PET/MR imaging is an integrated imaging system that combines the high soft tissue resolution of MR imaging with the quantitative data obtained from PET into a single system. It is a relatively new imaging technique but with potential clinical use in pediatric oncologic and nononcologic processes in additional to its role in research. It is particularly relevant in pediatric patients due to reduced radiation burden compared with PET/CT and the ability to obtain exquisite functional and anatomic imaging in a single imaging session, thereby reducing the number of anesthesia/sedations. This review article focuses on the current as well as future applications of PET/MR imaging in pediatric imaging, including both oncologic and nononcologic indications.
Key points
- •
PET/MR imaging is particularly relevant in pediatrics due to reduced radiation burden compared with PET/CT.
- •
PET/MR imaging is a highly promising imaging modality, although it presents many challenges that need to be addressed prior to routine use.
- •
Current PET/MR imaging use in pediatrics is primarily in oncology.
Introduction
Combined PET/MR imaging is an emerging hardware solution that integrates the advantages of high soft tissue contrast and exquisite anatomic characterization of MR imaging with the high sensitivity of and quantitative physiologic data obtained from PET into a single system. The first combined PET/MR imaging systems were installed in 2010, and since then there have been slowly and steadily increasing technological advancements and clinical adoption. Although the use of PET/MR imaging in the pediatric setting is still in its infancy, it is an attractive imaging technology that could result in radiation dose savings and also negate the need for multiple imaging studies and anesthetic exposures in children. This review article focuses on the current as well as future applications of PET/MR imaging in pediatric imaging, including both oncologic and nononcologic indications.
PET/MR imaging technique
Instrumentation
Broadly speaking, 2 different technical approaches to combined PET/MR imaging have been developed: sequential and concurrent or simultaneous PET/MR imaging.
Sequential PET/MR imaging
In sequential PET/MR imaging, the PET and MR imaging scanners are positioned in-line but separate, with the same patient-transfer tabletop moving between the 2 imaging gantries. The sequential approach is less challenging technically than simultaneous PET/MR imaging, because the distance between the scanners reduces interference of PET photomultiplier tube (PMT) function with magnetic fields from MR imaging and vice versa.
Simultaneous PET/MR imaging
Concurrent imaging with an integrated scanner necessitates addressing the space restrictions inside the MR imaging magnet bore and any possible interference between the 2 modalities. In the first prototypes of integrated scanners, the PMTs needed to be physically separated from the magnetic field of MR imaging using long cables. More recently, the PMTs have been replaced with semiconductor detectors, including avalanche photodiode detectors or silicon photomultipliers that are not sensitive to magnetic fields. Fully integrated simultaneous PET/MR imaging scanning is particularly pertinent to pediatric imaging in decreasing overall scanning time, given limitations of patient compliance and the need for general anesthesia/sedation. The remainder of this review focuses on this type of scanner, which is the preferred and more widely installed scanner type. All new PET/MR imaging systems are now of the simultaneous type.
Workflow, Technical/Protocol Considerations, and Challenges
Workflow considerations
Smooth operation of a PET/MR imaging program requires a great deal of planning, coordination, cross-modality training, and multidisciplinary effort involving pediatric radiologists, nuclear medicine physicians, radiologic technologists, nuclear medicine technologists, nursing staff, and referring physicians. The indications for PET/MR imaging should be established case by case but based on a few preinstituted guidelines and in specific patients and disease groups. Proper patient preparation is key to successful scanning, including choosing the appropriate tracer and determining the need for sedation. PET-related preparation, including fasting prior to injection of F-18 Fluorodeoxyglucose ( 18 F-FDG) and measures for suppression of physiologic brown adipose tissue uptake, are similar to those for PET/CT. Additionally, patients are screened with an MR safety questionnaire, similar to conventional MR imaging examination screening.
