Technical Aspects
The principles of magnetization and physics allow us to create images via magnetic resonance imaging (MRI) noninvasively. In this chapter, an overview is provided on how images are created, illustrating the concepts of protons, radiofrequency (RF) excitation, T1 and T2 relaxation, image acquisition, spatial encoding, and Fourier transform analysis and k-space.
Magnetization and Protons
The nucleus of choice for imaging is hydrogen because the human body consists of an abundance of water as well as hydrogen. Every water molecule contains two hydrogen atoms, and lipids or proteins frequently contain numerous hydrogen atoms. Hydrogen atoms, also referred to as protons, precess at the Larmor frequency ( Figure 9-1 ). The Larmor frequency can be calculated from the gyromagnetic ratio and also the magnetic field strength of the magnet. All the spins comprise the net magnetization as aligned to B0. The Larmor frequency is the frequency at which the RF excitation pulse must be to alter magnetization to generate a signal and, subsequently, images.
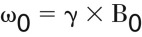
Radiofrequency Excitation
To use principles of magnetization to generate signals and anatomic information, an excitation RF pulse is generated. The frequency of this pulse will correspond to the center frequency of the system, whether it is a 1.5- or 3.0-Tesla system. The net RF pulse can change the net magnetization from 1 to 180 degrees; this angle is referred to as the flip angle.
T1 and T2 Relaxation
All proton spins reach equilibrium and in the process result in the release of measurable RF signal. In essence, after the RF excitation phase, the net magnetization realigns with the z-axis of the magnet.
There are two types of proton spin relaxations that can be described by the time constants T1 or T2. The T1 relaxation time constant refers to the recovery of longitudinal magnetization. The T2 relaxation time constant refers to the recovery of the transverse magnetization. By enabling the discrimination of anatomic structures via magnetic resonance, each tissue exhibits a different T1 or T2 relaxation time because all protons interact differently in their respective environments depending on whether the tissue is, for example, fat, muscle, or bone. T1 and T2 relaxation times are essentially determinants of tissue contrast ( Figures 9-2 and 9-3 ).
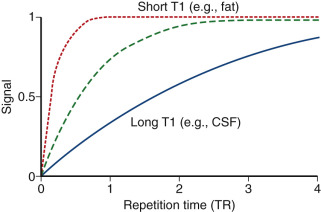
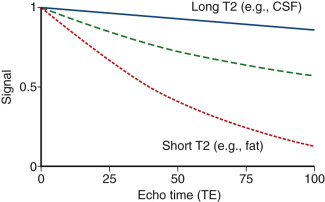
T1 relaxation, also known as spin-lattice relaxation, occurs in the z-axis of the magnet. A hydrogen proton may be bound tightly, in fat, for example, when compared with in water. More tightly bound protons release energy in a shorter period.
On the other hand, T2 relaxation, known as spin-spin relaxation, is an independent process in a perpendicular x-y plane. When the RF excitation pulse is applied, the protons are all spinning in phase. Eventually, protons will return to their out-of-phase initial pre-RF excitation state, as measured by the T2 relaxation time. The rate of dephasing is different for each tissue, resulting in further tissue contrast. T2 relaxation also occurs at a much faster rate than T1 relaxation, which can affect the design of sequences in abdominal MRI.
