamine D2/3 receptors, although binding has also been shown to be partly related to 5-HT2A receptors (Nyberg et al. 1999). Also antagonist PET radioligands with ultrahigh affinity for the dopamine D2/3 receptors have been developed successfully. For example, [11C]FLB 457 (Halldin et al. 1995) and [18F]fallypride (Mukherjee et al. 1995) are radioligands with a very high receptor affinity allowing for evaluation of extrastriatal dopamine D2/3 receptors (picomolar range; Mukherjee et al. 1995; for a review see Högberg 1993).
More recently, at least three agonist PET radioligands for dopamine D2/3 receptors have been developed and tested successfully in humans, that is [11C]PHNO, [11C]NPA and [11C]MNPA (Willeit et al. 2006; Laymon et al. 2009; Otsuka et al. 2009). The apomorphine derivatives NPA and MNPA bind with high affinity to both dopamine D2 and D3 receptors (Seeman et al. 1985; Neumeyer et al. 1990). In contrast, in vitro studies have shown that the affinity of PHNO is 30- to 50-fold lower for the dopamine D2 than for the D3 receptor (Freedman et al. 1994; van Vliet et al. 2000). Indeed, a recent study in humans demonstrated that binding of [11C]PHNO in several brain regions, e.g. hypothalamus, substantia nigra, ventral pallidum and globus pallidus, is predominantly to dopamine D3 receptors. On the other hand, in striatal subregions, in vivo binding is predominant to dopamine D2 receptors (Tziortzi et al. 2011). Although the agonist [11C]PHNO binds predominantly to dopamine D3 receptors in certain brain areas, selective radioligands for dopamine D2 or D3 receptors are not available yet.
Regarding dopamine D2 receptors, in vitro experiments on homogenized striata from rats and mice show that roughly 15–30 % of the receptors are in the high-affinity state with a range up to 80 % depending on the detection method used. In more intact tissue (brain slices), 90 % of these receptors may even be in the high-affinity state (van Wieringen et al. 2013). Initial results of in vivo PET measurements using amphetamine in non-human primates suggested that 60–80 % of these receptors are in the high-affinity state (Narendran et al. 2004). However, although several attempts have been made, formerly the existence of the high- and low-affinity state has until now not been confirmed or disputed in vivo (Finnema et al. 2009; see for reviews Finnema et al. 2010; Skinbjerg et al. 2012). The results of the recent application of agonist radioligands in human PET studies suggest that 60–65 % of dopamine D2 receptors are in the high-affinity state in vivo (Narendran et al. 2010; Shotbolt et al. 2012).
As discussed earlier, dopamine agonist radioligands may be more sensitive to detect changes in dopamine concentrations in the synapse, e.g. changes induced by dopamine releasers like amphetamines (Laruelle et al. 1995) or depletion induced by AMPT (Laruelle et al. 1997; Boot et al. 2008). Indeed, recent studies have indicated that the agonist radioligands ([11C]NPA and [11C]PHNO) are more vulnerable to endogenous dopamine competition than the antagonist radioligand [11C]raclopride in humans (Narendran et al. 2010; Shotbolt et al. 2012). It can be concluded that agonist dopamine D2/3 receptors radioligands hold promise for studying changes in dopamine concentrations although further confirmation in human subjects is required.
The dopamine D2/3 receptors are not only expressed on the plasma membrane of postsynaptic neurons, but also presynaptically (Fig. 10.1). There are two splice variants of dopamine D2 receptors, the dopamine D2 long (D2L) and the short variant (D2S) (Chio et al. 1990). These isoforms have different localizations, with D2S primarily located presynaptically (as an autoreceptor) (Khan et al. 1998). From a pharmacological point of view, these splice variants cannot be discriminated, and consequently, radioligands for the dopamine D2/3 receptors may bind to both D2L and D2S. However, since the striatal density of D2L is much higher than that of D2S (Rani and Kanungo 2006), imaging of dopamine D2/3 receptors is frequently referred to as imaging of postsynaptic D2/3 receptors. Also, imaging of the midbrain dopamine D2/3 receptors may predominantly visualize dopamine D2/3 autoreceptors (Buckholtz et al. 2010).
