Fig. 1
Functional magnetic resonance imaging brain activations with vibrotactile hand stimulation shown on anatomical images. The patient was investigated after complete median nerve transection of the right hand and immediate epineural end-to-end coaptation. Vibrotactile stimulation was separately applied to the left and right hands. (a) Representative anatomical slice illustrating the time course of maximum t-values in the primary somatosensory cortex (injured hand: blue voxels; healthy hand: red voxels). The graph illustrates the peak t value ratios of the injured/healthy hand (p < .01 uncorrected). Only top 150 t value voxels per brain volume are shown for display purposes and color bars indicate t-values. (b) Same illustration for frontoparietal cortex. (c) Proposed regeneration index calculated from graphs A and B (ratio primary cortex)/(ratio frontoparietal cortex) (Reproduced with permission from Rath et al. (2011)) (Color figure online)
6.2 Neuroplasticity After Nerve Repair in Brachial Plexus Lesions
Complete brachial plexus lesions lead to a total paresis of the affected arm resulting in a significant impairment in everyday life. Reinnervation of the affected musculocutaneous nerve via an intact C7 root or via the ipsilateral phrenic nerve can be performed to regain elbow flexion. In an fMRI study, Beisteiner et al. (2011) analyzed functional activation in three patients with complete brachial plexus lesions and in three healthy controls. Two patients underwent an end-to-side nerve reconstruction of the musculocutaneous nerve with the ipsilateral phrenic nerve and one patient received an end-to-end coaptation of the healthy contralateral C7 root with the musculocutaneous nerve. The advantage of the end-to-side technique is the preservation of the donor nerve function – that is, the control of the diaphragm persists via the modified phrenic nerve. However, after establishing an end-to-side connection, the phrenic nerve and its cortical projection area are required to perform a new double function: independent control of breathing and elbow flexion. In contrast, in the control patient (C7 – musculocutaneous connection) the phrenic nerve remained unchanged. FMRI tasks comprised elbow flexion of the healthy arm, elbow flexion of the diseased arm, forced abdominal inspiration, and foot flexion on the affected side. Clinical documentations such as electromyographic recording, chest radiography, and fluoroscopy of the thorax confirmed that end-to-side patients were able to control the diaphragm and the arm independently. In Fig. 2, functional imaging findings in patients and controls are summarized. Forced inspiration activated the diaphragm (phrenic nerve) area of the primary motor cortex bilaterally in all participants. In patients with end-to-side anastomoses, elbow flexion of the diseased arm resulted in unilateral activation of the arm area in the primary motor cortex and in an additional activation of the diaphragm area. This recruitment of the diaphragm area was absent when these patients performed movements of the healthy arm or the foot. As expected, neither the control patient nor the healthy control subjects showed activations in the phrenic nerve area during arm movement. These findings suggest that neuroplasticity may even transform a healthy monofunctional cortical area into a bifunctional area – here shown for the diaphragm area.
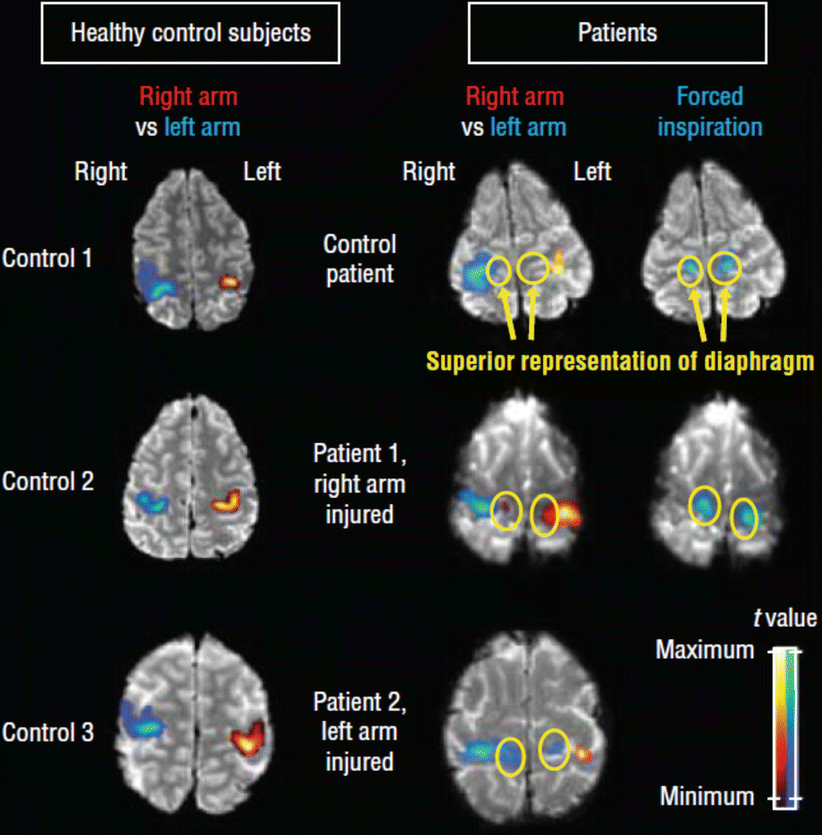
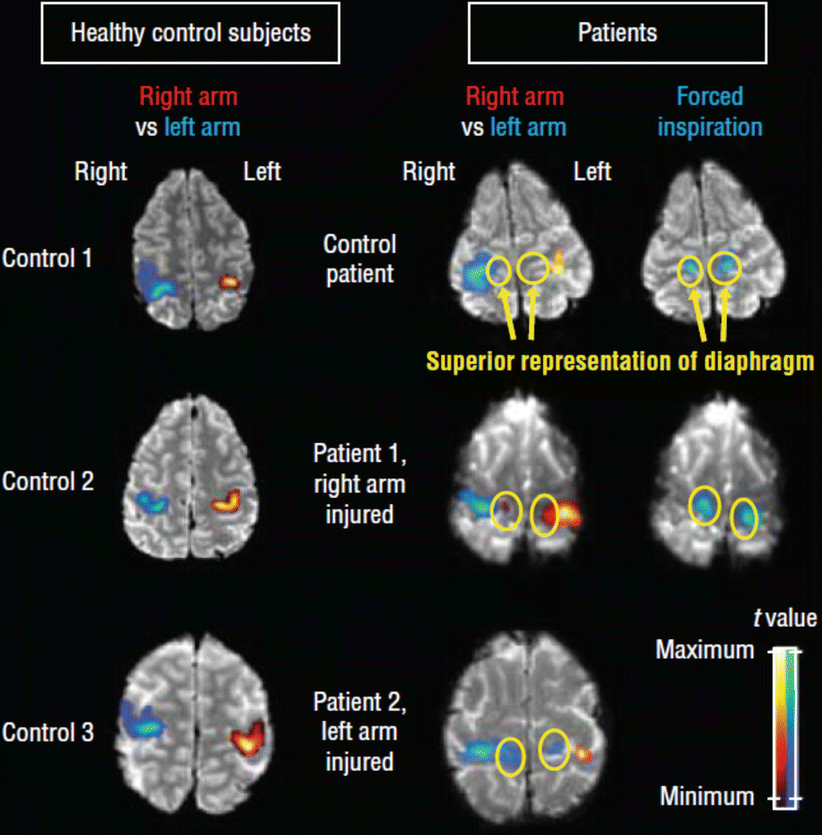
Fig. 2
