Fig. 19.1
Effect of cocaine dependence on [11C]MRB binding in humans. BP ND (binding potential) values were compared between healthy control and cocaine-dependent individuals
19.3.1.2 Duration of Abstinence Effect on NET Binding
In a subsequent study, we looked into the duration of the effect of abstinence on NET binding. Regional brain NET binding potential (BP ND, MRTM2) was measured with [11C]-MRB PET scanning in healthy controls (n = 12, 38 ± 11 years) and patients with chronic cocaine dependence (total n = 15, 43 ± 6 years, with 22 ± 7 years of cocaine use) after 1 week (n = 4), 2 weeks (n = 6), and 3–5 weeks (n = 5) of abstinence.
Our results served to reproduce our previous finding that NET is upregulated in chronic cocaine users after recent abstinence, as compared with controls. In addition, we found that subjects with cocaine dependence had higher NET BP ND values after 1 week of abstinence in the ventral lateral thalamus (70 %; P < 0.05); and that remained elevated (85 %; P < 0.01) during the second week of abstinence. By the third week, NET levels slowly declined (11 %; P = 0.60) (Lee et al. 2012). Similar significant patterns were observed in the measurement of the whole thalamus and the midbrain raphe nuclei.
The observations of an increased binding of [11C]MRB in our in vivo PET imaging studies of chronic COC subjects is consistent with previously published in vitro data in rodents, rhesus monkeys, and postmortem human brains (Beveridge et al. 2005; Macey et al. 2003; Mash et al. 2005). Our results are also in agreement with an in vitro study in which an upregulation of NET mRNA in the LC was observed in rats following chronic binge administration of cocaine (Burchett and Bannon 1997). Interestingly, in this rat study an alteration was observed in an opposite direction for the other two monoamine transporters, i.e., a decrease in SERT mRNA (in raphe) and DAT mRNA (in midbrain) following a binge regimen of chronic COC.
19.3.2 NET in ADHD
Attention-deficit hyperactivity disorder (ADHD), a complex developmental behavioral and cognitive disorder that affects approximately 3–7 % of school-age boys and 2–4 % of adults, is associated with impaired academic and occupational achievements, problem behaviors, increased risk for accidents, and substance abuse (American Psychiatric Press 1994; Barkley 1997; Dopheide and Pliszka 2009). The core features of ADHD – inattention, hyperactivity, and impulsivity – can be attributed to dysfunction in neural systems that regulate attention, executive function, motor control, and reward (Makris et al. 2009; Bush 2010); however, effective treatments remain elusive and the neurochemical mechanisms of this disorder are poorly understood (Bush 2010). The three therapeutic classes of pharmacologic treatments for ADHD – (a) psychostimulants (e.g., methylphenidate hydrochloride, MPH), (b) non-stimulant catecholamine reuptake inhibitors (e.g., atomoxetine, ATX), and (c) α2 adrenergic receptor agonists (e.g., guanfacine) – all modulate DA and NE neurotransmission (Makris et al. 2009; Bush 2010; Biederman et al. 2008; Dickstein et al. 2006; Madras et al. 2005). Small changes in extracellular NE or DA concentration affect networks of pyramidal cells in the prefrontal cortex (PFC) which regulates and sustains attention. The beneficial effects of DA occur at D1 receptors, while those of NE are believed to occur at α2 receptors (Arnsten 2009). Here we present several of our translational research studies of drug occupancy designed directly towards developing a better understanding of the mechanisms that contribute to the therapeutic effects of pharmacologic treatments for ADHD.
19.3.2.1 ATX Occupancy Study on NET in Humans and Nonhuman Primates
Atomoxetine (ATX, Strattera), a potent NET reuptake inhibitor (K i ~5 nM), is the first non-stimulant drug that was approved for the treatment of ADHD (Bymaster et al. 2002; Michelson et al. 2001; Swanson and Volkow 2009; Chamberlain et al. 2007a; Volkow et al. 2001; Del Campo et al. 2011; Arnsten 2006; Spencer et al. 2002). When [11C]MRB was initially tested in a drug occupancy study in humans using atomoxetine (ATX), a reduction in [11C]MRB binding was observed, but there was no obvious dose-dependent effect (Logan et al. 2007). One hypothesis was that the doses of ATX examined in the human PET study (oral, 25, 50 or 100 mg), though clinically relevant, were too high. That is, the three tested doses all reached saturation, the plateau portion of the corresponding dose-occupancy curve, and as a result there was no obvious dose-dependent effect. Another occupancy study of ATX using [18F]FMeNER-D2 in rhesus monkeys showed a reasonable dose-dependent occupancy, though only in one of the two monkeys studied and the IC50 (or ED50) was not determined (Seneca et al. 2006).