Technical/protocol considerations
Although numerous (and numerous combinations of) acquisition protocols are currently possible, most pediatric whole-body PET/MR imaging protocols are similar in their fundamental components. A sample protocol with standard MR imaging pulse sequences is shown in Fig. 1 and listed in Tables 1 and 2 . A whole-body (or torso) examination usually includes whole-body (or skull base to thighs) PET and MR acquisitions, with typically MR-based attenuation correction sequences and fat-suppressed T2-weighted and/or inversion recovery sequences acquired at each bed position (usually 3–4 minutes at each position). The most commonly used method for attenuation correction is segmentation based, with a double-echo chemical shift gradient-echo sequence (Dixon technique) that provides separate fat and water images that are then segmented into 4 tissue compartments: soft tissue, fat, lung, and background air. Atlas-based attenuation correction methods may be inaccurate for use in pediatric patients because they are based on adult atlases. Diffusion-weighted imaging (DWI) also can be performed as a part of the whole-body protocol. Additionally, dedicated imaging of a specific body part may be performed based on the indication, including administration of gadolinium-based contrast where needed. For brain imaging, anatomic sequences, such as T2-weighted fluid-attenuated inversion recovery (FLAIR) and/or magnetization-prepared 3-D volumetric T1 gradient-echo sequences, are performed in addition to those for attenuation correction with a typical total acquisition time of 20 minutes ( Table 3 ). The administered activity of 18 F-FDG for 18 F-FDG PET/MR imaging is currently the same as for PET/CT and is typically based on North American consensus guidelines for pediatric patients, with a suggested activity of 0.10 mCi/kg to 0.14 mCi/kg (3.7–5.2 MBq/kg) for body PETs and 0.10 mCi/kg (3.7 MBq/kg) for brain PETs. When complete brain or body protocol MR imaging is simultaneously performed, this potentially allows for a further reduction in administered radiotracer dose because of the longer MR imaging acquisition time per bed position compared with PET/CT.
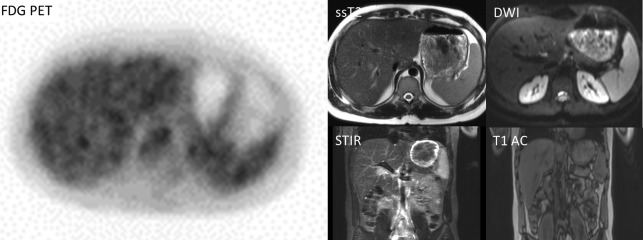
Sequence (Siemens [Siemens Healthcare, Erlangen, Germany] Biograph mMR) | Plane | Repetition Time (ms) | Echo Time (ms) | Matrix | Comments |
---|---|---|---|---|---|
T1 in/opposed phase attenuation correction, Dixon volumetric interpolated breath-hold examination | Coronal | 3.6 | 1.2 | 192 × 121 | |
T2 single-shot (Half-Fourier acquisition single-shot turbo spin-echo) | Axial | 1600 | 95 | 320 × 260 | |
DWI | Axial | 11,000 | 70 | 160 × 120 | B = 50,400,800 |
Short tau inversion recovery | Coronal | 4000 | 48 | 320 × 192 | Respiratory triggered for chest and abdomen |
Sequence (GE Signa, GE, Chicago, Illinois) | Plane | Repetition Time (ms) | Echo Time (ms) | Matrix | Comments | |
---|---|---|---|---|---|---|
T1 Dixon attenuation correction | Axial | 4 | 1.6 | 256 × 128 | ||
3-D T1 LAVA-Flex | Axial | 4 | Minimum | 288 × 224 | Fusion in 3 planes | |
DWI | Axial | 5500 | Minimum | 160 × 128 | B = 150,400,800 | |
Short tau inversion recovery | Coronal | 2000–6500 | 68 | 320 × 224 | Respiratory navigator for chest and abdomen if good waveform | |
T2 fast spin-echo, fat suppressed | Axial | 8000–12,000 | 80 | 320 × 224 | Add on at sites of interest |
Sequence | Plane | Resolution | Notes |
---|---|---|---|
3-D volumetric T1 with inversion preparation | Sagittal (with reformats in other planes) | 0.9–1.2 mm 3 isotropic | GE: BRAVO Siemens: MPRAGE |
3-D volumetric T2 with variable flip angle | Sagittal (with reformats in other planes) | 1.0–1.2 mm 3 isotropic | GE: CUBE Siemens: SPACE Can be done 2-D in other planes as well |
FLAIR | Axial (or coronal for epilepsy) | 3–5 mm thickness 256 × 192 in-plane matrix | Can be done 3-D as well |
