Clinical indication
Typical brain perfusion SPECT findings
Differential diagnosis in dementia (Borghesani et al. 2010)
Alzheimer’s disease (AD)
Hypoperfusion of bilateral temporoparietal association cortices (often asymmetric) with characteristic sparing of the primary sensorimotor cortex, primary visual cortex, and basal ganglia. Early changes also include hypoperfusion of the posterior cingulate (Bradley et al. 2002; Devous 2002; Bonte et al. 2004) (Fig. 5.1). In later stages, the frontal cortex may also show hypoperfusion (Brown et al. 1996; Bradley et al. 2002)
Dementia with Lewy bodies (DLB)
Main differentiating feature from AD is occipital hypoperfusion (Lobotesis et al. 2001). Similar to AD, may have mild perfusion deficit in temporoparietal and frontal regions, but, unlike AD, DLB usually spares medial temporal lobes (Fig. 5.2). Additional findings of hyperperfusion in the thalamus and bilateral striatum may strengthen the accuracy of the diagnosis of DLB (Shimizu et al. 2008)
Because of the loss of dopaminergic neurons in the nigrostriatal pathway, DaTSCAN SPECT imaging can also be performed to enhance diagnostic accuracy of DLB and differentiate this parkinsonian syndrome from AD (Papathanasiou et al. 2012)
Multi-infarct dementia
Multiple, randomly distributed focal areas of hypoperfusion. Hypoperfusion of motor and sensory cortices, although not always involved, can help to differentiate multi-infarct dementia from AD. Correlation with anatomic images, e.g., CT or MRI, is extremely important, as cortical or subcortical infarcts may be seen increasing the confidence in diagnosis. Subcortical infarcts alone, without cortical lesions on CT, can explain nearby cortical perfusion defects by disconnection between cortical and subcortical neurons. The Binswanger type of dementia, a rare variant of vascular dementia, is a gradually progressive syndrome caused by diffuse or patchy ischemic events to the deep white matter. The magnitude of cortical hypoperfusion has been shown to correlate with the severity of the disease (Tohgi et al. 1991)
Frontotemporal dementia (FTD)
Perfusion deficits in orbitofrontal area and temporal lobes, often asymmetric (Fig. 5.5). Basal ganglia may also be hypoperfused. When the right temporal lobe is involved, behavioral disturbances are usually a prominent clinical feature; aphasia is more frequent when the left temporal lobe is involved. In Pick’s disease, the frontal lobe is more affected than temporal lobe, with relatively symmetric hypoperfusion of the frontal lobes often extending to the cingulate gyrus (Miller et al. 1991)
Parkinson’s disease (PD) and other parkinsonian disorders
There is no consensus on a specific brain perfusion SPECT pattern in Parkinson’s disease or other parkinsonian disorders. However, generalized hypoperfusion of the cortex has been described. Brain perfusion SPECT is most useful to differentiate PD from the causes of dementia listed above. Demented PD patients and AD patients may share a common pattern of marked posterior hypoperfusion. However, the defects are more prominent and extensive in AD (Spampinato et al. 1991)
As with DLB, DaTSCAN SPECT imaging is extremely helpful in confirming the diagnosis of parkinsonian disorders because of the loss of dopaminergic neurons in the nigrostriatal pathway (Fig. 5.3)
Huntington’s disease (HD)
Decreased or absent tracer uptake in the bilateral caudate nucleus or basal ganglia, not necessarily symmetric. The sensitivity of brain SPECT with perfusion agents in HD has been high, even in patients with normal CT or MRI findings. Decreased caudate nuclei uptake has also been reported for several individuals at risk of HD who have undergone brain SPECT with perfusion tracers prior to onset of symptoms (Ichise et al. 1993). Brain perfusion SPECT findings may also be combined with DaTSCAN SPECT to enhance diagnostic accuracy