Thus, the suitability of [11C]MRB for occupancy studies was further evaluated by carrying out investigations in a nonhuman primate model (rhesus macaque) using lower doses of ATX than those used in the previous PET study with [11C]MRB (Logan et al. 2007) and with a continuous ATX infusion paradigm to maintain a constant concentration of ATX in the plasma during the PET acquisition time (120 min). Ten PET studies were performed in three anesthetized rhesus monkeys following an infusion of ATX or placebo.
For each PET study, two infusion rates were used as follows: ATX infusion at a “loading” rate was first given for 1 h starting at 2 h before the injection of [11C]MRB; then ATX was given at a “maintenance” rate (37.5 % lower than the “loading” rate) at 1 h before the injection of [11C]MRB. This infusion rate was maintained until the end of the 2-h PET scan (i.e., 3 h at “maintenance” rate) (Fig. 19.2). Three doses of “maintenance” rate were used: 0.01 mg/kg/h (low dose), 0.03 mg/kg/h (medium dose), and 0.09 mg/kg/h (high dose) (i.e., each monkey had four PET scans, including the baseline scan with placebo). The design was to have each monkey have four PET scans on two study days. These two study days were scheduled at least 3 weeks apart to allow recovery from the effect of the anesthesia and blood drawing. On each study day, two PET scans were acquired, beginning 4 h apart. The first study day consisted of the baseline and medium-dose scans, the second study day consisted of the low- and high-dose scans.
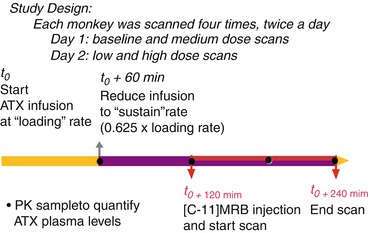
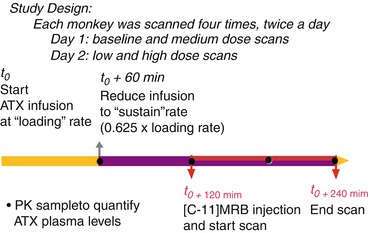
Fig. 19.2
Study design for the 2-day, four-dose studies to measure ATX occupancy of NET in nonhuman primates using [11C]MRB
A dose-dependent reduction of [11C]MRB volume of distribution was observed after correction for [11C]MRB plasma free fraction. Although the slow clearance of [11C]MRB in NET-rich regions such as the thalamus posed a concern for kinetic modeling, the combination of the suitably designed infusion study paradigm, and refined kinetic modeling methods, an unequivocal dose-dependent occupancy of atomoxetine was obtained (Fig. 19.3) clearly demonstrating the suitability of [11C]MRB for drug occupancy studies of the NET (Gallezot et al. 2010).