Arterial spin labeling perfusion | Axial (whole brain) | GE: 512 sampling along 8 spiral arms, 3–4 mm slice thickness Siemens: 1.6 m × 1.6 mm × 4 mm | GE: 3-D spiral pseudocontinuous arterial spin labeling Siemens: 3D GRASE pulsed arterial spin labeling Inversion time: 1900–2050 ms |
DWI | Axial | 3–5 mm thickness 128 × 128 in-plane matrix acquisition | B = 0, 1000—can be fast spin-echo DWI or multishot segmented k-space if needed, albeit with increased scan time—may substitute diffusion tensor imaging acquisition |
Technical challenges
Attenuation correction of the PET scan emission data is a prerequisite for accurate quantification of PET and is more challenging with PET/MR imaging due to the lack of bone density information, truncation of MR imaging–based image information along the patient’s arms, and the presence of additional attenuating hardware components, such as radiofrequency coils. There are newer methods of compensating gradient nonlinearities and extending the MR imaging field of view to include the arms and, therefore, decrease PET attenuation correction bias. Imaging of lung parenchyma is difficult with MR imaging due to low proton density, rapid loss of signal because of field inhomogeneity, and respiratory motion. For these reasons, as well as the inferior spatial resolution of MR imaging compared with CT, it is challenging to detect small pulmonary nodules with PET/MR imaging, and chest CT maintains in important role in thoracic staging of pediatric malignancies.
Data processing, display, and storage
Various software packages are offered by the PET/MR imaging system manufacturers and other third-party vendors for hybrid image viewing and evaluation. More work is likely needed to optimize the nuclear medicine workstations to support viewing of PET/MR imaging with various MR imaging acquisitions, including functional data, such as DWI and perfusion-weighted imaging. Despite smaller image matrix size, PET/MR imaging has larger data storage requirements in comparison to PET/CT because more numerous sequences are obtained. PET/MR imaging examinations have typical size requirements ranging from 500 MB to 2 GB.
Radiation Considerations
PET/MR imaging provides significant radiation dose savings compared with PET/CT. In addition to the dose reduction from eliminating CT imaging, the longer acquisition time per bed position associated with PET/MR imaging potentially allows lower administered radiopharmaceutical doses. Depending on the acquisition parameters of the CT scan (diagnostic-quality CT vs localization/attenuation correction CT) accompanying the PET/CT, dose reductions of up to 73% have been reported when performing PET/MR imaging instead of PET/CT.
Imaging evaluation
Image Interpretation Considerations
Reading and interpreting PET/MR imaging data are complex tasks requiring integration of information obtained from multiple MR imaging sequences with the PET data, which necessitates a high level of expertise in both pediatric MR imaging and pediatric nuclear medicine, and is highly demanding on the readers. The images could be interpreted in a joint read-out session between nuclear medicine physicians and pediatric radiologists, or, alternatively, a single pediatric imager who is trained in both nuclear medicine and radiology could interpret them. The former method may be preferred, particularly in situations where complex organ-specific MR acquisition has been performed, and could follow the typical workflow of the department for reading dedicated organ-system MR imaging by subspecialty pediatric radiologists (eg, thoracic radiologists, abdominal radiologists, musculoskeletal radiologists, or neuroradiologists), and separate MR imaging and PET reports may be issued. In contrast, a whole-body PET/MR imaging examination performed without a dedicated diagnostic-quality organ-specific MR imaging is probably most efficiently interpreted by a single hybrid pediatric imager.
