Fig. 16.1
Chemical structures of 4-MPB (left), (+)3-MPB and its N-alkyl substitution analogues, (+)3-EPB and (+)3-PPB (right). Affinity (Ki) values of each compound were determined by in vitro assay with [3H]QNB
Thereafter, Takahashi et al. proposed another novel mAChR probe, N-[11C]methyl-3-piperidyl benzilate ([11C]3-MPB) (Takahashi et al. 1997) (Fig. 16.1). Since its chemical structure contained a chiral carbon, it provided two stereoisomers, the active form as [11C](+)3-MPB and inactive one as [11C](−)3-MPB, and even active [11C](+)3-MPB revealed relatively low affinity to mAChR as determined by in vitro binding assay (Takahashi et al. 1997). To extend prior work in the rat brain, [11C](+)3-MPB was evaluated for quantification of cerebral mAChR binding in the monkey brain (Tsukada et al. 2001c). With [11C](+)3-MPB and its analogues (Nishiyama et al. 2001), we determined the aging effects on mAChR binding in the monkey brain (Tsukada et al. 2001a, 2004). In addition, the temporal relationship between the occupancy level of central mAChR by scopolamine and anticholinergic drugs for overactive bladder (OAB) treatment, as measured by [11C](+)3-MPB, and cognitive impairment, as assessed by a delayed matching to sample task, was determined in conscious monkeys (Yamamoto et al. 2011a, b). These monkey PET imaging studies were conducted with a high-resolution animal PET scanner which allowed us to image the brain under conscious conditions.
The PET studies reported in this chapter have been conducted with monkeys in conscious condition. Anesthetics have been used in nonhuman primate PET studies, because it is necessary to fix animal during PET scanning. However, anesthetics affect several neuronal functions, resulting in an alteration of neuronal activities in the central nervous system. Thus, the indices of brain function as well as the pharmacological actions as measured by PET should be affected by the anesthetics. In order to avoid these anesthetic effects, we developed a sophisticated total PET system (Hamamatsu SHR-7700, Watanabe et al. 1997) to conduct an “activation study” with conscious behaving monkeys (Onoe et al. 2001; Yokoyama et al. 2005; Nishimura et al. 2007). Its gantry including optical detectors can tilt up to 90° at any angle, and brain images of monkey sitting on a monkey chair can be measured even in the conscious condition. This PET system enabled us to conduct PET imaging of mAChR with [11C](+)3-MPB without any effects of anesthetics, making it easier to conduct the translational research of [11C](+)3-MPB from animals to humans.
Based on the conscious monkey PET studies, we have just started several translational studies with [11C](+)3-MPB from preclinical level with experimental animals to clinical levels with humans. We checked the safety issue of [11C](+)3-MPB based on the microdose concept and recently reported that chronic fatigue syndrome (CFS) patients with positive serum autoantibody against mAChR showed significantly lower [11C](+)3-MPB binding than negative patients and normal controls.
16.2 Development of [11C](+)3-MPB and Its Analogues
16.2.1 Assessment of [11C](+)3-MPB in Comparison with [11C]4-MPB
[11C](+)3-MPB and its stereoisomer [11C](−)3-MPB were evaluated and compared with the conventional muscarinic receptor ligand [11C]4-MPB for quantification of cerebral muscarinic receptors in the monkey brain. To minimize the effects of anesthetics on receptor binding, conscious monkeys were utilized in the present study (Tsukada et al. 2000a, 2001b; Ohba et al. 2009).
Positron emitting carbon-11 (11C) was produced by 14N(p,α)11C nuclear reaction using a cyclotron and chemical converted to [11C]methyl iodide. [11C](+)3-MPB and its stereoisomer [11C](−)3-MPB were labeled by N-methylation of their respective nor-compounds, (+)3-PB and (−)3-PB, with [11C]methyl iodide (Takahashi et al. 1997; Tsukada et al. 2001c). As a reference, [11C]4-MPB (Mulholland et al. 1988a; Zubieta et al. 1998) was also labeled by N-methylation of 4-PB.
