Fig. 2.1
Basic protocol for simultaneous cardiac PET/MR imaging. HASTE axial 2D half Fourier acquisition single-shot turbo spin echo sequence, μ attenuation map (μ-map) using 2-point Dixon sequences, Cine Cine imaging using balanced SSFP sequence, T2w T2-weighted imaging using turbo inversion recovery magnitude sequence, Rest Perfusion rest perfusion imaging using saturation recovery fast low-angle shot sequence, Gd ~10 min waiting for late gadolinium enhancement, LGE late gadolinium enhancement imaging using segmented 2D inversion recovery turbo FLASH sequence
Myocardial PET imaging with FDG usually requires a special dietary preparation [3, 6], which is known to be a source of patient discomfort and potential incompliance. In addition, the relatively long duration of cardiac MRI examinations associated with noise, narrowness, and immobility can result in premature cancellation of examinations by the patient. Indeed, a cardiac PET/MRI study revealed long examination times, the PET/MR imaging examination itself, and fasting to be the main sources of discomfort, resulting in only moderate patient compliance [5]. Thus, it seems mandatory to further optimize patient preparation and implement compact imaging protocols to pave the way for the clinical establishment of cardiac PET/MRI.
2.3 MRI-Based Attenuation Correction
Since there is no direct translation of MR-acquired image contrasts into gamma ray attenuation coefficients of tissue, alternative techniques for attenuation correction of PET data had to be developed. While in principle several methods are available [7], currently an MR-based image segmentation approach is typically used in integrated cardiac PET/MR imaging [8]. This method usually segments tissue into four compartments (background, lung, fat, and soft tissue) with fixed attenuation coefficients assigned to each compartment. Consequently, the resulting attenuation maps are relatively coarse as finer nuances between tissue attenuations are not represented; e.g., the myocardium vs. the blood, which are both classified as soft tissue. Moreover, the bone is also classified as soft tissue and thus severely misclassified regarding its attenuation for gamma rays. Therefore, in the bone tissue and in its vicinity, standardized uptake values (SUVs) derived from PET/MRI systems might be significantly underestimated when compared to standardized uptake values derived from PET/CT [9]. Using ultrashort echo time (UTE) sequences, the segmentation of tissue with very short T2* (such as the bone) has been demonstrated to be feasible [10]. However, this technique is still limited to a rather small field of view and thus not yet available in cardiac PET/MR imaging.
The segmentation-based approach is dependent on the accuracy of the tissue segmentation algorithm, which can be significantly wrong in the presence of artifacts in the underlying MR images. Artifacts that are frequently found in cardiac imaging often originate from foreign objects like implantable port systems, sternal wire cerclages, artificial heart valves, or artificial joint replacement of the humerus (Fig. 2.2).
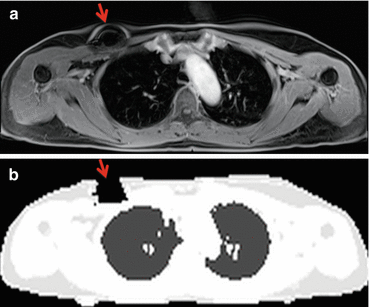
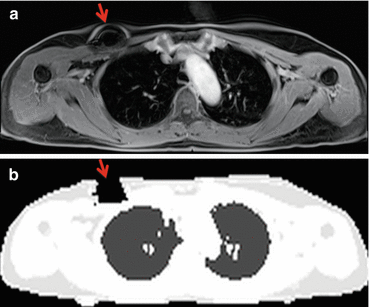
Fig. 2.2
Source of imaging artifacts. Implanted port system causing MR imaging artifacts (a, arrow) resulting in erroneous attenuation coefficients in μ-maps (b, arrow)
Also, cardiac MR imaging is usually performed with the patient’s arms aligned along the body axis. In larger patients, this can result in parts of the arms being placed outside the MR field of view, causing so-called truncation artifacts at the edges of the attenuation maps. This can at least partially be avoided by imaging in the arms-up position, extension of the MR field of view [11], or partial correction with PET emission data using maximum-likelihood reconstruction of attenuation and activity (MLAA) [12].