Original functional magnetic resonance images showing brain activation in the diaphragm area (circles) in the superior primary motor cortex and in the adjacent arm areas. The right hemisphere is shown on the left side. Only primary motor cortex activations are depicted, and colors indicate t value distributions within depicted activation clusters (relative scaling according to local maxima and minima). All images are thresholded at p < .001 uncorrected. Representative slices are shown for the three healthy control subjects, the control patient with C7 end-to-end coaptation, and the two patients with phrenic nerve end-to-side coaptation. The columns labeled “right arm vs left arm” show significant activation differences of right > left arm (red) and left > right arm (blue). In the patients with phrenic nerve end-to-side coaptation, the diaphragm area is activated only with flexion of the injured arm, not with flexion of the healthy arm. In all controls, arm flexion did not activate the diaphragm area. Forced abdominal inspiration selectively activated the diaphragm area in all subjects (rightmost column, blue activation clusters) (Reproduced with permission from Beisteiner et al. (2011)) (Color figure online)
6.3 Neuroplastic Changes of Effective Connectivity in Patients with Heterotopic Hand Replantation
Eickhoff et al. (2008) investigated cortical plasticity in two patients that underwent a resection of parts of the forearm including the elbow joint due to a malignant soft tissue tumor and a subsequent reimplantation of the amputated distal forearm including the hand. Thereby, tendons of the finger flexors and extensors were attached to upper arm muscles, and distal forearm nerves were coaptated with the nerve trunks of the upper arm.
The 2 patients and 14 healthy control subjects performed a motor fMRI task comprising fist-closing movements with the right, left, or both hands simultaneously. Dynamic causal modeling (DCM) was applied to explore effective connectivity within an anatomically and functionally defined bilateral motor network consisting of the primary motor cortex (M1), the supplementary motor area (SMA), the ventral premotor cortex (PMC), and extrastriatal visual cortex (V5). The latter region was defined as the input region for the bilateral SMA and PMC as movements were visually paced in the fMRI task. All regions were defined for the affected hemisphere (hemisphere contralateral to the side of peripheral surgery) and the healthy hemisphere (hemisphere ipsilateral to the side of peripheral surgery). Effects of interest analysis revealed a significantly increased activation during tasks compared to the baseline in the motor ROIs. Intrinsic connectivity, that is, task-independent connectivity, was symmetrically organized in controls (Fig. 3, top panel) with a positive influence (green arrows) of the SMA and PMC on M1 and an inhibition (red arrows) between bilateral M1 and SMA. In contrast, both patients showed altered influence of the PMC of the affected hemisphere. However, the actual patterns of intrinsic connectivity differed between both patients as depicted in Fig. 3 (bottom panel). When moving the healthy hand, the PMC of the affected hemisphere was inhibited by the PMC and M1 of the healthy hemisphere in both patients. Eickhoff and colleagues interpreted this result as an enhanced suppression of the influence of the affected hemisphere on the control of the healthy hand. In both patients, movements of the replanted hand resulted in increased activation of the affected PMC that might reflect an increased effort required to perform the task. In addition, a pathological inhibition of the healthy M1 on the affected M1 was present in both patients when moving the replanted hand. The most prominent difference between the two patients was present in the affected PMC (Fig. 3, bottom panel). In patient J.H., the affected PMC had an increased positive influence (compared to controls) on the M1 of the same hemisphere at rest (intrinsic connectivity) as well as during movements of the replanted hand, while in patient G.M., the M1 was inhibited by the PMC. Correspondingly, motor performance of G.M. was worse than that of J.H. Eickhoff et al. concluded that different neuroplastic connectivity changes were related to clinical progress and behavioral performance stressing the importance of an early and intense rehabilitative exercise as it was the case for patient J.H.
