Fig. 8.1
Beta amyloid (Aß) processes from soluble amyloid precursor protein (s-APP) to different forms of Aß with their possible interaction with the nerve terminal (synapse)
8.2 Molecular Imaging
The landscape of AD research has significantly changed during recent years, moving away from studies in autopsy brain tissue and evaluation of clinical trials with quite insensitive cognitive scales, and toward the use of structural and molecular imaging and biomarkers in cerebrospinal fluid (CSF), and increasing the understanding of the pathological cascade of events in AD. The introduction of amyloid PET imaging with radiotracers such as 11C-Pittsburgh compound B (PIB) (Klunk et al. 2004) has provided new and valuable insight into the dynamic processes and time course of deposition of fibrillar Aß in the brain from preclinical through clinical stages of AD. Several different 18F-labeled amyloid PET tracers have been tested, and one, 18F-florbetapir, has been recently approved by the US Food and Drug Administration (FDA) and the European Medicine Agency (EMA) for clinical assessment of cognitive problems. Recently also 18F-flutemetamol was approved by FDA. The new molecular imaging techniques have provided opportunities to develop diagnostic biomarkers for early detection at preclinical stages as well as for monitoring effects of drug therapy. Incorporation of biomarkers into the standardized clinical diagnosis of AD is recommended (Dubois et al. 2007, 2010; Albert et al. 2011). It is now possible to recognize different stages of AD in affected populations, such as “asymptomatic AD,” “prodromal AD,” “mild cognitive impairment caused by AD (MCI/AD),” and “clinical AD” stages (Dubois et al. 2010; Albert et al. 2011; McKhann et al. 2011; Sperling et al. 2011).
8.3 Amyloid PET Tracers
8.3.1 Different Chemical Classes
After testing some radiolabeled amyloid antibodies (Friedland et al. 1994) to peptide fragments (Wengenack et al. 2000) that failed to label brain Aß in vivo, the small molecule strategy emanating from amyloid dyes such as Congo red and thioflavine was more fruitful (Nordberg 2004). 18F-FFDNP (Shoghi-Jadid et al. 2002) and 11C-PIB (Klunk et al. 2004) were the two first PET Aß tracers studied in AD patients. The compounds differ in chemical structure (Fig. 8.2) as well as in the binding patterns in the brain. Increased binding of 18F-FDDNP was observed in the temporal, parietal, and frontal regions of AD brain compared with controls (Shoghi-Jadid et al. 2002). 11C-PIB showed higher binding in the frontal, parietal, temporal, occipital, and striatal brain regions compared with controls (Klunk et al. 2004). The difference in binding between AD brains and control brains was significantly higher for 11C-PIB compared with 18F-FDDNP (Klunk et al. 2004; Shoghi-Jadid et al. 2002). On the other hand, 18F-FDDNP was found to also bind to tau proteins in the brain (Small et al. 2006). 11C-PIB has so far been the most explored and most used amyloid PET tracer. As illustrated in Fig. 8.2, several Aß PET tracers have now been developed belonging to different chemical classes including thioflavine (11C-PIB, 18F-flutemetamol, 11C-AZD2184), benzoxazole (11C-BF227,18F-BF227), stilbene (18F-FDDNP), naphthalenes (11C-SB13, 18F-AV-1 (florbetaben), 18F-AV-45 (florbetapir)), and benzofuran (18F-NAV-4694; 18F-AZD-4694). 18F-labeled compounds have the advantage that they do not require a cyclotron on site because of the longer half-time of 18F (110 min) compared with 11C (20 min). 18F-labeled compounds also provide the possibility of a short scanning time of 15–20 min. Generally, 18F amyloid tracers show a greater binding to white matter compared with 11C-PIB.