Nevertheless, segmentation-based attenuation correction has been demonstrated to be a reasonably reliable procedure with regard to visual assessment of PET data by the experienced observer [8]. Regarding cardiac PET/MR imaging, it has been shown to provide excellent visual PET image quality that was in good concordance with corresponding PET images from PET/CT [5]. In a study comparing standardized uptake values for FDG using PET/MR and PET/CT in normal organ tissues of oncologic patients, excellent correlations between both modalities were found in the myocardium [13]. In another study in oncologic patients, an excellent correlation of myocardial standardized uptake values between non-ECG-gated PET/MRI and PET/CT has been reported. Furthermore, a variation over the cardiac cycle with higher standardized uptake values at end-systole and lower standardized uptake values at end-diastole was observed using ECG-gated PET/MRI [14]. In a dedicated cardiac PET/MRI study in patients with myocardial infarction, analysis of maximum standardized uptake values in the myocardium showed considerable variation between PET/MRI- and PET/CT-acquired data, while pairwise comparison did not show a significant difference between both modalities [5]. In summary, quantitative PET data acquired on an integrated PET/MR scanner should still be treated with caution, and visual assessment of attenuation maps and PET images for technical errors is mandatory (Fig. 2.3).
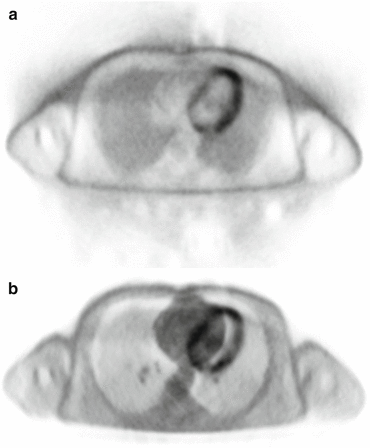
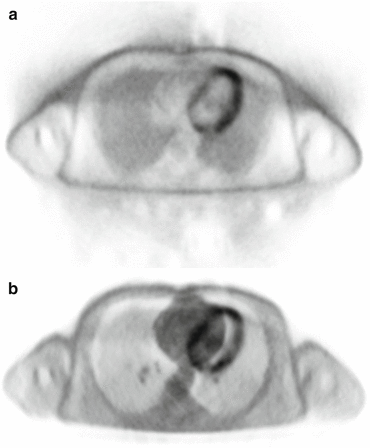
Fig. 2.3
Source of imaging artifacts. PET image artifacts due to patient movement (primarily in z-axis direction) during PET acquisition (a) (PET image without attenuation correction). The artifacts are even more pronounced using the wrong (no longer correctly co-registered) μ-map for attenuation correction (b)
2.4 Acute Myocardial Infarction
An initial report of integrated PET/MRI in a patient with a large transmural infarction after occlusion of the left circumflex artery and symptom-to-reperfusion time of 6 h demonstrated a nearly perfect match between the myocardium showing late gadolinium enhancement and the myocardium showing reduced FDG uptake [1]. In a feasibility study in patients with acute and chronic myocardial infarction, a good agreement between myocardial segments showing late gadolinium enhancement (LGE) and reduced FDG uptake was found. However, that feasibility study was based on visual evaluation of PET and LGE images by reference to the 17-segment model of the left ventricle, and no subgroup analyses between acute and chronic myocardial infarction were performed. Slightly more segments with reduced FDG uptake were found; however, particularly the findings in one patient with acute occlusion of the left anterior descending artery with ST-elevation myocardial infarction and symptom-to-reperfusion time of 3 h were unexpected. While no infarction was found on LGE images, PET images showed a reduced tracer uptake in the anterior wall that closely matched myocardial edema found on T2-weighted images [5]. Therefore it was hypothesized that reduced FDG uptake could be related to the area at risk in patients with reperfused acute myocardial infarction. In a follow-up study in patients with reperfused acute myocardial infarction, PET and MRI were compared by quantitative assessment of the myocardium showing late gadolinium enhancement and reduced FDG uptake. Also, the endocardial surface area (ESA) was calculated from LGE images and used as a reference standard for the area at risk. It was found that the area showing reduced FDG uptake was significantly larger than the area showing late gadolinium enhancement (Fig. 2.4). Furthermore, in six patients with complete salvage after reperfusion (in terms of showing no late gadolinium enhancement), an area