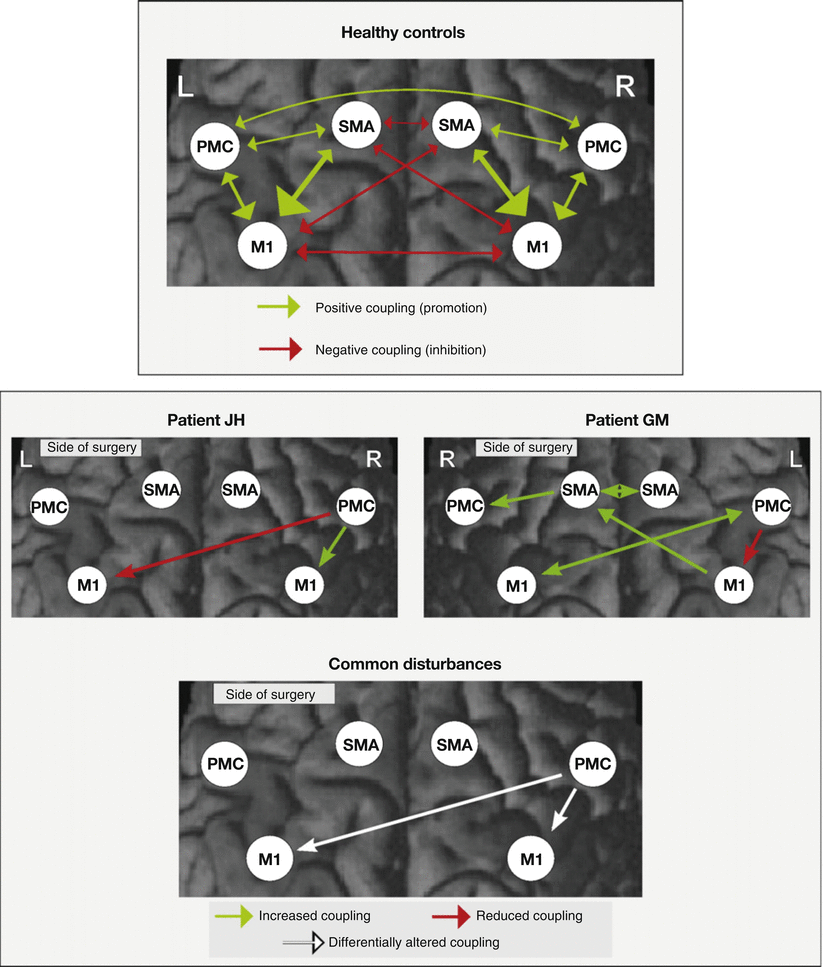
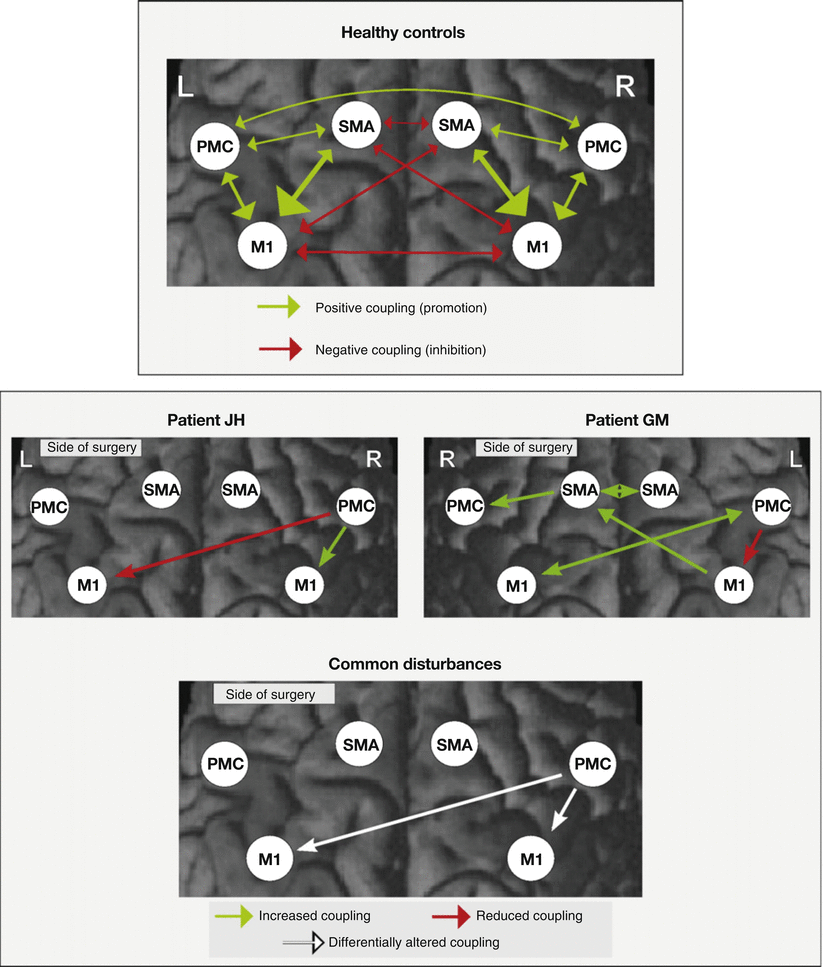
Fig. 3
Top panel: regions of interest selected for estimating cortical connectivity shown on a surface rendering of the MNI single-subject template. Displayed regions were significant at p < 0.05 (corrected) in an F-contrast testing for the effects of interest, that is, regions where hand task-related variance was significantly greater than noise. The intrinsic connectivity in healthy controls (N = 14) as estimated by means of dynamic causal modeling is displayed. Reciprocal connectivity is assumed for the six motor regions, while visual input is provided via left and right V5 into all four premotor regions. Coupling parameters indicate connection strength, which are coded for size and color. Positive coupling (green arrows) suggests facilitation, whereas negative coupling (red arrows) can be interpreted as neural inhibition. Bottom panel: differences in intrinsic connectivity between the two patients and the control group. Only those connections whose strength was significantly different (p < 0.05 corrected) from those of the controls in a two sample T-test are displayed. Green arrows denote coupling parameters which were significantly higher (more positive) as compared to normal subjects, red arrows those significantly lower, that is, more negative. In the synoptical panel in the bottom row, white arrows indicated those connections which were significantly altered in both patients but effect changes in opposite directions (Reproduced with permission from Eickhoff et al. (2008)) (Color figure online)
6.4 Neuroplastic Reorganization of Hand Functions After Hemispherectomy
Rath et al. (2008) reported a single-case fMRI study of a 27-year-old man who underwent a functional hemispherectomy at the age of 11. The patient suffered from an infarction of the right middle cerebral artery at 2 years of age. After the stroke he showed considerable improvements of motor functions of the left paretic hand; however, he