PET imaging data were collected on a high-resolution PET scanner (HAMAMATSU SHR-7700) with transaxial resolution of 2.6 mm full width at half maximum (FWHM) and a center-to-center distance of 3.6 mm (Watanabe et al. 1997).
Monkeys (Macaca mulatta) were trained to sit on a chair with head motion restriction using a specially designed head holder twice a week for more than 3 months (Onoe et al. 1994). After an overnight fast, a monkey was seated on the monkey chair under conscious condition and fixed with stereotactic coordinates aligned parallel to the orbitomeatal (OM) line. Each labeled compound (100–120 MBq/kg body weight) was injected through the venous cannula. The radioactive purity of each labeled compound used in this study was greater than 99 % and the specific radioactivity ranged from 61.7 to 92.4 for [11C](+)3-MPB, from 60.0 to 79.5 for [11C](−)3-MPB, and from 34.4 to 75.9 GBq/μmol for [11C]4-MPB. Each labeled compound was injected through the venous cannula, and a PET scan was made for 91 min. Regions of interest (ROI) were identified according to MR images of each monkey brain, and time-activity curves for these ROIs were calculated. To measure the input function of each labeled compound into the brain, arterial blood samples were obtained, the ratio of radioactivity in the unmetabolized fraction to that in total plasma was determined with a thin layer chromatography (TLC) plate. Logan (Logan et al. 1990) and Patlak’s (Patlak et al. 1983) graphical analyses were applied to measure the net accumulation of tracer in the reversible and irreversible compartment, respectively.
In Fig. 16.2, typical PET images of [11C](+)3-MPB (A), [11C](−)3-MPB (B), and [11C]4-MPB (C) are shown obtained in the same monkey under conscious condition. The regional uptake pattern of [11C](+)3-MPB was high in the striatum; intermediate in the occipital, temporal, and frontal cortices and hippocampus and thalamus; and low in the cerebellum (Fig. 16.2A). In contrast, the level of [11C](−)3-MPB was much lower than those of [11C](+)3-MPB in all regions of the brain (Fig. 16.2B). The patterns of distribution of [11C]4-MPB (Fig. 16.2C) were almost identical to that of [11C](+)3-MPB.
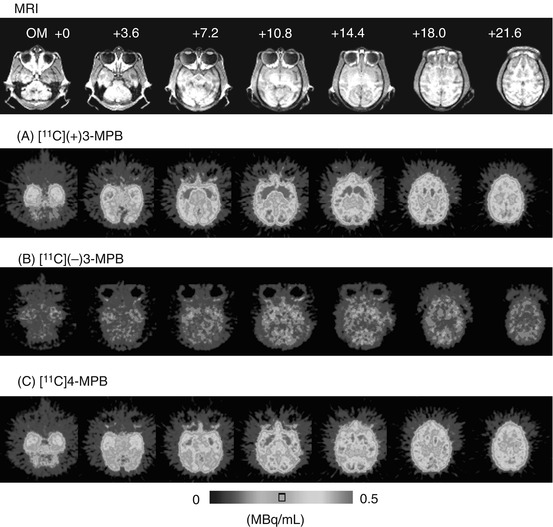
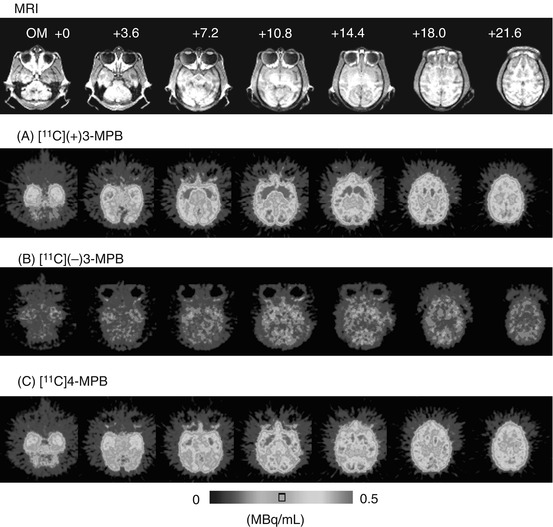
Fig. 16.2