Detection of acute ischemia (Ueda and Yuh 2005)
Focal or regional area of absent perfusion or hypoperfusion immediately following the ischemic event. In early acute ischemia, changes can be seen on brain perfusion SPECT that are not yet visible on CT or MRI, making this a modality of choice in the first few hours following the event. The hypoperfused region on SPECT will generally be larger than the lesion that eventually becomes visible on CT or MRI. Crossed cerebellar diaschisis is frequent in cortical strokes and is caused by disconnection of the cerebellar–corticopontine fibers as a consequence of ischemia or stroke. With either 99mTc-HMPAO or 99mTc-ECD, the perfusion defect will be fixed; however, with 123I-IMP, redistribution with partial reperfusion may occur
The timing of the study is important as the sensitivity for stroke localization may decrease as the stroke evolves due to the luxury perfusion phenomenon. Focal hyperperfusion of the lesion secondary to relative hyperemia can be seen at days 1–5 following the ischemia event and persist as long as 20 days. By 30 days following the initial ischemic event, the hypoperfused area should easily be detected again. Between the hyperemic and the delayed hypoperfusion phases, study findings may appear normal. Luxury perfusion may be easier to detect with 99mTc-HMPAO than with 99mTc-ECD (Miyazawa et al. 1998)
False-negative brain perfusion SPECT findings can frequently occur in lacunar or small cortical infarcts
Focally or regionally absent perfusion or hypoperfusion at the site of vasospasm. Findings correlate well with the severity and magnitude of neurologic deficits in the evolution of the condition. A follow-up post-interventional study should be obtained to confirm restoration of perfusion to the affected region
Candidacy for fibrinolytic therapy (Alexandrov et al. 1996)
Mild to moderate hypoperfusion in the vascular distribution of an acute stroke confers favorable outcome from fibrinolytic therapy
Complete absence of tracer uptake in the vascular distribution of the stroke suggests poor prognosis and is associated with an increased rate of hemorrhagic complication from fibrinolytic therapy
A normal brain SPECT image in a patient with CT or MRI evidence of stroke implies preexisting effective collateral circulation with favorable prognosis whether fibrinolytic therapy is used or not
Focal hyperperfusion at the epileptogenic focus may be seen, particularly in temporal lobe epilepsy (Fig. 5.4). Hyperperfusion may extend to the ipsilateral basal ganglia and thalamus and possibly also into the ipsilateral motor cortex and contralateral cerebellar cortex. Crossed cerebellar hyperperfusion of the contralateral cerebellum has been reported in up to 75 % of ictal studies for temporal lobe epilepsy, providing increased confidence in lateralization of the seizure focus
In frontal lobe seizures, the difficulty in detecting the epileptogenic focus is caused by the short duration of the seizure and the magnitude of hyperperfusion, which is frequently less than that of temporal lobe epilepsy
As described in the Seizure Imaging section, ictal images should always be interpreted in comparison with the interictal images, as subtle hyperpefusion relative to interictal hypoperfusion at the same site may only be appreciated when viewing the ictal and interictal images simultaneously or when using software to compare quantifiable differences in regional cerebral brain flow
Interictal detection of seizure focus (Devous et al. 1998)