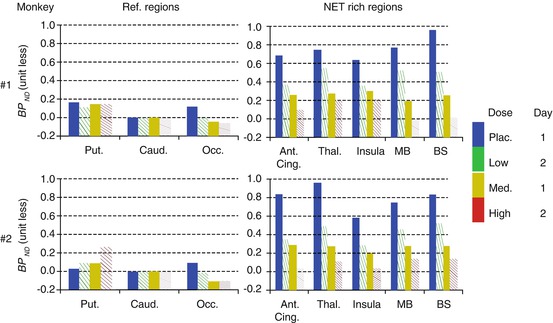
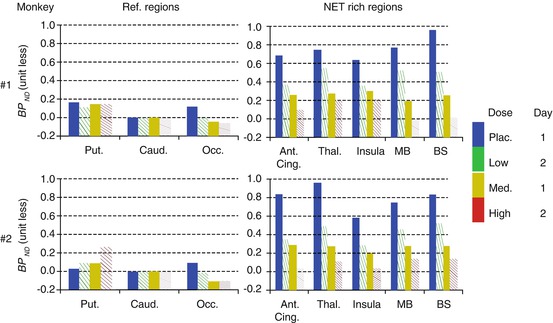
Fig. 19.3
Dose-dependent occupancy of ATX in NET-rich regions in two monkeys using [11C]MRB after the placebo (Plac.), low, medium, and high ATX doses. BP ND (binding potential) values were measured for NET-poor regions (putamen (put.) and occipital cortex (Occ.)) and NET-rich regions (anterior cingulate (Ant. Cing.), thalamus (Thal.), insular, midbrain (MB), and brain stem (BS)), using caudate as a reference region
Based on this study, ATX IC 50 was estimated to be 32 ± 13 ng/mL plasma, corresponding to an effective dose (ED 50) of 0.13 mg/kg, which is much lower than the therapeutic dose of ATX in ADHD (1.0–1.5 mg/kg). In the previous ATX occupancy study in humans, three clinically relevant doses were used (oral, 25, 50, or 100 mg), the blood levels of ATX (in ng/mL plasma) averaged over subjects were 116 (25 mg ATX), 206 (50 mg ATX), and 421 (100 mg ATX), which were significantly higher than the estimated IC 50 for ATX in plasma (~30 ng/mL). This suggests that the lack of an obvious dose-dependent effect in the previous study was indeed because the three clinically relevant doses all reached saturation with over 78–93 % occupancy, i.e., the plateau portion of the corresponding dose-occupancy curve (Logan et al. 2007). Taken together, our results clearly indicate the importance of dose selection for PET occupancy studies, especially when observation of dose-dependency of the study drug is one of the goals for the outcome measurement.
19.3.2.2 ATX Occupancy Study on SERT, Implication on Treatment of Depression and ADHD
The development of ATX and its approval for the treatment of ADHD by the Food and Drug Administration (Faraone et al. 2005) was based on the rationale that it selectively blocks the NET (Barton 2005) and increases the availability of intrasynaptic NE, resulting in the improvement of high-level cognitive functions that are often impaired in ADHD, such as working memory and inhibitory response control (Robbins and Arnsten 2009). In principle, ATX may offer clinical advantages by virtue of its limited effects on subcortical dopamine, which is thought to be responsible for the abuse potential of psychostimulant treatment (Volkow 2006). Furthermore, this single NE pathway of drug mechanism may facilitate further drug research and development.
The noradrenergic action also exerts an important clinical effect in different antidepressant classes such as desipramine and nortriptyline (tricyclics with prevalent noradrenergic effect). Thus, ATX has also been used to improve responses to treatment of depression (Dell’Osso et al. 2010; O’Sullivan et al. 2009).
Serendipitously, we discovered that ATX displayed a blocking effect similar to fluoxetine (a selective SERT inhibitor) on binding of [11C]DASB (Ding and Fowler 2005), a selective SERT ligand (Houle et al. 2000) (Fig. 19.4). These results suggested that ATX not only binds to NET but also binds to SERT with high affinity. To date, whether the therapeutic effects of ATX in the treatment of either ADHD or depression are due to inhibitory effects on one (NET) or two transporters (NET and SERT) is not known. Therefore, we took on this investigation by carrying out comparative occupancy studies with [11C]MRB (a NET ligand, as described above) (Gallezot et al. 2008, 2010) and [11C]AFM (a SERT ligand) (Huang et al. 2002) to evaluate the in vivo IC50 of ATX for both NET and SERT in nonhuman primates using PET.