MRI and PET images of [11C](+)3-MPB (A), [11C](−)3-MPB (B), and [11C]4-MPB (C) in the monkey brain. MR images of monkeys were obtained with a Toshiba MRT-50A/II (0.5 T). PET data were collected in the conscious state with a high-resolution PET scanner (HAMAMATSU SHR-7700) with a transaxial resolution of 2.6 mm (FWHM). Each labeled compound (100–120 MBq/kg body weight) was injected through the venous cannula. PET scans were performed for 91 min, and each PET image was generated by summation of image data from 60 to 94 min postinjection
The time-activity curves of [11C](+)3-MPB in the frontal, temporal, and occipital cortices reached their peaks 40 min after injection, whereas the striatal and hippocampal regions reached peak values 60 min after injection (Fig. 16.3a). In contrast, the time-activity curves of [11C](−)3-MPB showed similar patterns in all regions of the brain (Fig. 16.3b). Uptake of [11C]4-MPB in all regions except the thalamus and cerebellum gradually increased with time during the scan until 91 min after injection (Fig. 16.3c).
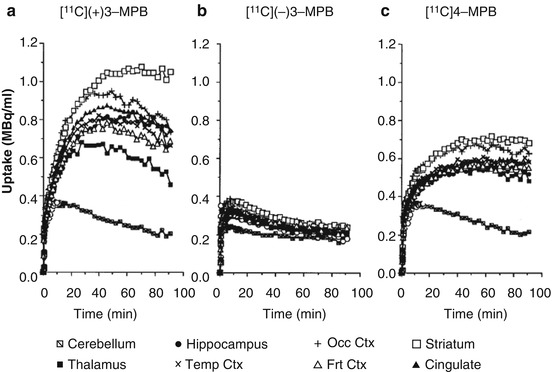
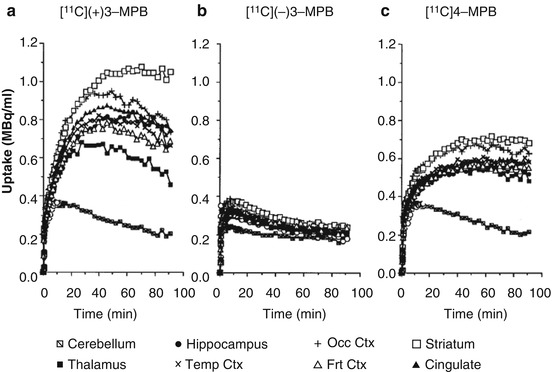
Fig. 16.3
Time-activity curves (TAC) of [11C](+)3-MPB (a), [11C](−)3-MPB (b), and [11C]4-MPB (c) in the conscious monkey brain. PET scans were performed as shown in the legend of Fig. 16.2. Regions of interest (ROls) were identified according to a MRI scan of the each animal
Patlak’s plot analysis demonstrated that [11C]4-MPB provided linear regression curves in all regions evaluated, while [11C](+)3-MPB provided nonlinear curves showing slope = 0 in late phase, suggesting that the dissociation rate constant (k4) from muscarinic cholinergic receptors was not negligible for [11C](+)3-MPB. Therefore, Logan plot analysis was applied to investigate the in vivo binding of [11C](+)3-MPB. The ratio of Logan slopes of [11C](+)3-MPB in each region against the cerebellum as reference region, and Patlak’s slope of [11C]4-MPB were correlated with the data of muscarinic cholinergic receptor density (Bmax) as measured by the in vitro assay (Snyder et al. 1975). These results demonstration that [11C](+)3-MPB was a more suitable [11C]labeled ligand for the quantification of muscarinic cholinergic receptors in the brain with PET than conventional [11C]labeled ligands such as [11C]4-MPB and [11C]scopolamine.