Interictal SPECT images may show focal or diffuse hypoperfusion of the epileptic focus. However, in approximately 50 % of patients, the interictal SPECT will be normal without any perfusion abnormalities. The sensitivity of interictal brain SPECT alone is relatively low at 44 %; therefore, interictal images should be interpreted in conjunction with ictal images whenever possible to identify foci of increased perfusion in the ictal state that were normal or hypoperfused in the interictal state
Brain death (Al-Shammri and Al-Feeli 2004)
Absent cerebral and cerebellar perfusion supports the clinical diagnosis of brain death. Planar images can generally confirm the absence of intracranial perfusion; however, SPECT may be useful in equivocal cases, particularly to differentiate persistent cerebral perfusion from hyperperfusion to overlying soft tissue injuries
Brain perfusion SPECT is more sensitive than either CT or MRI in detection of brain abnormalities, especially in the acute (<24 h) phase following head injury. Brain SPECT will often show focal, multifocal, or regional areas of hypoperfusion that correlate well with the neurologic status of the patient
Focal cerebral hyperemia after head injury was associated with a lower mortality rate and better outcome than was lack of hyperemia after head injury. Also, normal brain SPECT findings were found to be a reliable tool in the exclusion of the clinical sequelae of mild head injury
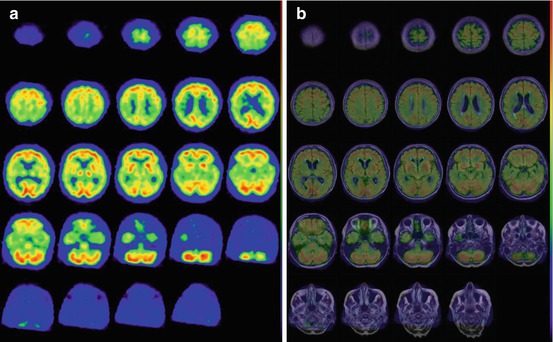
Fig. 5.1
In an 81-year-old woman with progressive cognitive decline and Mini-Mental Status Exam score = 24/30, (a) transaxial 99mTc-HMPAO SPECT images show severe hypoperfusion in the temporoparietal association cortices and posterior cingulate, with sparing of the occipital cortex, sensorimotor cortex, basal ganglia, thalamus, and cerebellum, consistent with a diagnosis of Alzheimer’s disease. (b) Fusion of SPECT with MRI confirms the above findings and demonstrates mild gross cerebral atrophy and white matter changes suggestive of concomitant microvascular disease, a common comorbidity in this population
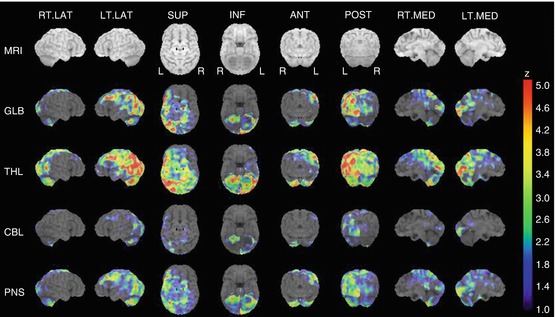
Fig. 5.2
In a 70-year-old man with dementia, 3D-SSP reconstruction of 99mTc-HMPAO brain SPECT images demonstrates generalized hypoperfusion, left greater than right, with profound hypoperfusion in bilateral parieto–occipital regions, consistent with Lewy body dementia. Involvement of the occipital cortices is often the key element differentiating this diagnosis from Alzheimer’s disease
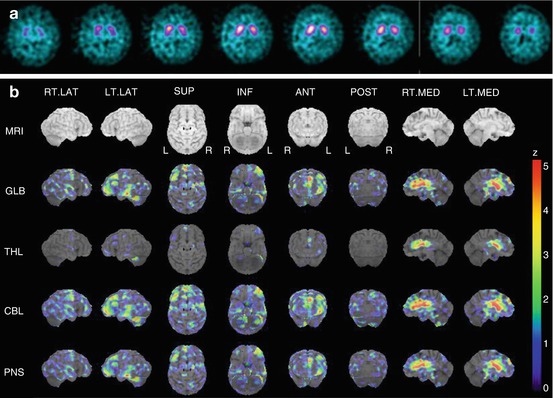
Fig. 5.3
In a 55-year-old man with rigidity, (a) transaxial DaTSCAN SPECT images show mild reduction of radiotracer uptake in the bilateral putamen, left more severe than right, confirming mild nigrostriatal degeneration typically seen in idiopathic Parkinson’s disease or related parkinsonian disorders. (b) 3D-SSP reconstruction of 99mTc-HMPAO brain SPECT demonstrates hypoperfusion in the frontal and anterior temporal lobe as well as in the upper brainstem and thalamus, providing further differentiation between the subtypes of parkinsonian disorders. Involvement of the upper brainstem seen on brain perfusion SPECT, combined with the findings of nigrostriatal degeneration seen on brain DaTSCAN, leads to the diagnosis of progressive supranuclear palsy
5.2 Radiopharmaceuticals
In order to be used for estimation of rCBF in brain perfusion SPECT imaging, a radiopharmaceutical must meet the following criteria:
1.
It must cross the blood–brain barrier (BBB).
2.
It must be retained within the brain long enough for images to be obtained and ideally will retain the initial distribution pattern of uptake without undergoing redistribution.
3.
Extraction of the radiotracer must be equal to or proportional to actual rCBF to provide an accurate estimate of blood flow.