developed intractable generalized epilepsy that required a resection of the affected right hemisphere. Although his hemiparesis worsened after hemispherectomy, hand function recovered again after physiotherapy and orthopedic surgeries. At the time of the MR investigation, the patient had spastic hemiparesis but voluntary control and sustained weak motor functions in the hemiparetic arm. Anatomical MRI revealed no pathological findings in the remaining left hemisphere. Functional imaging showed that movements of both hands induced significant activation in the remaining primary motor cortex. Movements of the healthy hand corresponded to activation in the typical hand motor area, whereas movements of the paretic hand produced two activation clusters in the periphery of the hand motor area (Fig. 4). This reorganization is likely to be induced by the hemispherectomy rather than by the initial stroke since hemiparesis showed an initial worsening after hemispherectomy and a secondary improvement after rehabilitation. This single case demonstrates that functional transition zones (i.e., the periphery of a functional cortical area) in the ipsilateral hemisphere can be used to restore functions when no contralateral functional tissue anymore exists.
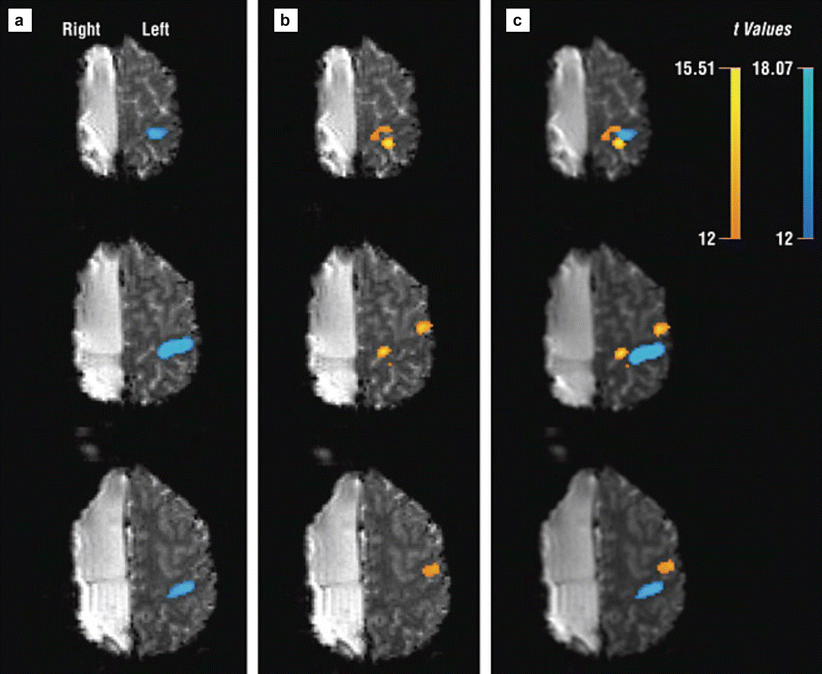
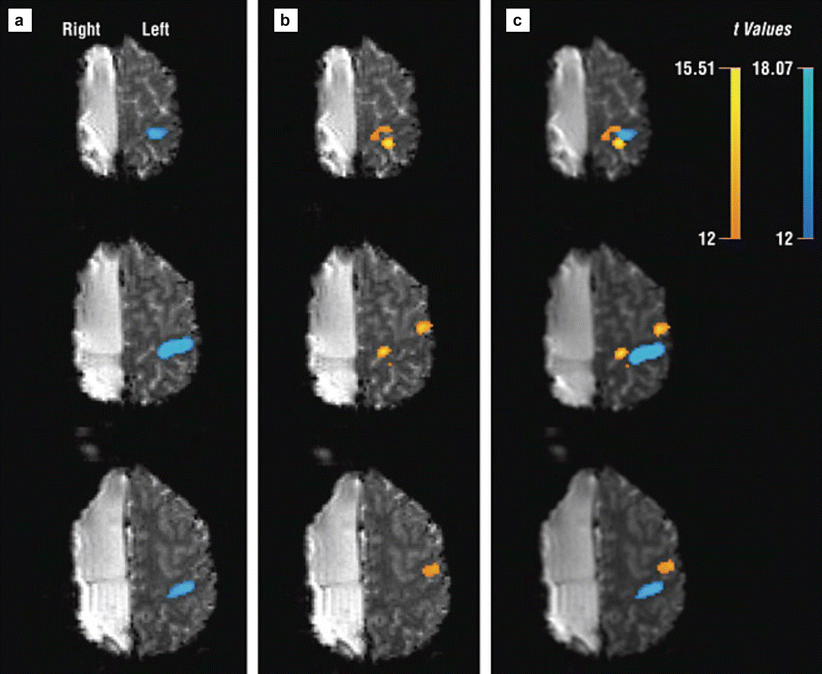
Fig. 4
Functional echoplanar imaging originals (t > 12) showing activation in three slices covering the hand motor area (top row is most superior slice). (a) Contralateral healthy hand activation shown in blue. (b) Ipsilateral paretic hand activation shown in orange. (c) Activation in both hands (Reproduced with permission from Rath et al. (2008)) (Color figure online)
6.5 Structural Neuroplasticity in Patients with Chronic Stroke
Acute stroke often leads to impaired motor and cognitive functions that are sought to be improved by rehabilitation. Patients with only partial recovery suffering from residual impairments after rehabilitation are referred as chronic stroke patients.