16.2.2 N-Substitution Analogues of [11C](+)3-MPB with Longer Alkyl Chain Length
The assessment of neurotransmitter release in the living brain should be very important from the neuroscientific and pharmacological points of view. Microdialysis method is the classical method to evaluate neurotransmitter release; however, since insertion of the needle-shaped dialysis probe into the brain is quite invasive, human studies would be difficult to justify. In the last decade, interactions between endogenous neurotransmitters released into the synaptic cleft and the binding of labeled compounds have been examined by neuroimaging with PET/SPECT, especially in the dopaminergic system (for review, see Laruelle 2000; Tsukada et al. 2000a, b). Thus, the changes in synaptic neurotransmitters could be measured noninvasively by PET using labeled compounds, which have moderate affinity for their specific receptors. In order to develop a PET probe to assess acetylcholine release into the synaptic cleft, we developed two N-substitution analogues of [11C](+)3-MPB, (+)N-[11C]ethyl-3-piperidyl benzilate ([11C](+)3-EPB) and (+)N-[11C]propyl-3-piperidyl benzilate ([11C](+)3-PPB), and evaluated their specificity, affinity, and kinetics in comparison with original [11C](+)3-MPB in the conscious monkeys (Nishiyama et al. 2001) (Fig. 16.1). Since previous studies have suggested that N-substitution of 4-PB with longer alkyl chain length affected their affinities in vitro and anticholinergic potencies in vivo (Tejani-Butt et al. 1990), the affinities of (+)3-MPB, (+)3-EPB, and (+)3-PPB for mAChR were assessed with in vitro competition binding assay of [3H]quinuclidinyl benzilate (QNB) using rat brain coronal slices before PET study, resulting in K i values of 1.7, 12.9, and 47.5 nM, respectively.
Carbon-11 (11C) was produced by 14N(p,α)11C nuclear reaction using a cyclotron and chemically converted into [11C]ethyl or [11C]propyl iodide by reaction with the appropriate Grignard reagent (Långström et al. 1986). [11C](+)3-EPB or [11C](−)3-EPB was synthesized by N-ethylation or N-propylation of (+)3-PB with [11C]ethyl iodide or [11C]propyl iodide using an automated synthesizer developed in our laboratory (Nishiyama et al. 2001). PET data acquisition, cortical TAC, and plasma metabolic analyses were conducted as described in Sect. 16.2.1. TACs for metabolite-corrected arterial plasma (input function) and for each ROI were fitted to a three-compartment model with a least-square fitting method to estimate the kinetic parameters, and the values of binding potential (BP = k3/k4) for mAChR in each region were calculated.
Figure 16.4 shows typical PET images of [11C](+)3-MPB (a), [11C](+)3-EPB (b), and [11C](+)3-PPB (c) in the conscious monkey brain. The regional distribution patterns of these three labeled compounds were well correlated with data on mAChR density as measured by in vitro assay (Snyder et al. 1975). The time-activity curves demonstrated that the peak times shifted earlier implying a faster clearance rate after injection of ligands with longer [11C]alkyl chain lengths (Fig. 16.5). Kinetic analysis revealed that labeling with longer [11C]alkyl chain length induced lower binding potential (BP = k3/k4 in Frontal cortex; 2.4, 2.0, and 1.4 for [11C](+)3-MPB, [11C](+)3-EPB, and [11C](+)3-PPB, respectively), consistent with the rank order of affinity of these ligands determined by an in vitro assay. The administration of Aricept, a cholinesterase inhibitor, increased acetylcholine level in extracellular fluid of the frontal cortex (ca. 150 and 175 % of baseline at 50 and 150 μg/kg, respectively) as measured by microdialysis in the conscious condition (Nishiyama et al. 2001) according to the method previously reported (Tsukada et al. 2000c). The binding of [11C](+)3-PPB with the lowest affinity to the receptors was displaced by the endogenous acetylcholine induced by cholinesterase inhibition, while [11C](+)3-MPB with the highest affinity was not significantly affected (Fig. 16.6). These results suggested that increasing [11C]alkyl chain length did alter the kinetic properties of PET probes by reducing the affinity to mAChR, which might make it possible to assess the interaction between the endogenous neurotransmitter acetylcholine and ligand-receptor binding in vivo as measured by PET.