Image Pitfalls
Certain pitfalls related to MR-based attenuation correction have to be considered to avoid misinterpretation. Quantitative errors are observed in and around skeletal structures (eg, bone metastases and bone marrow) because bone attenuation is often neglected in segmentation-based MR attenuation correction. Additionally, segmentation artifacts also can occur around metal implants due to MR imaging susceptibility artifacts. Even though these artifacts have the potential to affect PET quantification (ie, standardized uptake values [SUVs]) and visual PET interpretation, likely no changes in diagnosis occur, especially when the attenuation maps are checked for artifacts and PET/MR imaging is read in conjunction with the non–attenuation-corrected PET. Truncation artifact that can be seen with PET/CT also may occur with PET/MR due to differences in field of view of PET and MR imaging ( Fig. 2 ). Important pearls and pitfalls in performance and interpretation of pediatric PET/MR imaging and its clinical applications are listed in Box 1 .
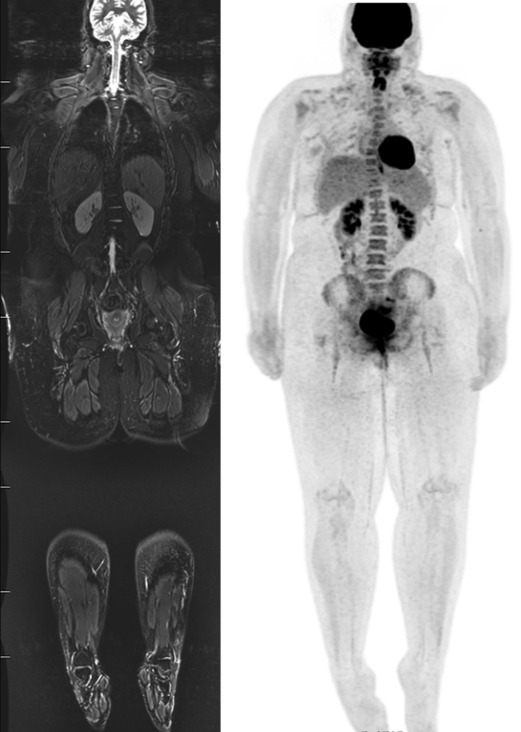
- •
The 2 types of PET/MR imaging scanners are sequential and simultaneous, and the latter is preferred and more widely installed.
- •
In integrated PET/MR imaging only a normal-bore (60-cm) MR imaging system is available.
- •
PET/MR imaging cannot be performed in patients who have contraindications to MR imaging.
- •
Fully integrated PET/MR imaging is particularly relevant in children in decreasing overall scanning time, given limited patient compliance and the need for anesthesia/sedation.
- •
PET/MR imaging protocols should maximize the time of simultaneous acquisition of MR and PET and need to be optimized to achieve the highest diagnostic accuracy.
- •
PET/MR imaging protocol preferably should not be longer than the equivalent PET/CT plus organ system/region-specific MR imaging for a given indication.
- •
Dose reductions of up to 73% have been reported when performing PET/MR imaging instead of PET/CT due to lack of the CT component.
- •
Decreasing the amount of PET tracer administered (due to longer imaging times in PET/MR imaging) could further reduce the radiation dose.
- •
It is highly recommended to assess the quality of the MR-based attenuation map (μ map) as an initial step in reading PET/MR imaging to assess for potential artifacts.
- •
Quantitative errors are observed within and around skeletal structures (eg, osseous metastases and bone marrow) because bone attenuation is usually neglected or inaccurately calculated.
- •
Time-of-flight technology in PET/MR imaging may significantly reduce metal artifacts.
- •
Segmentation artifacts can occur in MR-based attenuation correction that are mostly observed around metal implants causing MR susceptibility artifacts.
- •
Because of smaller size of young pediatric patients and resultant relatively larger FOV of the MR imaging portion of the scan, the arms that are by a patient’s side are imaged as a part of the attenuation correction sequences, which avoids attenuation correction errors.
- •
MR imaging information/data also can be used to improve PET reconstruction and data analysis. For instance, because MR imaging sequences could be obtained during breath-holds or using respiratory gating or, alternatively, used for motion correction. Therefore, PET data could be filtered and reconstructed with motion-free or motion-corrected data, with decrease in motion artifact.
- •
A majority of the basic system performance and quality-control tests for a clinical PET/MR imaging scanner typically are performed with standard MR imaging and PET phantoms.
- •
PET/MR imaging examinations have increased data storage size requirements in comparison to PET/CT ranging from 500 MB to 2 GB.