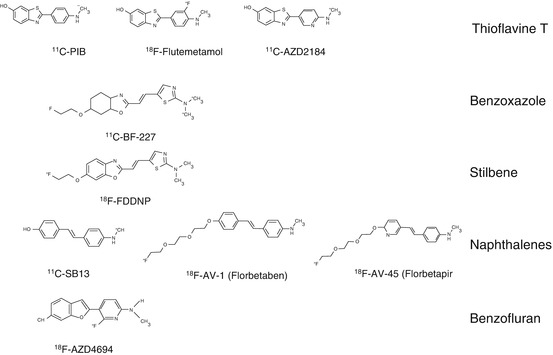
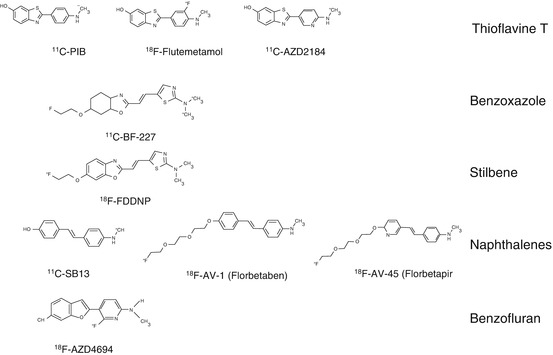
Fig. 8.2
Different PET amyloid tracers and their chemical structures
8.3.2 Multiple Binding Sites
Two binding sites have been observed in autopsy human brain tissue for 11C-PIB with a high-affinity site in the nanomolar range in addition to a low-affinity binding site (Klunk et al. 2004). High-affinity binding sites in the nanomolar range have also been reported in in vitro binding studies in human brain for AV-45 (florbetapir) (Choi et al. 2009) as well as AV-1 (florbetaben) (Fodero-Tavoletti et al. 2012). When comparing the in vitro binding properties of several of the PET amyloid tracers, a multitracer binding-site model could be postulated where PIB, flumetamol, and florbetaben all show nanomolar affinity for a major high-affinity site 1, whereas BF227 shows affinity mainly to binding sites 3 and FDNNP, with only some binding to site 2 (Ni et al. 2013). Different chemical structures may offer binding to different parts of the Aß fibrils but also to other nonfibrillar Aß components. Whether the Aß tracer binding properties differ in brain tissues from different familial AD (FAD) brains compared with sporadic cases has not yet been shown, nor have possible differences between the different stages of AD been investigated. Consistent comparable data have been reported between in vivo 11C-PIB (Bacskai et al. 2007; Ikonomovic et al. 2008; Kadir et al. 2011),18F-florbetapir (Clark et al. 2012), 18F-flumetamol (Wolk et al. 2011), and brain histopathology. There are, however, few studies in which different forms of Aß were quantified with PCR or similar methods and additional research is needed to study the similarities/dissimilarities between in vivo Aß scanning and in vitro autopsy studies.
8.4 Amyloid Imaging in AD and MCI
Representative PET images with 11C-PIB overlaid on magnetic resonance imaging (MRI) images for patients with mild AD are shown in Fig. 8.3. The high retention of 11C-PIB observed in the frontal cortex, parietal cortex, and temporal cortex, with sparing of the cerebellum, follows the deposition of Aß as described earlier by Braak and Braak (1991) from autopsy histopathological studies. The first 11C-PIB scan study, in a cohort of 15 AD patients, convincingly showed a significantly higher 11C-PIB retention in both cortical and subcortical brain regions in comparison with control subjects (Klunk et al. 2004), since then, numerous PET studies with 11C-PIB have verified the findings (for reviews see (Nordberg et al. 2010; Cohen et al. 2012; Rowe and Villemagne 2013)). A large multicenter study on 238 subjects scanned with 11C-PIB at five AD centers in Europe demonstrated the robustness of this technique in measuring Aß deposition in the brain (Nordberg et al. 2013). A high correlation between 11C-PIB imaging and different 18F-amyloid PET tracers (florbetapir, flumetamol, florbetaben, and NAV-4694 (AZD-4694)) has been found (for review, see (Johnson et al. 2013)).