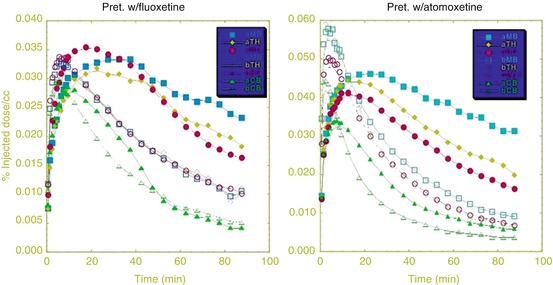
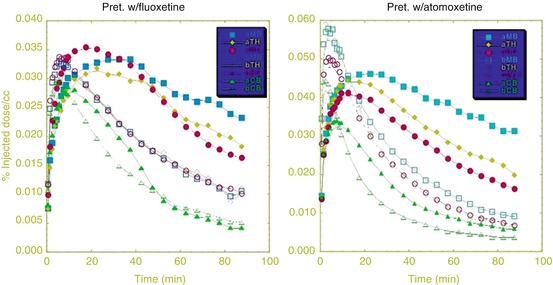
Fig. 19.4
ATX displayed a blocking effect similar to fluoxetine (a selective SERT inhibitor) on binding of [11C]DASB (a selective SERT ligand), questioning the in vivo selectivity of ATX
The continuous infusion protocol with [11C]AFM was similar to our previous study with [11C]MRB (see above) (Gallezot et al. 2008, 2010). Two monkeys were scanned with maintenance rates: 0.045 mg/kg/h (low dose), 0.135 mg/kg/h (medium dose), and 0.405 mg/kg/h (high dose). One monkey was scanned with the higher maintenance rates: 0.135 mg/kg/h (medium dose), 0.405 mg/kg/h (high dose), and 1.054 mg/kg/h (very-high dose) (details of this study will be published elsewhere).
19.3.2.3 Summary of the Comparative Occupancy Studies of ATX for NET (Using [11C]MRB) and SERT (Using [11C]AFM)
ATX displayed dose-dependent occupancy of both NET and SERT, with a higher occupancy of NET: IC 50 of 31 ± 10 and 99 ± 21 ng/mL plasma for NET and SERT, respectively. At a clinically relevant dose (1.8 mg/kg, ~600 ng/mL plasma), ATX would occupy >95 % of NET and occupy >85 % of SERT. It is worth noting that this value of 3.2 for the selectivity ratio of ATX towards NET vs. SERT measured via in vivo PET imaging in nonhuman primates (NHP) is similar to a value of 4.5 for the selectivity ratio derived from the previously reported in vitro Kd values using human NET and SERT tissue homogenates (8.9 and 2 nM for SERT and NET, respectively) (Tatsumi et al. 1997). Based on these data, we propose that ATX at clinically relevant doses occupies high proportions of both NET and SERT. Thus, the interpretation of the therapeutic mode of action of ATX for treatment of depression and ADHD may be more complex than selective blockade of the NET.
19.3.2.4 The Mechanisms of Action of ATX in ADHD and Depression
It has been shown that response inhibition (the ability to exert high-level inhibitory control over motor responses so as to suppress unwanted actions) is impaired in subjects with ADHD and is one of the most robust cognitive findings in ADHD research (Lijffijt et al. 2005). Response inhibition (aka impulsivity) can be measured by the stop-signal task (SST), and quantified by the stop-signal reaction time (SSRT), an estimate of the time taken to inhibit the prepotent motor response (Aron et al. 2003a). The research group at Cambridge, UK, found that single doses of atomoxetine (60 mg) were able to improve response inhibition in humans and in rats (Chamberlain et al. 2006, 2007a; Eagle et al. 2008; Bari et al. 2009). By contrast, serotonin manipulations with citalopram, a SERT blocker (Chamberlain et al. 2006; Del Campo et al. 2011), or with buspirone, a serotonin 5-HT1A receptor agonist (Chamberlain et al. 2006, 2007b), appear to have no behavioral effects on the SSRT. These results suggest that atomoxetine enhances stopping selectively via actions on NE uptake and that NET drugs may be more suited to ameliorating impaired response inhibition as an ADHD therapeutic target.
Taken together, the inhibitory effect of ATX on NET plays a crucial role on its therapeutic effect on ADHD, while the inhibitory effect of ATX on SERT may play a more important role on its therapeutic effect on depression. Further studies to elucidate the actual relationship between the drug mechanisms and drug therapeutic effects are needed.
19.3.2.5 MPH Occupancy Study on NET in Humans
The effects of MPH on the DA system have been studied extensively. Our previous PET studies have demonstrated that MPH’s pharmacological effects reside in d–threo-MPH, the active enantiomer (Ding et al. 1997), and that at clinical doses MPH significantly occupies DAT in vivo in humans (Swanson and Volkow 2009; Volkow et al. 1998), suggesting that DA reuptake inhibition may be an important therapeutic mechanism in ADHD. However, MPH has shown higher in vitro affinity for NET than DAT (K i = 38 nM vs. 193 nM) (Eshleman et al. 1999). The potential role of NE in ADHD was supported by animal and human data (Biederman et al. 2008). Low doses of MPH increase levels of NE in the PFC in animals (Berridge et al. 2006), and low doses of MPH improve performance of PFC tasks in subjects with ADHD (Aron et al. 2003b). The absence of a suitable NET tracer has delayed similar in vivo PET studies of NET until now.