- •
Advanced functional MR imaging techniques, such as DWI, MR spectroscopy, and perfusion-weighted imaging, in conjunction with PET may further enhance disease detection and characterization.
- •
For 18 F-FDG examinations, physiologic brown fat uptake needs to be suppressed (by warming or pharmacologic means) to preserve image quality and diagnostic accuracy.
- •
Small lung nodules are challenging to detect on PET/MR imaging and, therefore, separate CT may need to be acquired in patients likely to have pulmonary metastases.
- •
For both bone and soft tissue sarcoma, PET/MR imaging does not have incremental value over MR imaging alone for the primary tumor but is beneficial in staging of nodal and distant metastases.
- •
18 F-FDG PET is useful in the assessment of primary as well as metastatic disease in neuroblastomas that are not mIBG avid or when clinical symptoms and/or conventional imaging modalities, including MR imaging, suggest more disease than the extent of mIBG uptake.
- •
18 F-FDG uptake by brain tumors correlates approximately with the grade of the tumor and, therefore, is used to image poorly differentiated tumors, such as high-grade gliomas.
- •
To reduce the effect of post-therapy inflammation, 18 F-FDG PET/MR imaging in assessment of brain tumors should be performed 3 months after end of treatment.
- •
For brain tumor imaging with 18 F-FDG PET, delayed imaging at 3 hours to 4 hours improves the tumor-to-brain uptake ratio.
- •
The main neurologic indication for pediatric PET/MR imaging is assisting presurgical localization of epileptogenic focus.
- •
18 F-FDG PET has a high sensitivity for detecting epileptogenic foci in the temporal lobe with lower sensitivity for extratemporal epilepsy.
- •