5.2.1 133-Xenon (133Xe)
Historically, the gold standard radiopharmaceutical for rCBF quantification was the diffusible gas 133Xe. By measuring cerebral washout of the inhaled 133Xe, an absolute rCBF value can be obtained (mL/min/100 g tissue). However, this radiopharmaceutical has several limitations that have restricted its use in clinical practice. Due to the rapid clearance of 133Xe, a short acquisition time (around 5 min during the inhalation of the 133Xe gas) necessitates dynamic SPECT instrumentation, which provides high-sensitivity but low-resolution SPECT images. In addition, the low gamma-ray energy of 133Xe results in marked attenuation of deep structures, which makes this isotope less than optimal to obtain good-quality SPECT images. Finally, because 133Xe inhalation requires active cooperation, patients with respiratory or severe cognitive impairment may not be studied adequately.
Several other radiopharmaceuticals, such as 123I-labeled amines and technetium-labeled compounds, can offer higher-resolution SPECT images with the use of conventional rotating gamma cameras. However, these more commonly used radiotracers require generation of arterial input functions via blood sampling and extraction techniques to estimate rCBF (Tsuchida et al. 1997) rather than providing an absolute rCBF value.
5.2.2 123I-Isopropyliodoamphetamine (123I-IMP)
Among the initially used 123I-labeled amines, 123I-IMP (IK-3; CIS Bio-International, Gif-Sur-Yvette, France) has been the most frequently used. The typical adult dose is 5 mCi, representing an effective dose of approximately 3.53 mSv. After crossing the intact blood–brain barrier, 123I-IMP binds to amphetamine receptors on neurons. This radiopharmaceutical has good characteristics for brain perfusion SPECT. However, peak brain activity is reached as late as 20 min after injection. In addition, 123I-IMP shows redistribution over time such that there is reuptake by the cerebral cortex that is not proportional to blood flow. These latter characteristics, in addition to the high cost and poor availability of 123I-labeled compounds, have contributed to the current predominant use of technetium compounds for brain perfusion SPECT examinations.
5.2.3 99mTc-Hexamethyl Propylene Amine Oxime (HMPAO) and 99mTc-Ethyl Cysteinate Dimer (ECD)
Two virtually interchangeable technetium-labeled compounds are primarily used for brain perfusion imaging (Table 5.2): technetium-99 m (99mTc) hexamethyl propylene amine oxime (HMPAO; Ceretec™; GE Healthcare, Buckinghamshire, United Kingdom) and 99mTc-ethyl cysteinate dimer (ECD; Neurolite™; Lantheus, MA, USA). The doses used in adults are typically 20 mCi (mCi) (740 MBq) for 99mTc-HMPAO and 30 mCi (1,100 MBq) for 99mTc-ECD, representing effective doses of 0.0093 and 0.011 mSv/MBq, respectively. These compounds have several advantages over 123I-IMP. The peak brain activity is reached faster (within 2 min after injection), and there is no redistribution, so the initial tracer uptake and distribution, which are proportional to rCBF at the time of injection, remain unchanged up to at least 2 h, independent of rCBF variations occurring after the fixation time. Accordingly, the tracer can be injected while the subject is performing a specific task, when seizure activity starts, or at the moment of maximum central effect of a drug, so that SPECT images reflect the rCBF distribution at the time of injection, independent of the timing of SPECT acquisition. Thus, the tracer can be injected into the patient outside the nuclear medicine facility, and images can be acquired later providing a “snapshot” of rCBF at the time of injection. This offers an advantage over PET or MRI, because patients do not need to stay positioned in the imaging device with the head fixed while performing tasks to allow simultaneous image acquisition, as is necessary with these other modalities.