of reduced FDG uptake was found in the perfusion territory of the culprit artery. And finally in patients with both late gadolinium enhancement and reduced FDG uptake, a good correlation between the area of reduced FDG uptake and the endocardial surface area was found [15]. However, the ESA method for the assessment of the area at risk is technically limited in that it cannot detect the area at risk in the absence of LGE or its extent lateral to the myocardium showing LGE. Thus, further validation using an alternative standard of reference such as the assessment of myocardial edema by T2-weighted MR imaging is needed. If reduced FDG uptake can be confirmed to reflect the area at risk in terms of a metabolic imprint of ischemia, PET/MRI could allow for the retrospective assessment of myocardial salvage in patients with reperfused acute myocardial infarction. This could be used for the prediction of left ventricular functional recovery in these patients or as a surrogate parameter for the evaluation of strategies to reduce infarct size, such as pre- and post-conditioning or remote conditioning.
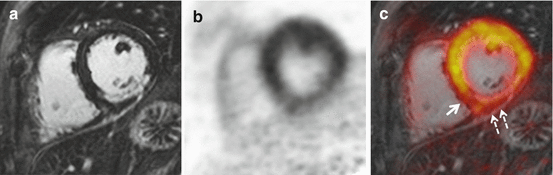
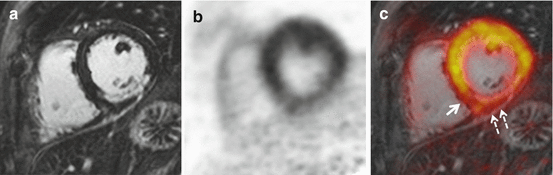
Fig. 2.4
FDG PET/MRI in a patient with reperfused acute myocardial infarction. Late gadolinium-enhanced MRI demonstrated 50–90 % transmural myocardial infarction in the perfusion territory of the right coronary artery (a). FDG uptake (b) was significantly reduced in the infarct area. Closer inspection of fused images (c) and comparison between late gadolinium enhancement and FDG uptake reveals lateral (c, solid arrow) and epicardial (c, dashed arrows) extent of reduced FDG uptake. Overall, the area of reduced FDG uptake was significantly larger than the infarct area and thus could rather correspond to the total area at risk than myocardial necrosis alone
2.5 Chronic Myocardial Infarction and Ischemic Cardiomyopathy
Data on simultaneous PET/MR imaging of coronary artery disease is still sparse. The available data from PET/CT and MR studies indicates that simultaneous PET/MRI with tracers for PET perfusion imaging could be a powerful modality for the assessment of coronary artery disease and myocardial viability. In such imaging protocols, viability imaging could be performed using LGE MRI, which seems not only to be the most accurate method but would also allow omitting FDG imaging. This could greatly simplify the PET examination as the complex task of a dual-tracer study would become obsolete. On the other hand, PET myocardial perfusion imaging has been demonstrated to yield the highest sensitivity and specificity for the noninvasive detection of coronary stenosis and to allow for the absolute quantification of myocardial blood flow, which thus could be an improvement over MR perfusion imaging [16]. In an older side-by-side comparison of sequentially performed FDG PET/CT and LGE MRI of the heart in patients with known coronary artery disease, a close agreement between PET and MRI in detecting transmural myocardial scars was found. However, a significant number of segments with subendocardial late gadolinium enhancement showed normal FDG uptake in the PET study, thus indicating a higher sensitivity of LGE imaging for the detection of subendocardial scars. These findings have not yet been confirmed using simultaneous PET/MRI. Preliminary data shows the feasibility of simultaneous PET/MR perfusion imaging using 13 N-ammonia. In this context, a protocol for the parallel acquisition and comparison of simultaneous PET and MR measurements of myocardial perfusion at rest and during pharmacologic stress has been demonstrated [17]. A more recent study compared 13 N-ammonia PET/MR to SPECT perfusion imaging and reported superior specificity and diagnostic accuracy of PET/MR in patients with reversible ischemia [18].