PET/MR imaging may have an important role in the identification of cortical dysplasia by detecting subtle changes on MR images that may initially be reported as normal.
- •
For accurate interpretation of 18 F-FDG PET for epilepsy, it is beneficial to monitor with electroencephalogram during the 18 F-FDG uptake phase. Seizures that occur during the uptake period may affect tracer distribution and degree of uptake in the epileptogenic foci.
- •
18 F-FDG is a sensitive agent but nonspecific in evaluation of infection/inflammation. Additionally, assessment for abnormal uptake in regions of normal physiologic distribution is challenging. Simultaneous 18 F-FDG PET/MR imaging can potentially increase the overall accuracy of diagnosis in this clinical setting.
Clinical applications
The major applications of PET/MR imaging in pediatrics are discussed in this section. The reader is also referred to Table 4 for a list of major clinical applications, the radiopharmaceuticals used, and pertinent imaging features and evaluation.
Clinical Applications | Major PET Radiopharmaceuticals | Imaging Assessment | |
---|---|---|---|
Oncologic | Lymphoma | 18 F-FDG |
|
Primary bone tumors | 18 F-FDG |
| |
Soft tissue sarcomas | 18 F-FDG |
| |
NF-1 | 18 F-FDG |
| |
Neuroendocrine tumors | 68 Ga-DOTATATE 18 F-FDG 18 F-DOPA 11 C-hydroxytryptophan |
| |
Neuroblastoma | 18 F-FDG PET 68 Ga-DOTATATE 11 C-hydroxyephedrine 11 C-epinephrine 18 F-fluorodopamine 18 F-DOPA |
| |
Primary brain tumors |
|
| |
Nononcologic | Epilepsy | 18 F-FDG |
|
| 18 F-FDG |
| |
| Metabolic imaging 18 F-FDG Perfusion imaging 83 Ru 13 N-ammonia 15 O-water |
|
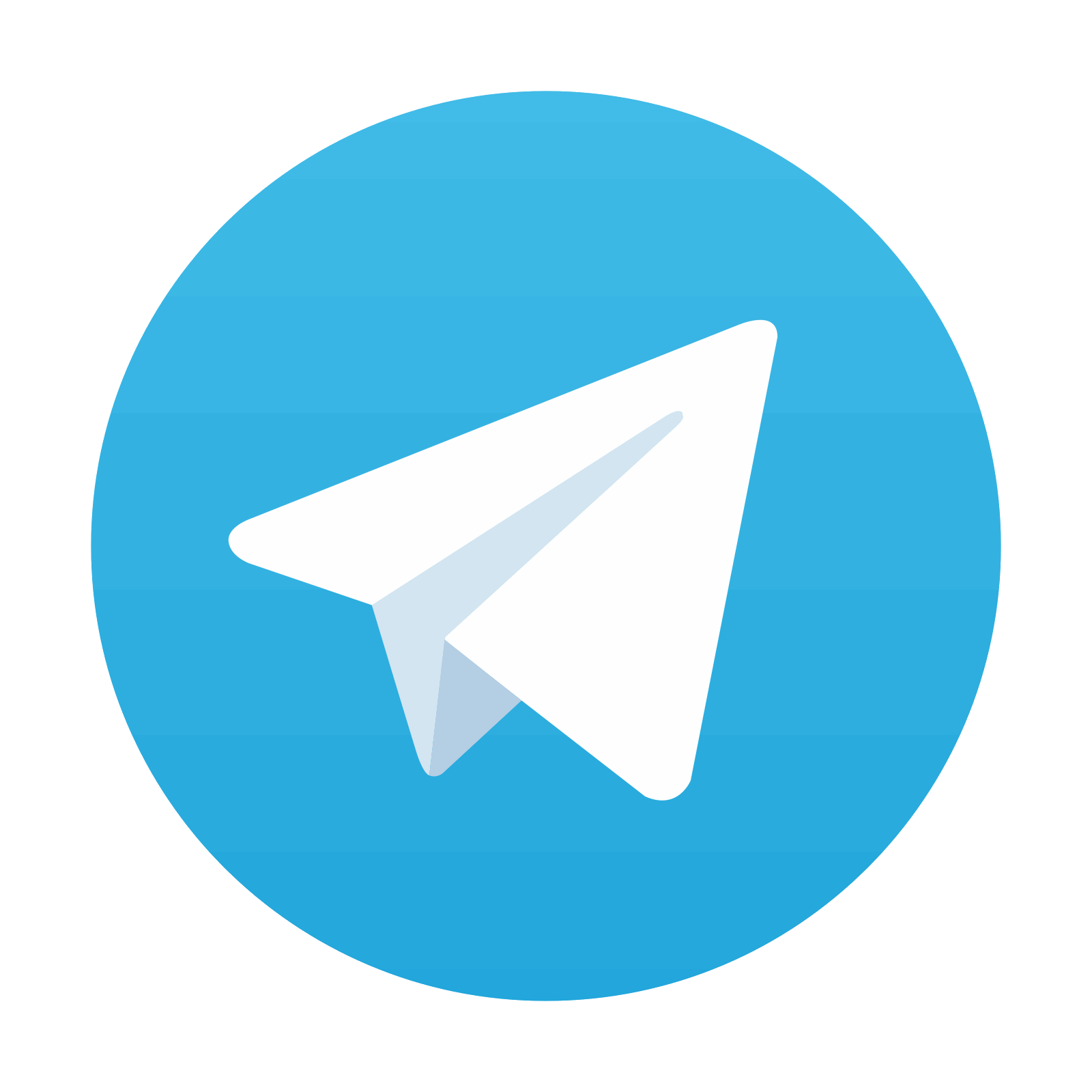
Stay updated, free articles. Join our Telegram channel

Full access? Get Clinical Tree
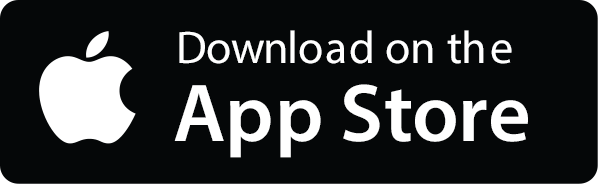
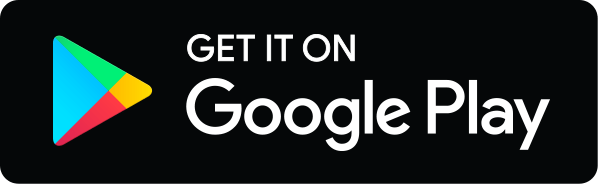