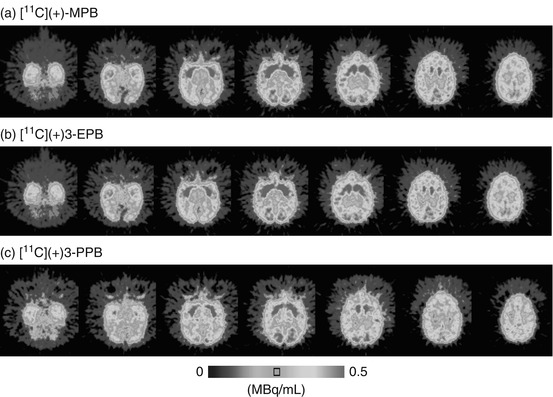
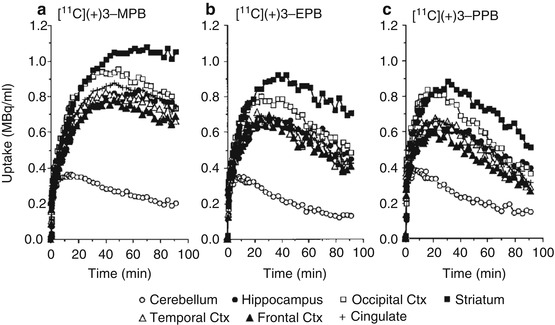
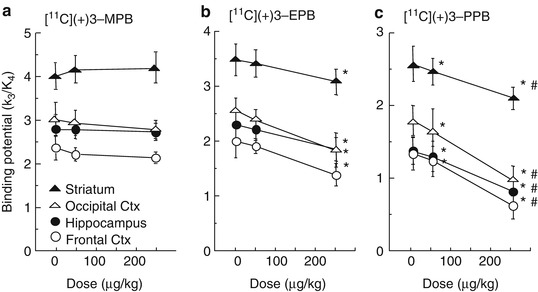
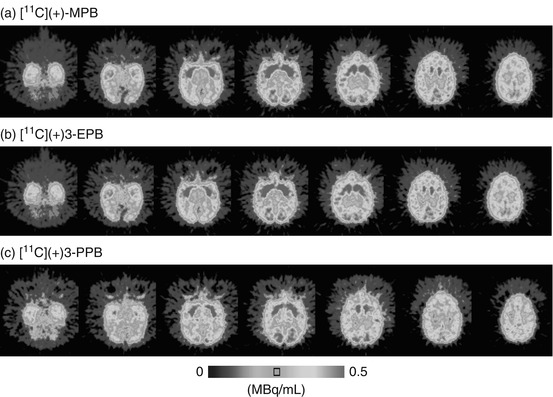
Fig. 16.4
MRI and PET images of [11C](+)3-MPB (a), [11C](+)3-EPB (b), and [11C](+)3-PPB (c). MR images of monkeys were obtained with a Toshiba MRT-50A/II (0.5 T). PET data were collected in the conscious state with a high-resolution PET scanner (HAMAMATSU SHR-7700). Each labeled compound (100–120 MBq/kg body weight) was injected through the venous cannula. PET scans were performed for 91 min, and each PET image was generated by summation of image data from 60 to 94 min postinjection (Tsukada et al. 2001b)
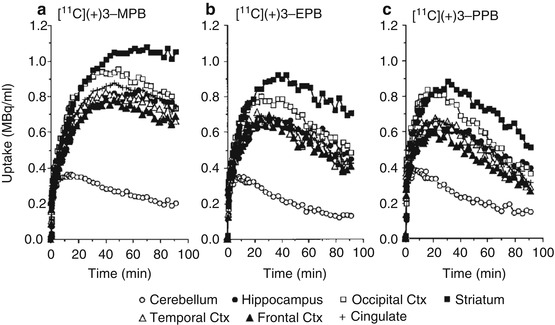
Fig. 16.5
Time-activity curves of [11C](+)3-MPB (a), [11C](+)3-EPB (b), and [11C](+)3-PPB (c) in the conscious monkey brain. PET scans were performed as described in the legend of Fig. 16.4. Regions of interest (ROls) were identified according to a MRI scan of each animal
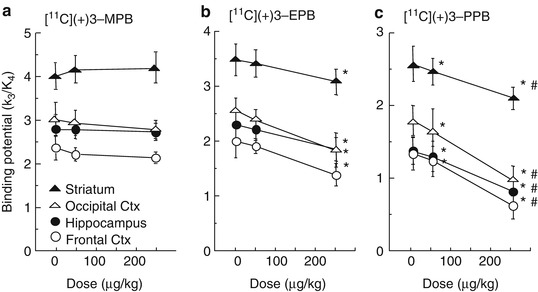
Fig. 16.6
Dose-dependent effects of Aricept on the binding potential (BP = k3/k4) of [11C](+)3-MPB (a), [11C](+)3-EPB (b), and [11C](+)3-PPB (c) in the cortical regions of the conscious monkey brain. PET scans were performed as shown in the legend of Fig. 16.4. Receptor binding of each labeled compound was analyzed based on a three-compartment model with metabolite-corrected plasma time-activity curve. Data are expressed as means ± SD for five animals per treatment condition. *P < 0.01 vs. Saline. # P < 0.01 vs. 50 μg/kg Aricept
16.3 Detection of Aging Effects on mAChR with [11C](+)3-MPB
Clinical PET studies with [11C]benztropine, [11C]4-MPB, and [11C]tropanyl benzilate quantitatively detected the neurological alterations of mAChR in aging (Dewey et al. 1990b; Suhara et al. 1993; Lee et al. 1996; Yoshida et al. 1998). The PET probes for mAChR listed above, however, showed relatively low uptake to the brain and also slow dissociation rates from receptor binding sites, which may limit estimation of the density of binding sites in vivo (Koeppe et al. 1994). So, we tried to assess the age-related changes in mAChR with [11C](+)3-MPB in the living