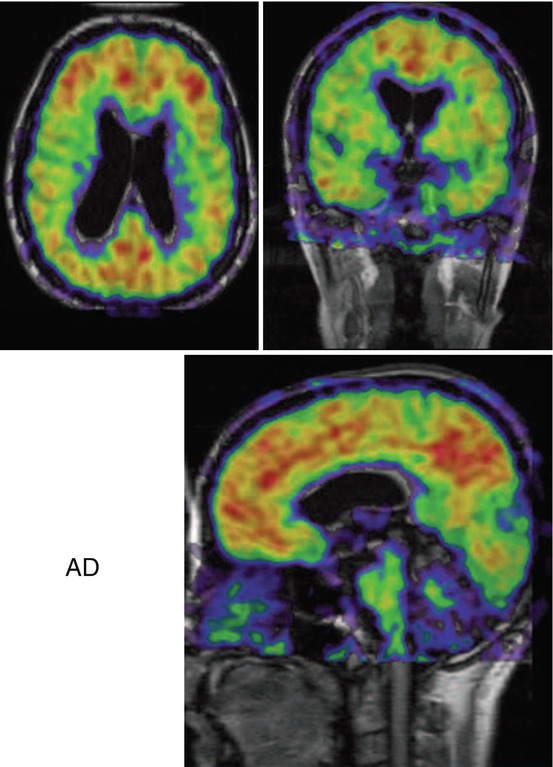
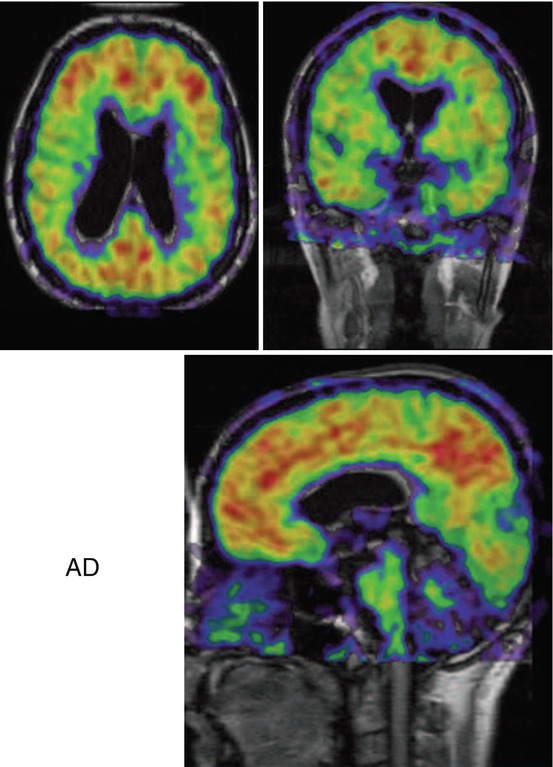
Fig. 8.3
11C-Pittsburg compound B (11C-PIB) retention in brains of Alzheimer patients. Color scale: red high, yellow medium, green medium-low, blue low (Photo: A. Nordberg, Karolinska Institutet)
Most interestingly, patients with mild cognitive impairment (MCI) have shown either high (PIB+) or low (PIB–) 11C-PIB retention (Forsberg et al. 2008; Okello et al. 2009; Wolk et al. 2009; Koivunen et al. 2011). Figure 8.4 shows 11C-PIB retention in multiple brain regions of patients with MCI. It is evident from the scans that high 11C-PIB retention is observed in large parts of the brain and is quite comparable to what is observed in AD patients. Because the cerebellum shows very low 11C-PIB retention, the cerebellum is often used as a reference region when expressing standard uptake values (SUV). Consistent data from several follow-up studies indicate that PIB+ MCI patients have a higher risk to convert to AD than PIB– MCI patients. When 64 MCI patients were longitudinally followed clinically for a mean duration of 28 ± 15 months, 67 % of PIB+ MCI patients converted to AD, whereas none of the PIB– MCI patients converted to AD (Nordberg et al. 2013). The observations support the possibility that, during memory assessments, the patients with MCI who are most likely to convert to AD in the future could be identified and therefore offered preventive or disease-modifying drug treatment.