Table 5.2
99mTc-labeled radiopharmaceuticals for brain perfusion SPECT imaging
99mTc-HMPAO | 99mTc-ECD | |
---|---|---|
Radiopharmaceutical dose | 740 MBq (20 mCi) | 1,000 MBq (30 mCi) |
Effective whole body dose | 0.0093 mSv/MBq | 0.011 mSv/MBq |
Stability/labeling issues | Highly unstable in vitro; high radiochemical purity must be assured before injection because only a small proportion of injected dose will reach the brain. | Stable up to at least 4 h in vitro and freshly eluted 99mTc is not required. However, the labeling procedure is longer, taking about 30 min |
Stabilized forms of 99mTc-HMPAO using either methylene blue or cobalt chloride have recently become available and allow easier labeling and improvement of image quality by reducing background activity | ||
Maximal tracer uptake | Cerebellum | Calcarine cortex |
Response to cerebral perfusion–metabolic uncoupling | Increased uptake may occur at site of infarct, called the “luxury” perfusion phenomenon | Uptake usually remains low, more accurately reflecting hypometabolism at the site of infarct. “Luxury” perfusion is rare |
The mechanism underlying the fixation of these radiotracers within the intracellular space is due to their highly lipophilic nature which allows them to easily cross the blood–brain barrier where they undergo rapid cellular localization by diffusion. These agents are then converted into hydrophilic compounds after entering the brain, causing them to become “fixed” intracellularly. As well, the ability to obtain delayed images is beneficial in cases in which sedation must be used, as sedating agents can be administered after the injection without compromising the images. Although both 99mTc-HMPAO and 99mTc-ECD are distributed proportionally to rCBF, their retention is not completely linear with rCBF because of an initial back diffusion. Accordingly, compared to 133Xe, high blood flow may be underestimated, and low blood flow may be overestimated with both tracers (Devous et al. 1993; Payne et al. 1996).
The main differences between 99mTc-HMPAO and 99mTc-ECD relate to their in vitro stability, dosimetry, and uptake mechanism. 99mTc-HMPAO is highly unstable in vitro. Moreover, high radiochemical purity must be assured before injection, because only a small proportion of the injected dose will reach the brain. This purity is not difficult to achieve if the timing of the labeling process is done properly, as follows: time since the last generator elution <24 h; time since the 99mTc dose was eluted <2 h; and time since the cold vial was labeled with fresh 99mTc <20 min. Older labeling methods advised against mixture of 99mTc-HMPAO with blood during intravenous injection because the lipophilic compound enters into red blood cells. Stabilized forms of 99mTc-HMPAO using either methylene blue or cobalt chloride have recently become available and allow easier labeling and improvement of image quality by reducing background activity. By contrast, 99mTc-ECD is stable up to at least 4 h in vitro, and freshly eluted 99mTc is not required. However, the labeling procedure is longer, taking about 30 min.
The dosimetry for 99mTc-ECD is more favorable compared to 99mTc-HMPAO due to rapid urinary excretion of 99mTc-ECD, allowing for higher doses of 99mTc-ECD to be administered. The dosimetry is similar to lower doses of 99mTc-HMPAO if patients are instructed to force diuresis and void after the scan procedure. The use of higher doses, together with the higher gray-matter-to-white-matter ratio, contributes to the better image quality obtained with 99mTc-ECD in comparison with 99mTc-HMPAO.
In normal brain tissue, the kinetic properties are similar for 99mTc-ECD and 99mTc-HMPAO. However, in patients with brain disease, the distribution of these compounds may differ because of the biochemistry of lipophilic-to-hydrophilic conversion. In 99mTc-ECD, the hydrophilic conversion is related to de-esterification of the compound, requiring enzymatic function in the cell. For 99mTc-HMPAO, instability of the lipophilic form with intracellular conversion to hydrophilic form via glutathione interaction has been proposed. Therefore, 99mTc-ECD would have a predominant cellular–metabolic uptake mechanism of retention, while 99mTc-HMPAO would reflect only blood flow to cerebral regions. This situation accounts for slight differences in the normal SPECT pattern (e.g., the cerebellum tends to have maximal tracer uptake with HMPAO, as opposed to the calcarine cortex with ECD), and it is one of the explanations suggested for the different behavior of these tracers in subacute stroke. For episodes of cerebral perfusion–metabolic uncoupling, increased 99mTc-HMPAO uptake may occur, thus reflecting the “luxury” perfusion phenomenon, whereas 99mTc-ECD uptake usually remains low during this period, reflecting hypometabolism at the site of infarct.
5.3 Patient Preparation
For optimal imaging, patient preparation is an important consideration, with particular attention paid to environmental conditions, patient cooperation, and patient positioning.