Dopamine D2 receptors can internalize into the intracellular compartment after agonist stimulation, similar as for other G-protein-coupled receptors. Interestingly, Guo and co-workers recently reported that dopamine D2 receptor radioligands have a lower affinity to internalized than to non-internalized receptors (Guo et al. 2010). A frequently used approach to assess dopamine release in vivo is the use of dexamphetamine (see for review Laruelle 2000). Administration of dexamphetamine intravenously or orally in doses of 0.2–0.3 mg/kg body weight induces a substantial release of endogenous dopamine, which will result in a displacement of radioligand binding to dopamine D2/3 receptors (i.e. decrease of, e.g. striatal [123I]IBZM or [11C]raclopride binding; Laruelle et al. 1995; Breier et al. 1997). Typically, administration of dexamphetamine leads to a rapid release of extracellular dopamine which is not long lasting (Breier et al. 1997). However, in imaging studies a prolonged displacement of radioligand binding has been observed, which may be caused (partly) by receptor internalization (Laruelle 2000; Cárdenas et al. 2004; Scott et al. 2007). Indeed, studies in knockout mice, which are incapable of internalizing dopamine D2 receptors, suggest that the prolonged displacement is mainly due to internalization of D2 receptors (Skinbjerg et al 2010). Additional studies are needed to demonstrate whether molecular imaging studies are able to assess internalization of dopamine D2 receptors in humans, which may be relevant to study the role of a possible abnormal internalization of these receptors in neuropsychiatric disorders.
10.3.2.2 Dopamine D4 Receptor Imaging
The dopamine D4 receptor was cloned for the first time in 1991 (Van Tol et al. 1991) and is thought to play a role in a variety of neuropsychiatric disorders. This receptor is mainly expressed postsynaptically (Rivera et al. 2002). In contrast to dopamine D2/3 receptors, dopamine D4 receptors do not internalize after agonist stimulation (Spooren et al. 2010). Interestingly, the antipsychotic clozapine, for instance, demonstrates a substantially higher affinity for the dopamine D4 than for the D2 receptor subtype (Van Tol et al. 1991; Eisenegger et al. 2010; Smith 2010). Very recently, progress has been reported on the development of a dopamine D4 receptor radioligand. This has been a challenging task as the density of dopamine D4 receptors in the human brain is much lower than that of other D2-like receptors (Marazziti et al. 2009) (Lacivita et al. 2010; Kügler et al. 2011). Although Lacivita and co-workers reported that their radioligand mainly demonstrated high binding in the retina of non-primates (Lacivita et al. 2010), it is promising that a recent study demonstrated clear brain uptake of a dopamine D4 receptor antagonist radioligand in mice (Kügler et al. 2011).
10.4 Conclusion
Imaging of the nigrostriatal pathway in humans can be performed quantitatively with PET and SPECT techniques, using the PET radiotracer [18F]FDOPA, PET radioligands for the VMAT-2 and PET or SPECT radioligands for the DAT. With PET, also several other dopaminergic projections (e.g. mesocortical projections) can be assessed adequately in the human brain. Several antagonist PET radioligands for the dopamine D1 receptor have been developed successfully. In addition, well-validated antagonist PET and SPECT radioligands are available to image dopamine D2/3 receptors in the living human brain. Recently, also agonist PET radioligands for the dopamine D2/3 receptors have been developed, which affords the opportunity to evaluate the existence of the high-affinity state of these receptors in vivo, and these radiopharmaceuticals may be more sensitive to detect changes in dopamine concentrations. Finally, although selective antagonist PET radioligands for the dopamine D4 receptor have been synthesized and evaluated successfully in small laboratory animals, these radioligands have not yet been applied in human research.