Lazaridou et al. (2013b) investigated structural neuroplastic changes corresponding to a rehabilitative motor training by assessing cortical thickness with voxel-based morphometry (VBM) and white matter integrity with DTI in four patients with chronic stroke. The time interval between the onset of left-sided ischemic subcortical middle cerebral artery stroke and the participation in the study was more than 6 months. The patients underwent an 8-week-lasting motor training consisting of 1 h squeezing an exercise ball with the paretic hand for 3 days per week. MR scans were performed at baseline (before the training), after 4 weeks of training, at the end of the training period (after 8 weeks), and finally another 4 weeks later. For DTI fiber reconstruction, deterministic tractography was performed and visually inspected for directionality and location. The pons, the posterior limb of the internal capsule, and the motor cortex were selected as seed regions to reconstruct the corticospinal tract (CST).
Statistical analysis of CST fibers of the affected hemisphere revealed a significant increase in the number of fibers and average tract length as illustrated in Fig. 5 depicting CST fibers of a representative patient before, during, and after motor training. CST fibers (in blue) projected progressively closer to the motor cortex and showed increased density with ongoing training progress. In addition, VBM analysis showed a significantly increased cortical thickness of the ventral postcentral gyrus after training compared to the baseline. With this study, Lazaridou et al. could demonstrate that intensive motor training resulted in structural neuroplasticity in the sensorimotor system in chronic stroke patients and provided a new approach for the evaluation of the rehabilitation progress.
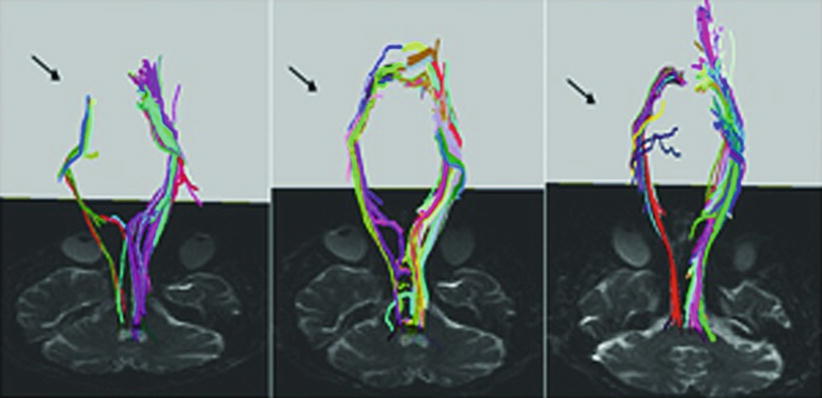
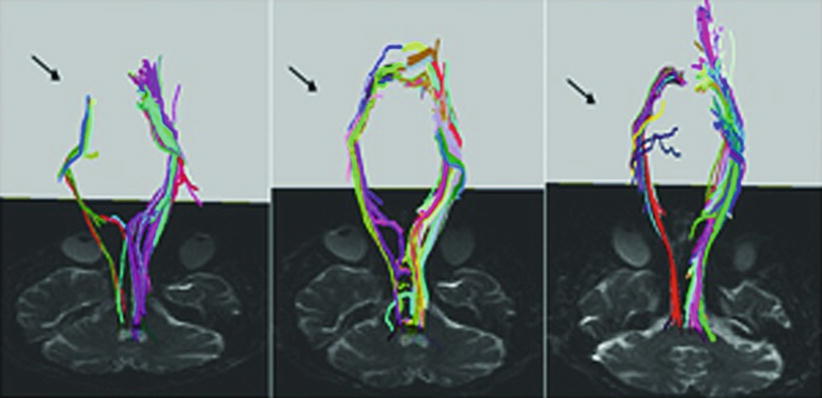
Fig. 5
Reconstructed corticospinal tracts (CSTs) from the same stroke patient before training (left panel), after 4 weeks of training (middle panel) and after 8 weeks of training (right panel). Note that reconstructed CST fibers increased dramatically on the right side with training (arrows) (Reproduced with permission from Lazaridou et al. (2013b))
6.6 Neuroplastic Network Changes in Traumatic Brain Injury
Traumatic brain injury (TBI) is often followed by persistent cognitive deficits affecting several cognitive domains like attention, working memory, speech, and executive functions such as planning, inhibition, and cognitive control.
Caeyenberghs et al. (2012b) addressed the latter issue using a functional imaging approach combined with a graph theoretical analysis. They examined 23 patients with mild to moderate TBI and 26 healthy control subjects applying an event-related fMRI paradigm previously used to investigate cognitive control in young and elderly adults (Coxon et al. 2010) and in TBI patients (Leunissen et al. 2013). Participants performed temporally coupled circular hand movements (either clockwise or counterclockwise) with each hand using a joystick. Visual cues indicated whether to switch the movement patterns from asymmetric to symmetric or vice versa or to continue the prior movement. The authors constructed a switching motor network consisting of 22 regions of interest that were defined as 10 mm spheres centered on peak activation coordinates reported by Coxon et al. (2010) and Leunissen et al. (2013). The network comprised regions of the medial frontal cortex, anterior cingulate cortex, bilateral dorsolateral prefrontal cortex, bilateral inferior frontal cortex (BA 44), basal ganglia, cerebellum, right precuneus, left premotor cortex, bilateral insula, and right superior and inferior parietal cortex. First, a contrast Switch > Continue was conducted to compute the average time series for each ROI of each subject which was then subjected to a partial correlation analysis. This method allowed a quantification of the unique correlation between two ROIs and the content filtering of the effects of all the other ROIs giving an appropriate measure for functional connections. For graph theoretical analysis, matrices with significant connections weighted by the correlation values between nodes (i.e., ROIs) were constructed. Weighted matrices were used to calculate the mean connection strength of the single nodes as well as of the overall network connection strength. The Brain Connectivity Toolbox (Rubinov and Sporns 2010) was employed to assess global network architecture and nodal properties.