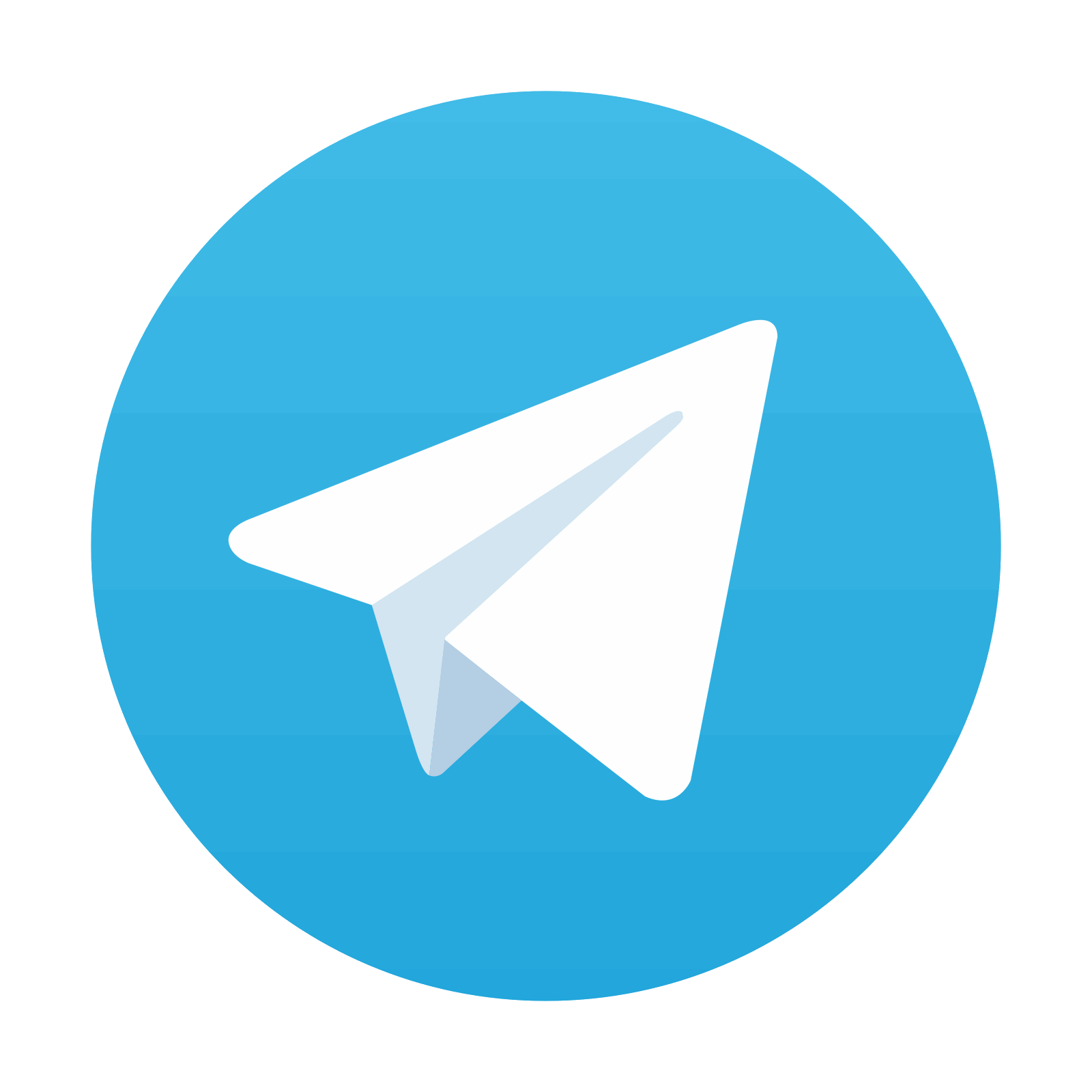
Stay updated, free articles. Join our Telegram channel

Full access? Get Clinical Tree
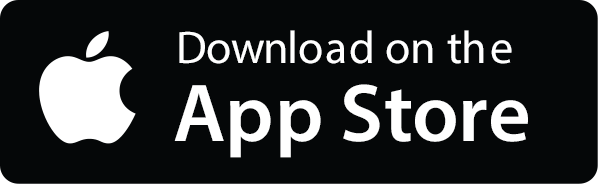
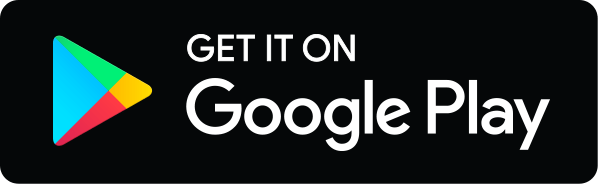