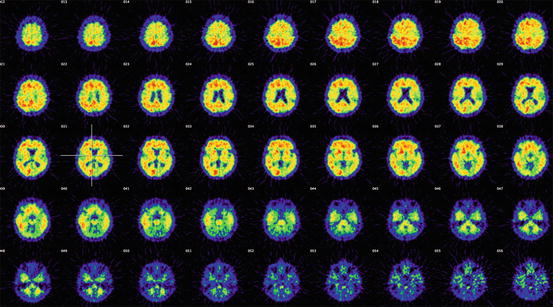
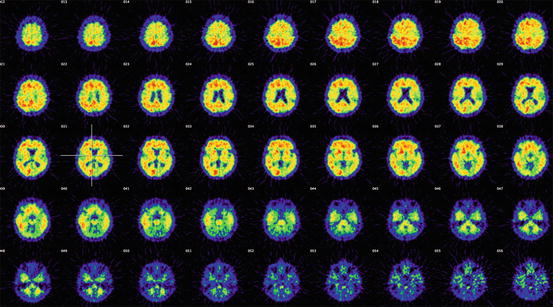
Fig. 8.4
11C-Pittsburg compound B (11C-PIB) retention in 45 transverse sections of the brain of patients with mild cognitive impairment (MCI) who later converted to AD. The first section illustrates high cortical regions and the last is on the level of the cerebellum (Photo: A. Nordberg, Karolinska institutet)
8.5 Longitudinal Changes in Amyloid Deposition in AD
A 2-year follow-up study in 15 AD patients showed unchanged 11C-PIB retention in the brain while declines in cerebral glucose metabolism (assessed by 18F-fluorodeoxyglucose (18F-FDG) uptake) and cognition (Engler et al. 2006) were observed; this study was followed by a 5-year follow-up study in the same cohort of AD patients (Kadir and Nordberg 2010). A discussion of whether there is a continuous increase in fibrillar Aß with the progression of AD has been ongoing in the literature. Although imaging studies in placebo-treated AD patients in immunization trials showed increases in amyloid (Rinne et al. 2010; Ostrowitzki et al. 2012), several studies based on larger numbers of subjects suggest an Aß plateau in AD (Jack et al. 2013b; Villemagne et al. 2013). Patients with mild MCI who are PIB+ show longitudinal increases in Aß (Kadir et al. 2012). From data generated from 18-month follow-up PIB PET studies in healthy subjects, patients with MCI, and AD patients, Villemagne et al. (2013) estimated that it takes 19 years to reach the Aß levels observed during the clinical phase of AD. Recent crosssectional PET imaging studies in subjects from families with autosomal dominant inherited AD have shown high fibrillar Aß deposition up to 15 years before the expected onset of symptoms (Batesman et al. 2012; Morris et al. 2012; Fleisher et al. 2012).
8.6 Correlation of Amyloid, Cerebral Glucose Metabolism, ApoE Genotype, and Cognition
The deposition of fibrillar Aß starts earlier in the course of AD in comparison with the decline in cerebral glucose metabolism as measured by 18F-FDG uptake (Nordberg et al. 2010; Jack et al. 2013a). A negative correlation has been observed between cerebral glucose metabolism and 11C-PIB retention in brain regions of AD patients and control patients in the parietal and temporal cortices but not in the frontal cortex (Klunk et al. 2004; Engler et al. 2006). 11C-PIB PET seems to detect prodromal AD at an earlier disease stage and better separates between MCI subtypes than 18F-FDG uptake (Forsberg et al. 2008). The decline in cerebral glucose metabolism seems to be more regionally specific compared with the increased 11C-PIB retention in large areas of the AD brain (Engler et al. 2006; Forsberg et al. 2010). Higher fibrillar Aß deposition has been observed in brains of patients with MCI and AD who have the ApoE E4 gene carrier compared with those who have the ApoE3 gene carrier, as measured by 11C-PIB and 18F-florbetapir (Drzezga et al. 2009; Fleisher et al. 2013; Nordberg et al. 2013). Correlation between Aß PET imaging results and cognition has been observed in patients with MCI as well as in control subjects (Pike et al. 2007; Forsberg et al. 2008; Kawas et al. 2013; Rosenberg et al. 2013); but also in AD patients when analyzing regional Aß load and episodic memory (Engler et al. 2006; Chetelat et al. 2012).