We used [11C]MRB to determine the effective dose 50 (ED50) of MPH for NET (Hannestad et al. 2010). We first conducted a preliminary duration study to determine how much time it takes from oral MPH administration to maximum occupancy of brain NET. As peak displacement of [11C]MRB by MPH (oral, 40 mg, i.e., 0.35–0.55 mg/kg, the average clinical maintenance dose of MPH) was achieved in 75 min, we chose 75 min as the time between oral MPH dosing and [11C]MRB injection for the subsequent occupancy study. Based on our previous experience with ATX occupancy studies in humans, dose selection is critical if dose-dependent occupancy is one of the goals to be achieved. Thus, in this within-subject design study, healthy subjects (n = 11) received oral, single-blind placebo and 2.5, 10, and 40 mg of MPH 75 min prior to [11C]MRB injection. Dynamic PET imaging was performed for 2 h with HRRT. Three regions with known lowest density of NET (occipital cortex, caudate, and putamen) were investigated (Logan et al. 2005) further as reference regions by comparing the distribution volume (V T) in these three regions between the placebo and 40 mg MPH conditions (Hannestad et al. 2010). We found no significant change of V T (or V T/f P)(f P: plasma free fraction) in these three regions between the placebo and 40 mg MPH conditions, and the putamen and occipital had similar V T values. Thus, the multilinear reference tissue model with occipital cortex as the reference region was used to estimate binding potential (BP ND ) in the thalamus and other NET-rich regions.
We found that BP ND was reduced by MPH in a dose-dependent manner in the thalamus and other NET-rich regions (Fig. 19.5). The global ED50 was estimated to be 0.14 mg/kg; therefore the average clinical maintenance dose of MPH (0.35–0.55 mg/kg) produces 70–80 % occupancy of NET. This is the first in vivo study in humans showing that clinically relevant doses of MPH occupy significant levels of NET, despite the fact that MPH has been used for the treatment of ADHD for decades. The ED50 is lower than that for DAT (0.25 mg/kg), suggesting the potential relevance of NET inhibition in the therapeutic effects of MPH in ADHD.
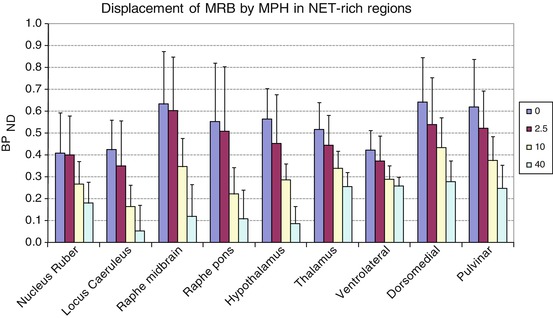
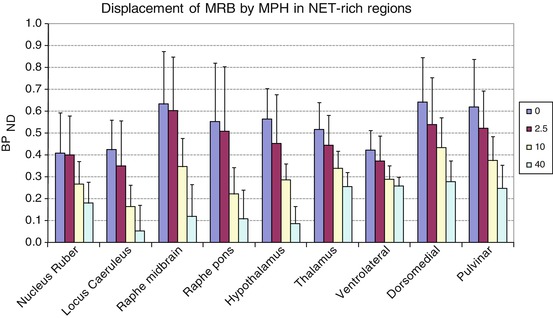
Fig. 19.5
BP ND values were reduced by methylphenidate in a dose-dependent manner in NET-rich regions after four doses of oral methylphenidate (0, 2.5, 10, 40 mg)
Previous studies also suggested that effects on delayed responding and working memory are mediated by noradrenergic afferents from LC (the highest NET density region) to PFC (Biederman et al. 2008; Solanto 1998). However, limited studies were able to link brain process with attention and ADHD. It has also been shown that the temporal, parietal, and prefrontal (PFC) association cortices mediate different aspects of attention and that treatments for ADHD enhance catecholamine actions in PFC and improve PFC functions (Arnsten 2009). Although the density of NET in PFC is very low, we were able to detect dose-dependent displacement of [11C]MRB by MPH in two cortical regions in which NET densities were high enough: paracentral lobule and the supplementary motor area (SMA) (Fig. 19.6), which are areas involved in the response to MPH and inhibition control during cognitive tasks, respectively (Hannestad et al. 2010; Volkow et al. 2008; Li et al. 2006). Although we cannot assess the effect of MPH in the PFC directly, our assumption is that the global ED50 calculated using NET-rich regions, including the neocortical paracentral lobule, is similar to the ED50 in regions with lower NET density, such as the PFC. It is also important to fill the gap by combining PET (offers specificity) and other modality such as fMRI (offers spatial and temporal resolution) to explore the underlying molecular processes.