References
Andersen PH, Grønvald FC, Hohlweg R et al (1992) NNC-112, NNC-687 and NNC-756, new selective and highly potent dopamine D1 receptor antagonists. Eur J Pharmacol 219:45–52
Antonini A, Vontobel P, Psylla M et al (1995) Complementary positron emission tomographic studies of the striatal dopaminergic system in Parkinson’s disease. Arch Neurol 52:1183–1190PubMed
Beaulieu JM, Gainetdinov RR (2011) The physiology, signaling, and pharmacology of dopamine receptors. Pharmacol Rev 63:182–217PubMed
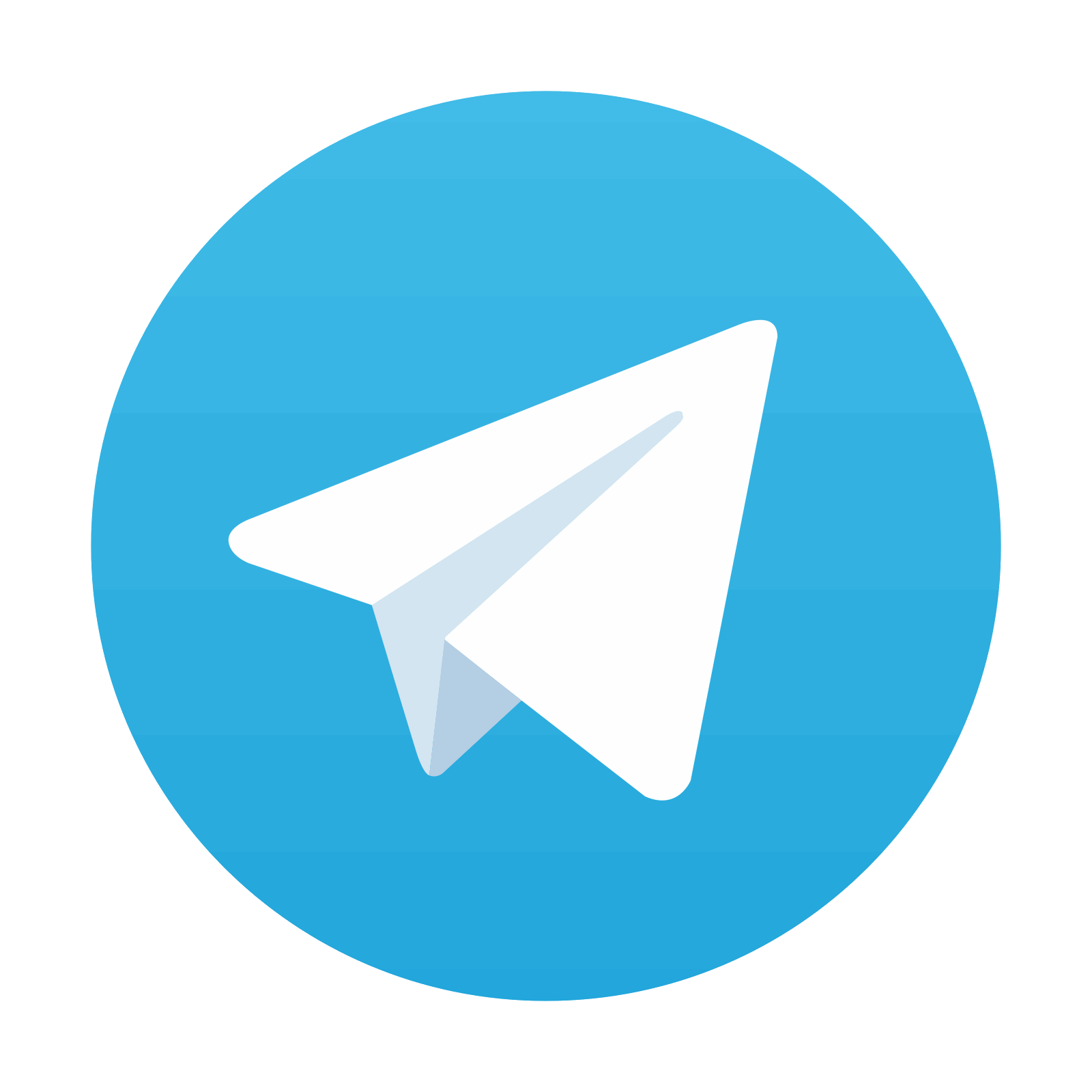
Stay updated, free articles. Join our Telegram channel

Full access? Get Clinical Tree
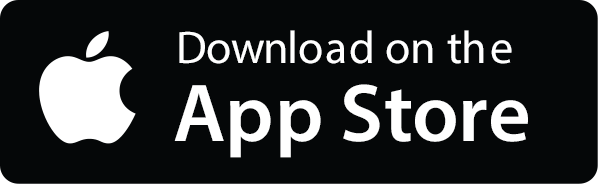
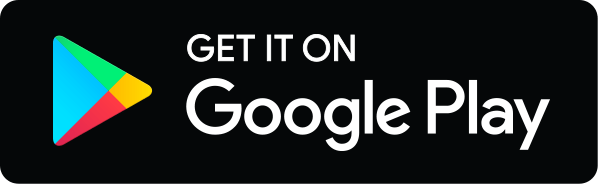