8.7 Amyloid Deposition in Healthy Elderly
An increasing Aß load has been measured by PET in cognitively healthy subjects, with the measured load ranging between 10 % (Nordberg et al. 2013; Mintun et al. 2006), 22 % (Pike et al. 2007), 33 % (Rowe et al. 2010), and 55 % (Mathis et al. 2013). The increase in fibrillar Aß as measured by amyloid PET tracers appears to increase with age, and it has recently been estimated that approximately 20 years is needed to reach clinical symptomatology (Villemagne et al. 2013). The presence of brain injury measured by MRI and PET FDG in cognitively healthy elderly subjects assigned for preclinical AD has been reported to be unrelated to the presence of Aß (Knopman et al. 2012; Wirth et al. 2013). This lack of correlation may change the view of the role of Aß in AD (Chetelat 2013).
8.8 Amyloid Imaging in Other Conditions
Amyloid imaging may help in differentiating AD from other forms of dementia. For example, in most patients with FTLD, PET Aß scans are negative (Raboinovici et al. 2007; Engler et al. 2008; Drzezga et al. 2008; Laforce and Rabinovici 2011). In corticobasal syndrome, PIB-positive patients showed greater visuospatial deficits and repetition impairment than PIB-negative patients (Burrell et al. 2013). The presence of Aß, as assessed by PET imaging, has not been found in brains of Parkinson patients who have no cognitive impairment (Johansson et al. 2008). Patients with Parkinson MCI and amyloid show declines in cognition compared with patients with no Aß in brain observed in imaging studies (Gomperts et al. 2012). High fibrillar Aß levels are often observed in the brains of patients with DLB (Rowe et al. 2007; Edison et al. 2008; Maetzler et al. 2009; Shimada et al. 2013), and it is therefore difficult to discriminate between AD and DLB by Aß tracer PET imaging. High 11C-PIB retention has been observed in patients with cerebral Aß angiopathy, and the regional pattern is different from that observed in AD (Johnson et al. 2007).
8.9 Tau PET Imaging
Accumulation of tau is known to occur in several neurodegenerative diseases, including AD, FTLD, Down’s syndrome, cortical basal degeneration, progressive nuclear palsy, FTLD with Parkinson dementia, multiple system atrophy, and prion disease. Although tau is present in several diseases, there are probably variations in the presence of the different tau isoforms. Histopathologically, the presence of tau in the brain is demonstrated as NFTs, as paired helical filaments as well as dystrophic neurites in senile plaques. The number of NFTs in autopsy brain tissue has been reported to negatively correlate with cognitive function before death. 18F-FDDNP, which was introduced as an Aß PET tracer, was demonstrated to bind to NFTs (Agdeppa et al. 2001). A correlation between 18F-FDDNP binding and episodic memory was reported in AD and MCI patients (Tolboom et al. 2009). Presently, other candidates for PET tracers, such as 18F-THK523, 18F-5105, 18F-5117, and 18F-T-807, have been suggested (Fodero-Tavoletti et al. 2011; Zhang et al. 2012; Harada et al. 2013; Chien et al. 2013; Chun-Fang et al. 2013; Okamura et al. 2013), and clinical studies of these compounds are in progress. Harada et al. (2013) assessed the in vitro binding profile to tau and Aß fibrils and compared the binding of 18F-THK523 with the Aß ligands 18F-BF-227, 18F-FDDNP, and 3H-PIB. They observed that 18F-THK523 showed a higher affinity for binding tau fibrils compared with Aß fibrils than the other three PET tracers investigated (Harada et al. 2013). Okamura et al. (2013) demonstrated a higher in vitro binding affinity for THK-5105 and THK-5117 compared with THK-523. In autoradiographical studies in autopsy AD brain tissue, they also showed that the radiotracers bound preferentially to NFTs and that the distribution was different from the 11C-PIB binding (Okamura et al. 2013). 18F-T-807 has also been shown to bind with high affinity to human brain tissue NFTs (Zhang et al. 2012). In vivo PET studies showed a higher retention of 18F-T-807 in the temporal cortex and hippocampus of AD patients compared with controls (Chien et al. 2013). Because the number of NFTs seems to correlate with the degree of dementia, tau PET tracers might be more useful for following the progression of AD and for evaluating drug efficacy than for being a very early diagnostic biomarker.