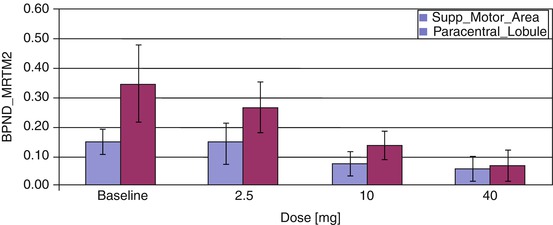
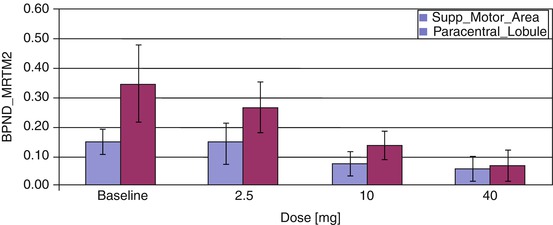
Fig. 19.6
Dose-dependent displacement of [11C]MRB by methylphenidate in two cortical regions (paracentral lobule and the supplementary motor area (SMA)) was also detected after four doses of oral methylphenidate (0, 2.5, 10, 40 mg). MTRM2 multilinear reference tissue model with two parameters (Ichise et al. 2003)
19.3.3 NET in PTSD
Termination of the action of NE on adrenergic receptors is accomplished primarily by NE reuptake into the presynaptic terminal via the NE transporters (NET). Animal studies and postmortem studies in humans have implicated reductions in NET expression in depression, which is often comorbid with PTSD. While acute stress does not alter NET density, there is evidence from rodent studies that repeated stress decreases NET density in the locus coeruleus (LC) and limbic brain regions (Zafar et al. 1997). These important findings, however, have not yet been confirmed in humans using in vivo methods because brain imaging of NET in living humans has been hampered for over 20 years by the lack of suitable radioligands. Given that the NET is a suitable target for antidepressants and the established role of noradrenergic mechanisms in PTSD, additional research on the distribution and function of NET in the brain of patients with PTSD is important to better understand the hypothesized dysregulations in NE function in PTSD. Thus, the hypothesis that lower NET density may be related to the development of mood and anxiety disorders (Moron et al. 2002; Liprando et al. 2004; Miner et al. 2006; Arnsten and Li 2005) was investigated using the most promising NET ligand, [11C]MRB. In order to evaluate whether trauma exposure and PTSD would be associated with lower NET density in the locus coeruleus (LC), we conducted PET studies using [11C]MRB in three groups of individuals: 18 healthy adults (healthy controls: HC), 16 adults exposed to trauma who did not develop PTSD (trauma control: TC), and 22 adults exposed to trauma who developed PTSD. As a secondary aim, we evaluated the relation between NET availability in LC and a contemporary phenotypic model of PTSD symptomatology, namely, a five factor model comprised of reexperiencing, avoidance, numbing, dysphoric arousal, and anxious arousal (Pietrzak et al. 2012; Armour et al. 2012; Elhai et al. 2011). Our study results revealed that both the PTSD group and the TC group had significantly lower NET density than the HC group (41 % lower with Cohen’s d = 1.04 for the PTSD group and 31 % lower with Cohen’s d = 0.77 for the TC group, respectively), while NET density was not significantly different between the TC and PTSD groups (15 % lower in PTSD versus TC group, Cohen’s d = 0.27). In the PTSD group, NET density in the LC was independently positively associated with the severity of anxious arousal (i.e., hypervigilance symptoms, r = 0.52), but not with any of the other symptom clusters. Results of this study suggest that TC and PTSD are associated with significant reductions in NET availability in LC and that NET density in this brain region is associated with increased severity of anxious arousal symptoms among individuals with PTSD (Pietrzak et al. 2013).