TBI patients exhibited slower response times and more disruptions during the switching task than the controls that could not be explained by motor difficulties. Graph theoretical analysis revealed a significantly altered functional network in patients with higher connectivity degree and strength than in controls. Specifically, the patients exhibited a higher connectivity in the left ventral portion of M1 (compare yellow sphere in Fig. 6) possibly reflecting functional compensation as this area is involved in coordination and is strongly interconnected within the primary motor cortex. In addition, local efficiency was enhanced in patients with TBI which might indicate a compensatory increase of short-range connections between adjacent nodes. Besides, graph theoretical analysis provided information about hubs, that is, regions with a central role in a functional network. In both the patients and controls, the right insula with its critical role in the differentiation between internal and external stimuli presented as a hub, putatively due to the strong directive of a visual input to trigger movement switching. Interestingly, two regions mainly involved in movement planning (right dorsolateral prefrontal cortex and left dorsal premotor cortex) acted as hubs in the TBI group only. Caeyenberghs and colleagues (2012b) concluded that TBI patients might rely more on cognitive control and performance monitoring during the switching task. However, the higher mean connectivity degree in patients was related with poorer switching performance implying that increased connectivity not necessarily improves function. Moreover, higher connectivity degree was positively correlated with injury severity and might therefore be a result of a more severe brain damage reflecting a higher effort to manage cognitive control tasks.
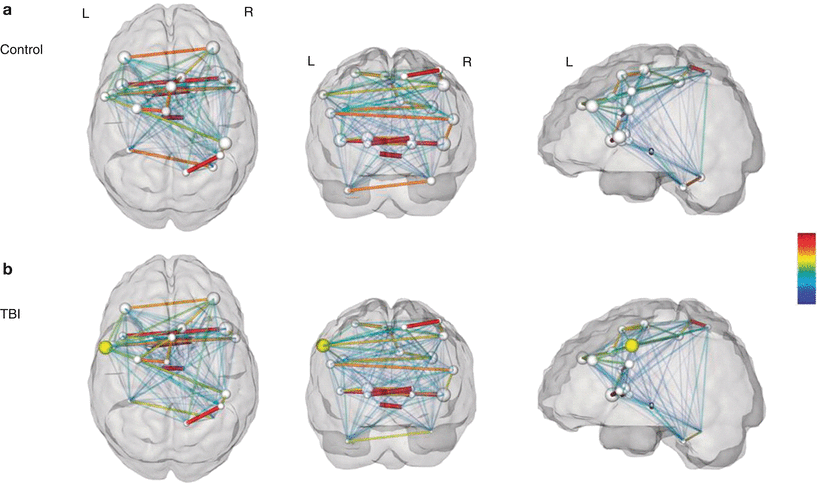
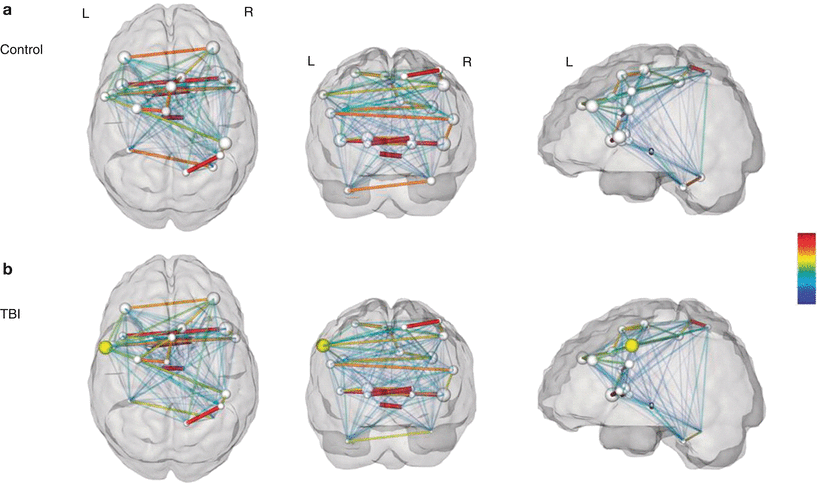
Fig. 6
Group differences in connectivity degree. (a) Controls and (b) patients with TBI. The size of the regions of interest (spheres) represents connectivity degree. The edge widths represent the strength of the partial correlations between nodes. Notably, the networks shown here were constructed by summing the partial correlation matrices of all subjects in each group (as shown by the color bar). Yellow sphere indicates higher connectivity in left ventral premotor cortex (p < 0.002 corrected) in patients with TBI (Reproduced with permission from Caeyenberghs et al. (2012b)) (Color figure online)
References
Allendorfer JB, Storrs JM, Szaflarski JP (2012) Changes in white matter integrity follow excitatory rTMS treatment of post-stroke aphasia. Restor Neurol Neurosci 30:103–113. doi:10.3233/RNN-2011-0627 PubMedCentralPubMed
Altenmüller E, Müller D (2013) A model of task-specific focal dystonia. Neural Netw 48:25–31. doi:10.1016/j.neunet.2013.06.012 PubMed