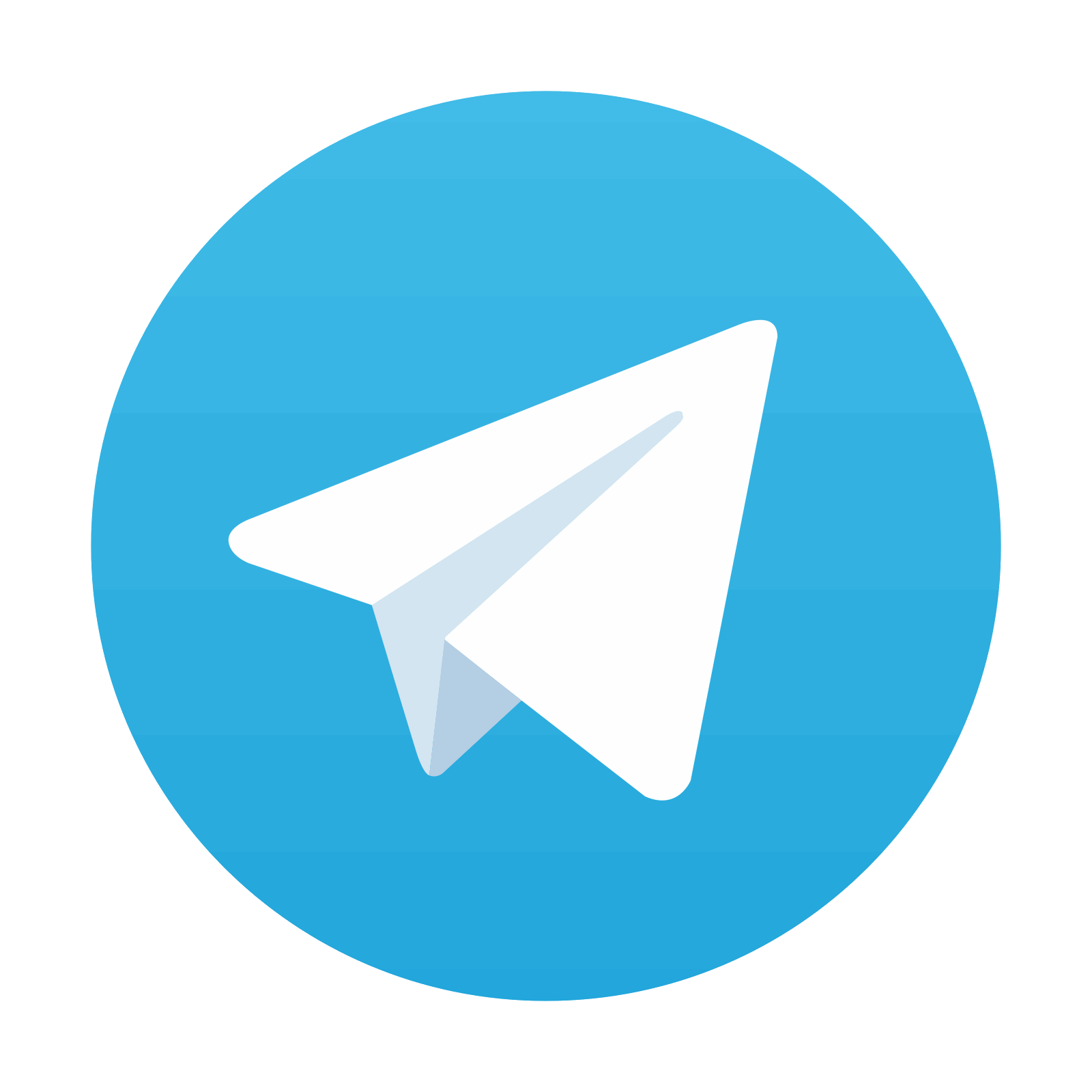
Stay updated, free articles. Join our Telegram channel

Full access? Get Clinical Tree
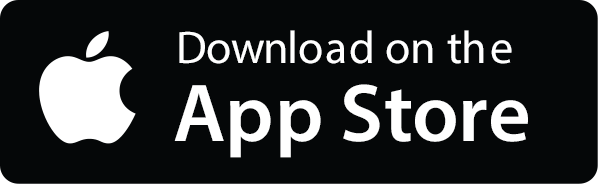
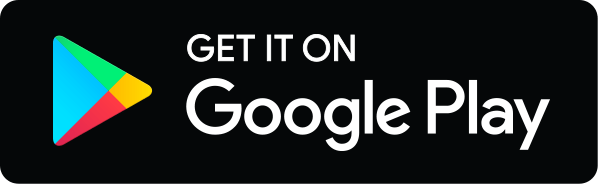