brains of young (ca. 6 years old) and aged (ca. 20 years old) monkeys in the conscious state (Tsukada et al. 2001a). In addition, the effects of Aricept, a cognitive enhancer, on mAChR binding as well as cholinesterase inhibitor activity were evaluated with [11C](+)3-PPB and N-[11C]methyl-4-piperidyl acetate ([11C]MP4A) (Tsukada et al. 2004).
[11C]MP4A was labeled by N-methylation of its nor-compound with [11C]methyl iodide (Irie et al. 1996). The synthesis of [11C](+)3-MPB, PET data acquisition, cortical TAC, and plasma metabolic analyses were conducted as described in Sect. 16.2.1, and the synthesis of [11C](+)3-PPB and data analysis were done as shown in Sect. 16.2.2.
In aged monkeys, the time-activity curves of [11C](+)3-MPB in regions rich in muscarinic receptors peaked at earlier time points and showed faster elimination rates than those in young monkeys, while curves in the cerebellum showed no significant difference between young and aged animals. When analyzed using the three-compartment model with arterial input function, significant age-related alterations of the in vivo binding of [11C](+)3-MPB were observed in the temporal and frontal cortices and the striatum (Fig. 16.7, P < 0.05 vs. young animals in each region). In contrast, the previous studies demonstrated when expressed relative to the cerebellum as assumed nonspecific and free tracer reference region, age-related reduction of mAChR was observed in almost all cerebral regions in humans as measured by [11C]benztropine (Dewey et al. 1990b) and [11C]4-MPB (Suhara et al. 1993; Yoshida et al. 1998). It should be noted that there were trends toward reduced cerebral mAChR binding and toward elevated cerebellar binding with aging in humans as measured by a kinetic analysis method using [11C]tropanyl benzilate in conjunction with arterial blood sampling (Lee et al. 1996). Although we cannot exclude species differences (monkeys vs. humans) and of differences in labeled compounds used for analysis, the combination of opposing cortical and cerebellar changes of receptor binding with aging may have led to the previous conclusion that the loss of mAChR was more substantial than that reported here.