Andersson D, Wilhelmsson U, Nilsson M, Kubista M, Ståhlberg A, Pekna M, Pekny M (2013) Plasticity response in the contralesional hemisphere after subtle neurotrauma: gene expression profiling after partial deafferentation of the hippocampus. PLoS One 8:e70699PubMedCentralPubMed
Appel-Cresswell S, de la Fuente-Fernandez R, Galley S, McKeown MJ (2010) Imaging of compensatory mechanisms in Parkinson’s disease. Curr Opin Neurol 23:407–412. doi:10.1097/WCO.0b013e32833b6019 PubMed
Araque A, Navarrete M (2010) Glial cells in neuronal network function. Philos Trans R Soc Lond B Biol Sci 365:2375–2381. doi:10.1098/rstb.2009.0313 PubMedCentralPubMed
Bates E, Wilson SM, Saygin AP, Dick F, Sereno MI, Knight RT, Dronkers NF (2003) Voxel-based lesion-symptom mapping. Nat Neurosci 6:448–450PubMed
Beauchamp MS, Ro T (2008) Neural substrates of sound-touch synesthesia after a thalamic lesion. J Neurosci 28:13696–13702. doi:10.1523/JNEUROSCI.3872-08.2008 PubMed
Beaulieu JY, Blustajn J, Teboul F, Baud P, De Schonen S, Thiebaud JB, Oberlin C (2006) Cerebral plasticity in crossed C7 grafts of the brachial plexus: an fMRI study. Microsurgery 26:303–310PubMed
Beisteiner R (2013) Clinical fMRI – improving paradigms or field strengths? AJNR Am J Neuroradiol 34:1972–1973. doi:10.3174/ajnr.A3722 PubMed
Beisteiner R, Höllinger I, Rath J, Wurnig M, Hilbert M, Klinger N, Geißler A, Fischmeister F, Wöber C, Klösch G, Millesi H, Grishold W, Auff E, Schmidhammer R (2011) New type of cortical neuroplasticity after nerve repair in brachial plexus lesions. Arch Neurol 68:1147–1470. doi:10.1001/archneurol.2011.596
Benzagmout M, Gatignol P, Duffau H (2007) Resection of World Health Organization Grade II gliomas involving Broca’s area: methodological and functional considerations. Neurosurgery 61:741–752PubMed
Bettus G, Ranjeva JP, Wendling F, Bénar CG, Confort-Gouny S, Régis J, Chauvel P, Cozzone PJ, Lemieux L, Bartolomei F, Guye M (2011) Interictal functional connectivity of human epileptic networks assessed by intracerebral EEG and BOLD signal fluctuations. PLoS One 6:e20071. doi:10.1371/journal.pone.0020071 PubMedCentralPubMed
Binkofski F, Seitz RJ (2004) Modulation of the BOLD-response in early recovery from sensorimotor stroke. Neurology 63:1223–1229PubMed
Bitter T, Sorger B, Hesselmann V, Krug B, Lackner K, Guntinas-Lichius O (2011) Cortical representation sites of mimic movements after facial nerve reconstruction: a functional magnetic resonance imaging study. Laryngoscope 121:699–706. doi:10.1002/lary.21399 PubMed
Bonelli SB, Thompson PJ, Yogarajah M, Vollmar C, Powell RH, Symms MR, McEvoy AW, Micallef C, Koepp MJ, Duncan JS (2012) Imaging language networks before and after anterior temporal lobe resection: results of a longitudinal fMRI study. Epilepsia 53:639–650. doi:10.1111/j.1528-1167.2012.03433.x PubMed
Braun M, Finke C, Ostendorf F, Lehmann TN, Hoffmann KT, Ploner CJ (2008) Reorganization of associative memory in humans with long-standing hippocampal damage. Brain 131:2742–2750. doi:10.1093/brain/awn191 PubMed