8.10 Conclusions
Molecular imaging techniques have increased the opportunities for studying the time course of pathophysiological processes in the brain during neurodegenerative disorders. Aß peptide deposition in plaques can measured by PET with different available Aß tracers. Different time courses of pathological changes have been observed when using imaging (Fig. 8.5). The accumulation of fibrillar Aß in the brain starts much earlier than the decline in cerebral glucose metabolism. This seems to be similar for early astrocytosis in the brain. Changes in cognition, neurotransmitter impairment, and brain atrophy seem to be closely related temporally. The introduction of new PET tracers for visualizing different forms of Aß peptides (including Aß oligomers), different forms of tau (including tau oligomers), and other not-yet studied peptides, such as α-synuclein, will open up new possibilities for the further understanding of proteinopathies.
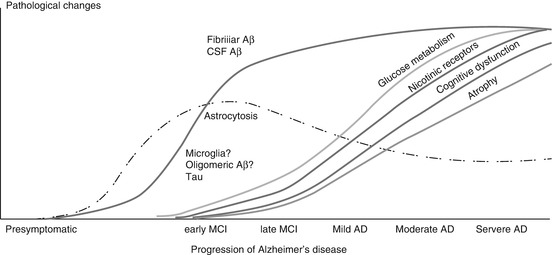