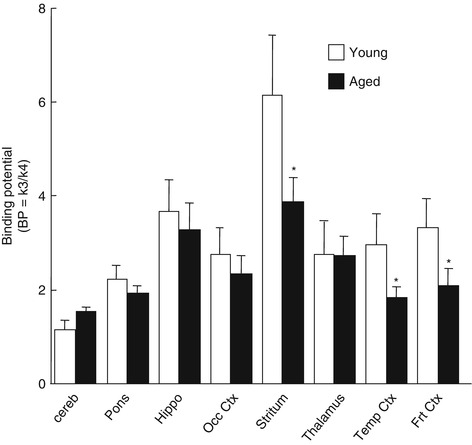
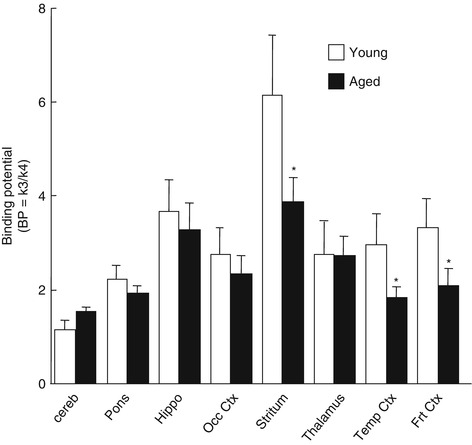
Fig. 16.7
Age-related changes in cerebral muscarinic cholinergic receptor binding in vivo. PET scans were performed as shown in the legend of Fig. 16.4. Receptor binding of each labeled compound was analyzed based on a three-compartment model with metabolite-corrected plasma time-activity curve. Data represent means ± SD for seven animals per group. *P < 0.05 vs. young animals
Furthermore, we evaluated the effects of Aricept, a cognitive enhancer with AChE inhibition, on the central cholinergic neuronal system in aged monkeys. The degree of AChE inhibition by Aricept was assayed by [11C]MP4A as well as a microdialysis method. The effect of increased acetylcholine on mAChR binding was demonstrated by [11C](+)3-PPB, which was sensitive to synaptic acetylcholine levels (Nishiyama et al. 2001). In aged monkeys, when Aricept was administered at the doses of 50 and 250 μg/kg, the k3 value of [11C]MP4A in the neocortical regions decreased in a dose-dependent manner, suggesting the suppression of AChE activity. The suppression of AChE activity by Aricept in the cortex was also confirmed by microdialysis analysis. The mAChR binding evaluated with [11C](+)3-PPB showed age-dependent reduction in all regions as shown with [11C](+)3-MPB previously, and Aricept at a dose of 250 μg/kg resulted in a significant reduction in BP of [11C](+)3-PPB in all regions assayed. Aged monkeys showed impaired working memory performance compared to young monkeys, and the impaired performance was partly improved by the administration of Aricept, due to the facilitation of the cholinergic neuronal system by AChE inhibition (Data not shown). These results demonstrated that the PET imaging technique with specific labeled compounds in combination with microdialysis and a behavioral cognition task could be a useful method to clarify the mechanism of drugs in the living brains of experimental animals.