Briganti C, Sestieri C, Mattei PA, Esposito R, Galzio RJ, Tartaro A, Romani GL, Caulo M (2012) Reorganization of functional connectivity of the language network in patients with brain gliomas. AJNR Am J Neuroradiol 33:1983–1990. doi:10.3174/ajnr.A3064 PubMed
Buma FE, Lindeman E, Ramsey NF, Kwakkel G (2010) Functional neuroimaging studies of early upper limb recovery after stroke: a systematic review of the literature. Neurorehabil Neural Repair 24:589–608. doi:10.1177/1545968310364058 PubMed
Bütefisch CM, Wessling M, Netz J, Seitz RJ, Hömberg V (2008) Relationship between interhemispheric inhibition and motor cortex excitability in subacute stroke patients. Neurorehabil Neural Repair 22:4–21PubMed
Cadotte DW, Bosma R, Mikulis D, Nugaeva N, Smith K, Pokrupa R, Islam O, Stroman PW, Fehlings MG (2012) Plasticity of the injured human spinal cord: insights revealed by spinal cord functional MRI. PLoS One 7:e45560. doi:10.1371/journal.pone.0045560 PubMedCentralPubMed
Caeyenberghs K, Leemans A, De Decker C, Heitger M, Drijkoningen D, Linden CV, Sunaert S, Swinnen SP (2012a) Brain connectivity and postural control in young traumatic brain injury patients: a diffusion MRI based network analysis. Neuroimage Clin 1:106–115. doi:10.1016/j.nicl.2012.09.011 PubMedCentralPubMed
Caeyenberghs K, Leemans A, Heitger MH, Leunissen I, Dhollander T, Sunaert S, Dupont P, Swinnen SP (2012b) Graph analysis of functional brain networks for cognitive control of action in traumatic brain injury. Brain 135:1293–1307. doi:10.1093/brain/aws048 PubMed
Calabrese M, Rinaldi F, Seppi D, Favaretto A, Squarcina L, Mattisi I, Perini P, Bertoldo A, Gallo P (2011) Cortical diffusion-tensor imaging abnormalities in multiple sclerosis: a 3-year longitudinal study. Radiology 261:891–898. doi:10.1148/radiol.11110195 PubMed
Carmichael ST, Archibeque I, Luke L, Nolan T, Momiy J, Li S (2005) Growth-associated gene expression after stroke: evidence for a growth-promoting region in peri-infarct cortex. Exp Neurol 193:291–311PubMed
Carter AR, Astafiev SV, Lang CE, Connor LT, Rengachary J, Strube MJ, Pope DL, Shulman GL, Corbetta M (2010) Resting interhemispheric functional magnetic resonance imaging connectivity predicts performance after stroke. Ann Neurol 67:365–375. doi:10.1002/ana.21905 PubMedCentralPubMed
Carter AR, Patel KR, Astafiev SV, Snyder AZ, Rengachary J, Strube MJ, Pope A, Shimony JS, Lang CE, Shulman GL, Corbetta M (2012) Upstream dysfunction of somatomotor functional connectivity after corticospinal damage in stroke. Neurorehabil Neural Repair 26:7–19. doi:10.1177/1545968311411054 PubMed
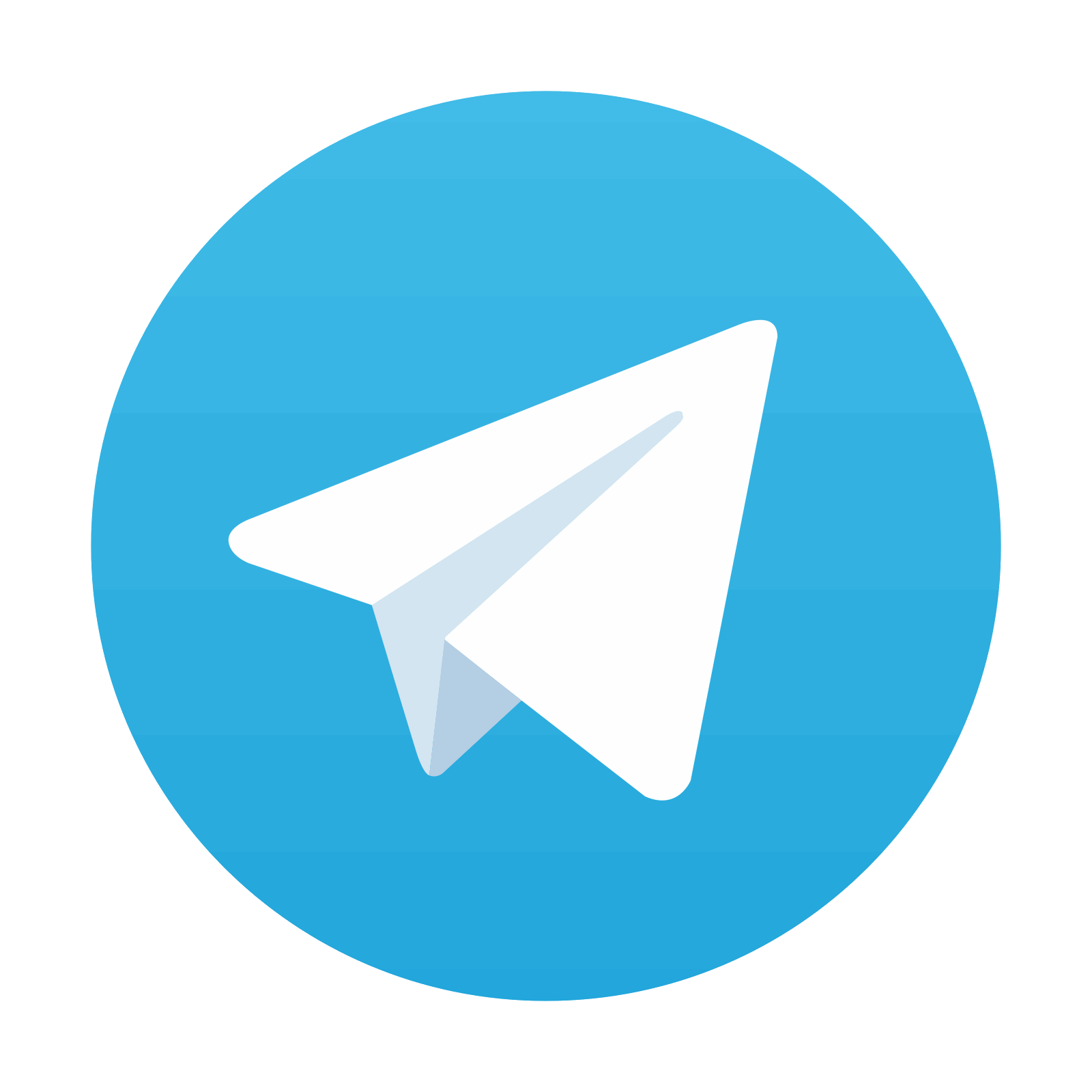
Stay updated, free articles. Join our Telegram channel

Full access? Get Clinical Tree
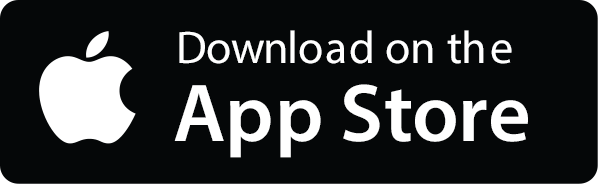
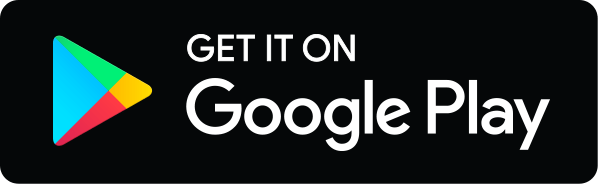