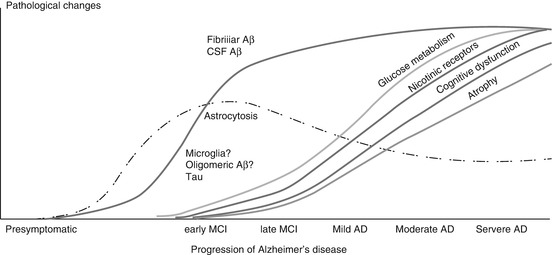
Fig. 8.5
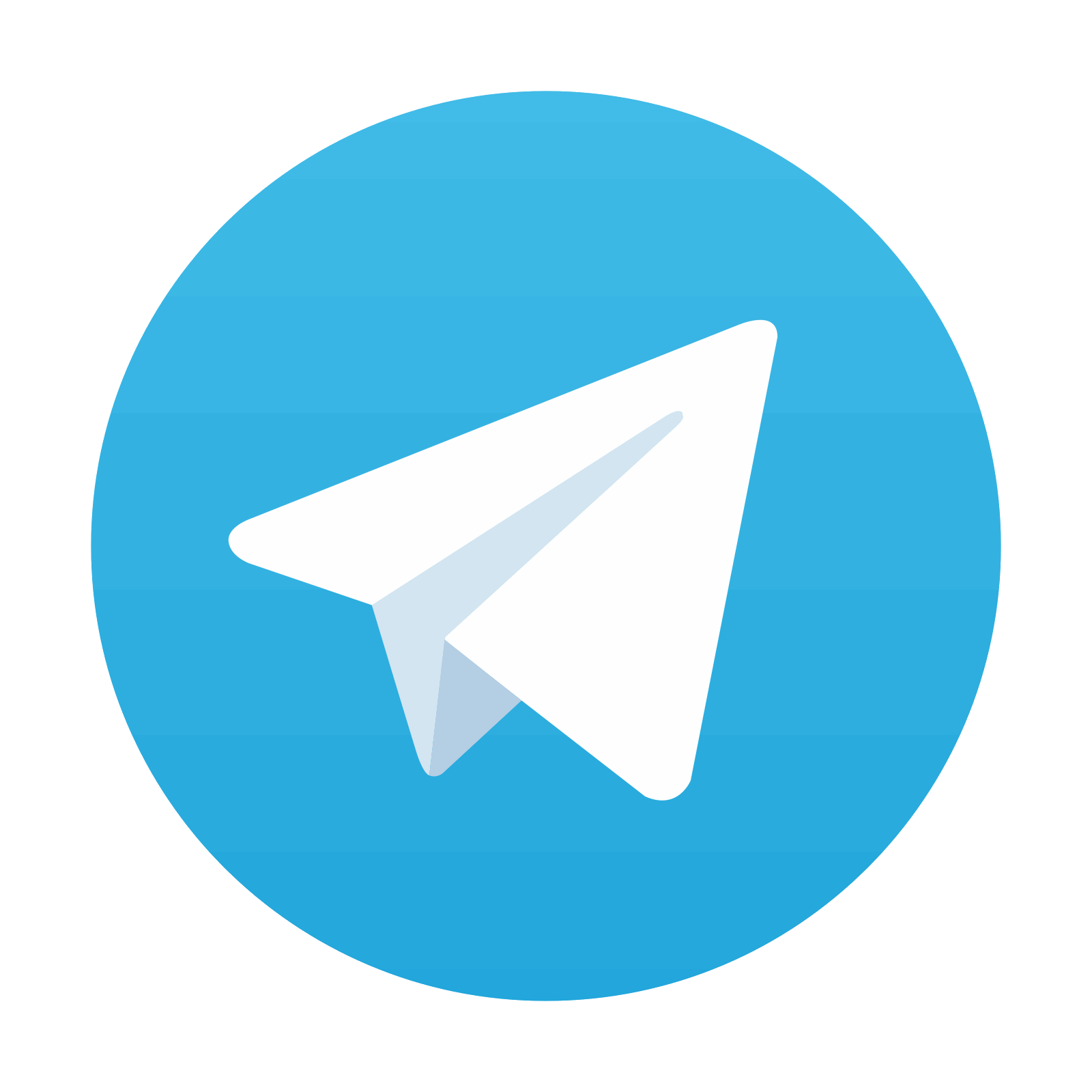
Time course of pathological and functional imaging markers during progression of Alzheimer’s disease. Aß amyloid beta, AD Alzheimer’s disease, CSF cerebral spinal fluid, MCI mild cognitive impairment (From Nordberg 2011)
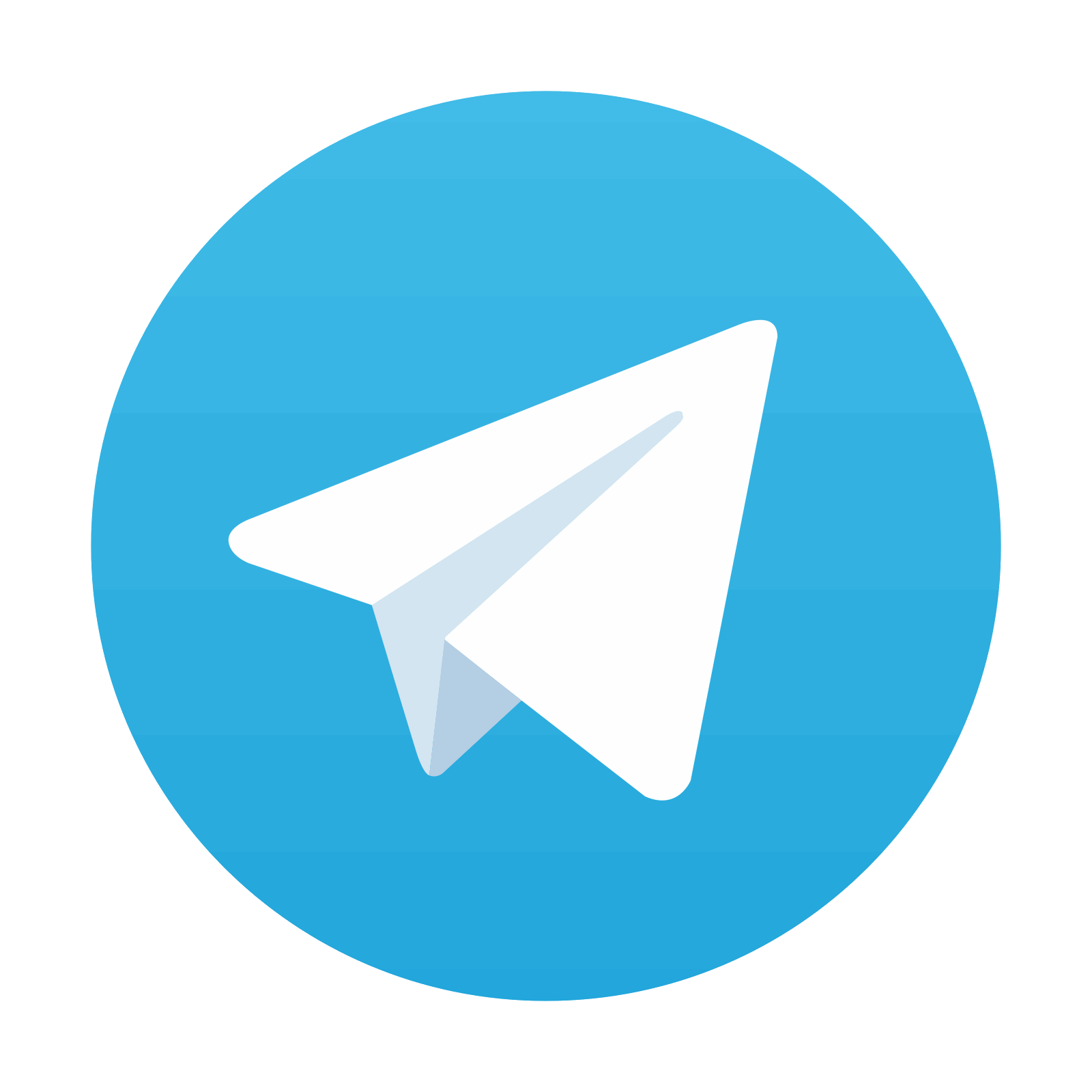
Stay updated, free articles. Join our Telegram channel

Full access? Get Clinical Tree
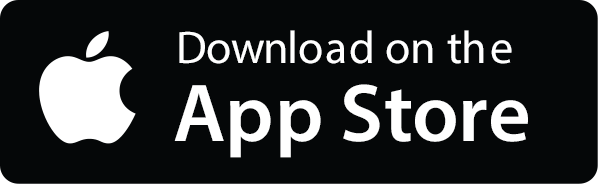
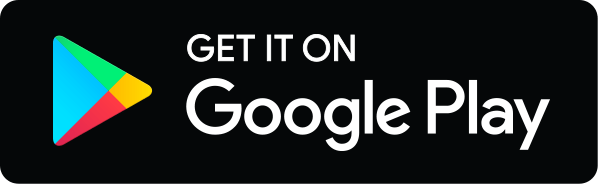