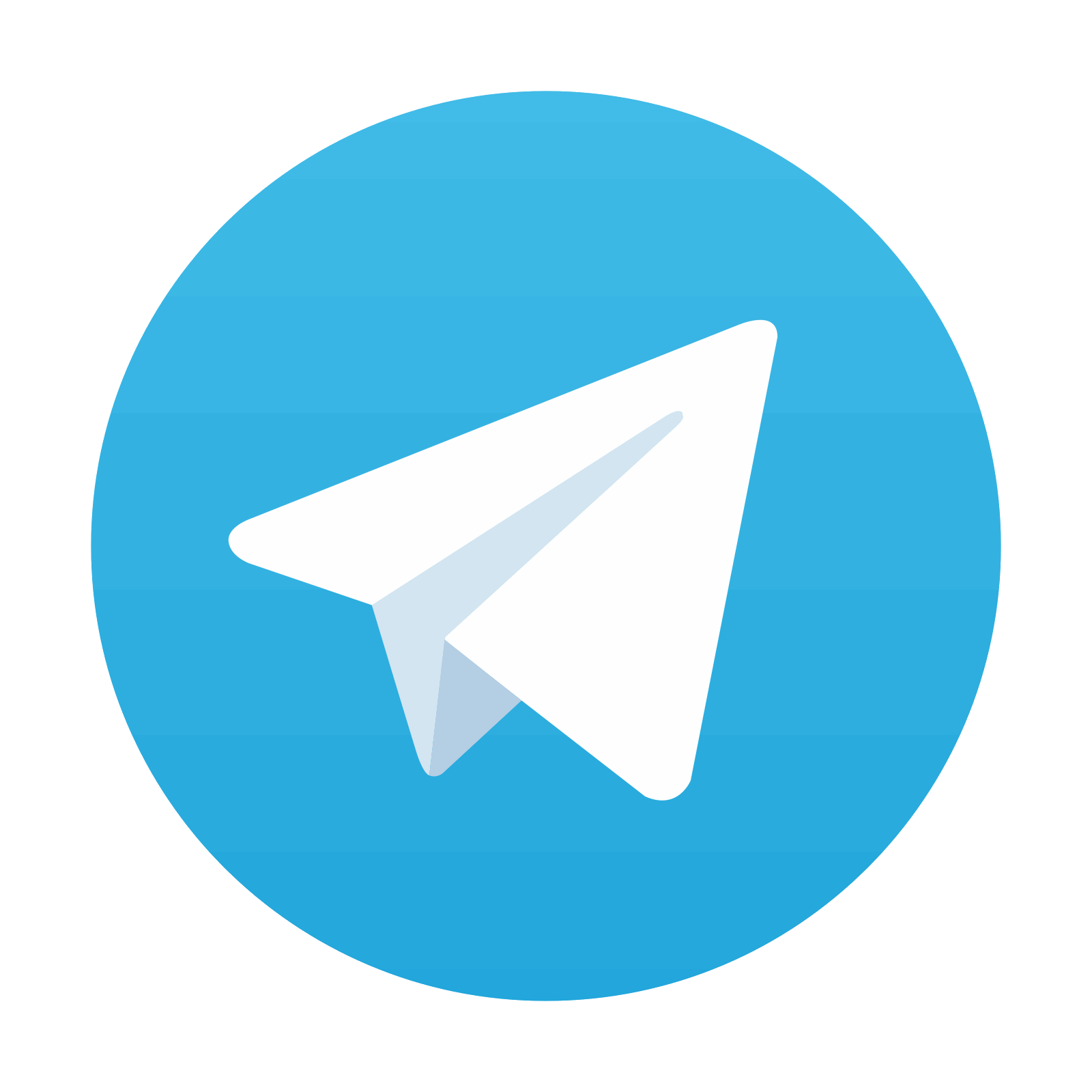
Stay updated, free articles. Join our Telegram channel

Full access? Get Clinical Tree
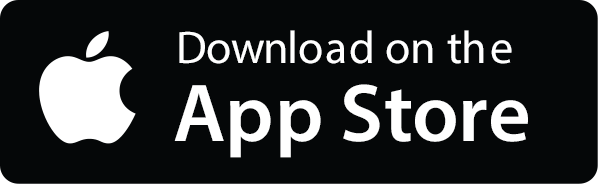
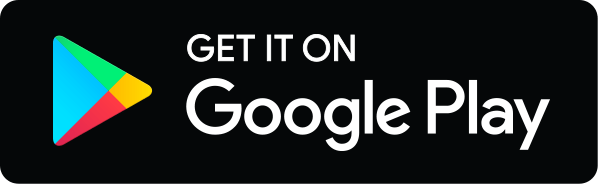