2.6 Myocarditis and Cardiac Sarcoidosis
FDG PET/MRI represents a promising noninvasive imaging technique to identify and monitor inflammatory condition of the heart. Cardiac MRI has become the reference standard for the imaging assessment of myocardial inflammation, as it allows a multiparametric evaluation of cardiac function, myocardial edema, and necrosis as well as myocardial hyperemia and pericardial effusion [19]. With cardiac MRI, one can rapidly detect the disease, evaluate its extent, and quantify the impairment of cardiac function. FDG PET, however, is increasingly recognized as a valuable tool for the assessment of various inflammatory conditions including those of the heart [20]. FDG uptake is not only a sensitive marker of inflammation but also a quantifiably parameter for disease activity that could complement cardiac MRI in the detection, differential diagnosis, and monitoring of cardiac inflammation.
PET imaging of myocardial inflammation using FDG is complicated by the fact that cardiomyocytes metabolize a variable mixture of glucose and fatty acids under uncontrolled conditions, with a preference for fatty acid metabolism. To differentiate FDG uptake of intramyocardial inflammatory infiltrates from normal myocardial FDG uptake, the latter needs to be shifted to a more or less exclusive utilization of fatty acids. However, several techniques including long fasting, high-fat low-carbohydrate diet, and heparin loading have been demonstrated to successfully suppress myocardial FDG uptake [21–23].
To date, no studies have been published that evaluate PET/MR for the imaging of myocarditis. However, several case reports indicate the potential of PET/MRI in that context. One report has demonstrated the use of FDG PET/MRI in a case of myocarditis caused by parvovirus B19 and highlights the multiparametric imaging features available for diagnostic workup [24]. While neither an impairment of cardiac function nor a pericardial effusion was found, T2 and LGE imaging revealed focal edema and necrosis in the anterior left ventricular wall, matched by significant hyperemia as assessed by dynamic MR perfusion imaging. These findings were in excellent agreement with increased FDG uptake in the anterior wall.
In addition, another case report demonstrated the feasibility of FDG PET/MRI in a case of diffuse myocarditis of the lateral wall caused by an Epstein-Barr virus infection [25]. In this case, T2-weighted MRI only revealed very subtle signs of myocardial edema, while LGE imaging demonstrated focal intramyocardial nodules and diffuse subepicardial enhancement of the lateral wall. Therefore, these MR imaging findings were compatible with either acute myocarditis or chronic myocarditis or myocardial scarring. However, intense FDG uptake in the lateral wall allowed for the diagnosis of acute myocarditis.
Several case reports have already demonstrated the feasibility of FDG PET/MRI in cardiac sarcoidosis [4, 26, 27]. A study in 51 consecutive patients with cardiac sarcoidosis has found an improved diagnostic accuracy of combined FDG PET/MRI over PET and MRI alone [28]. Cardiac involvement is the major risk factor for fatal outcome in sarcoidosis, often manifesting in arrhythmia and heart failure. Besides direct treatment of cardiac symptoms, in many cases therapy comprises also immunosuppression, which needs to be carefully balanced regarding side effects. Thus, not only methods for the accurate diagnosis but also for monitoring of disease activity with the objective of drug titration are needed (Figs. 2.5 and 2.6).