19.3.4 NET in Alcohol Dependence
We investigated the role of NET in alcohol dependence in human subjects, in vivo, using [11C]MRB and PET. Unmedicated, recently abstinent (4–6 weeks abstinence to compensate for acute alcohol withdrawal effects) non-obese men and women meeting DSM-IV (First et al. 1996) criteria for current alcohol dependence (n = 15) and age- and sex-matched healthy control subjects (n = 15) were studied and compared. Our hypothesis was that in areas of highest NET density, we would detect lower ligand binding commensurate with NET downregulation due to chronic alcohol exposure. We identified two brain regions in alcoholics, the thalamus and locus coeruleus, which exhibited reduced NET binding potential (BP ND ). We have postulated that these regions are involved in alcohol craving, acquisition, and ingestion and that they may serve as biomarkers of altered NET expression in alcohol dependence disorders and as potential therapeutic targets for drug development (unpublished results; details of this study will be published elsewhere).
19.3.5 NET in Obesity and Brown Adipose tissue (Brown Fat)
We have conducted studies in humans to investigate the role of NET in obesity. The preliminary data suggest that there is lower NET binding in the thalamus (including pulvinar) in obese subjects (body mass index (BMI) of 34.7 ± 2.6) as compared to matched lean subjects (BMI of 23.1 ± 1.4) (unpublished results, details of this study will be published elsewhere).
Obesity is characterized by impaired energy expenditure associated with adaptive thermogenesis (Camastra et al. 1999; Golay et al. 1986; Yeckel et al. 2009, Jequier and Schutz 1985). Brown adipose tissue (BAT, brown fat), one of the primary tissues responsible for adaptive non-shivering thermogenesis in mammals (Wijers et al. 2009), was previously thought to disappear in humans as part of development into adulthood. The recent confirmation that BAT still exists in adult humans provides a renewed interest in this tissue; however, the relative importance of BAT activity to adult human whole-body energy expenditure is still unknown. Lessons learned from steps taken to reduce undesirable FDG-labeling during many false-positive clinical scans (Nedergaard et al. 2007; Hany et al. 2002; Christensen et al. 2006) demonstrate that repeat scans after beta-adrenergic blockade eliminate the interfering BAT signal (Agrawal et al. 2009). These data suggest the presence of strong sympathetic nervous system (SNS) activation of BAT in humans and the potential involvement of the noradrenergic system in the regulation of BAT activity. Thus, in addition to a NET obesity study in brain, we also carried out a pilot study to look into the NET involvement in both central and peripheral SNS function and to determine whether BAT can be visualized with NET-PET imaging and whether NET binding in BAT can be quantified under both basal and activated conditions in humans.
Based on [18F]FDG-PET imaging data, [18F]FDG-imaging requires subjects under cold stimulation during the PET imaging, and BAT does not appear to be visualized with [18F]FDG-PET at room temperature (Saito et al. 2009; van Marken Lichtenbelt et al. 2009). The BAT metabolic activity is related to age, gender, BMI, cold exposure and medications (Cypess et al. 2009). Thus, the [18F]FDG-PET approach may not be suitable for imaging BAT at the basal state, i.e., room temperature. Since BAT activation appears to be dynamic, expressing large variability due to thermal sensitivity, individual differences in BAT activation due to variations in thermal response are likely to make comparisons between obese and normal-weight subjects difficult. Moreover, [18F]FDG is not specific to BAT nor is glucose the primary substrate for BAT heat production. The [18F]FDG-PET strategy will not address regulatory mechanisms that are related to the specific SNS modulation features of BAT, which are likely to be key features to understanding potential dysfunction. Therefore, it is important to develop new and more specific methods to address both the plasticity of BAT activation and the basal characteristics that do not require stimulation or that could alternatively be used in combination with strategies that highlight BAT stimulated activity.
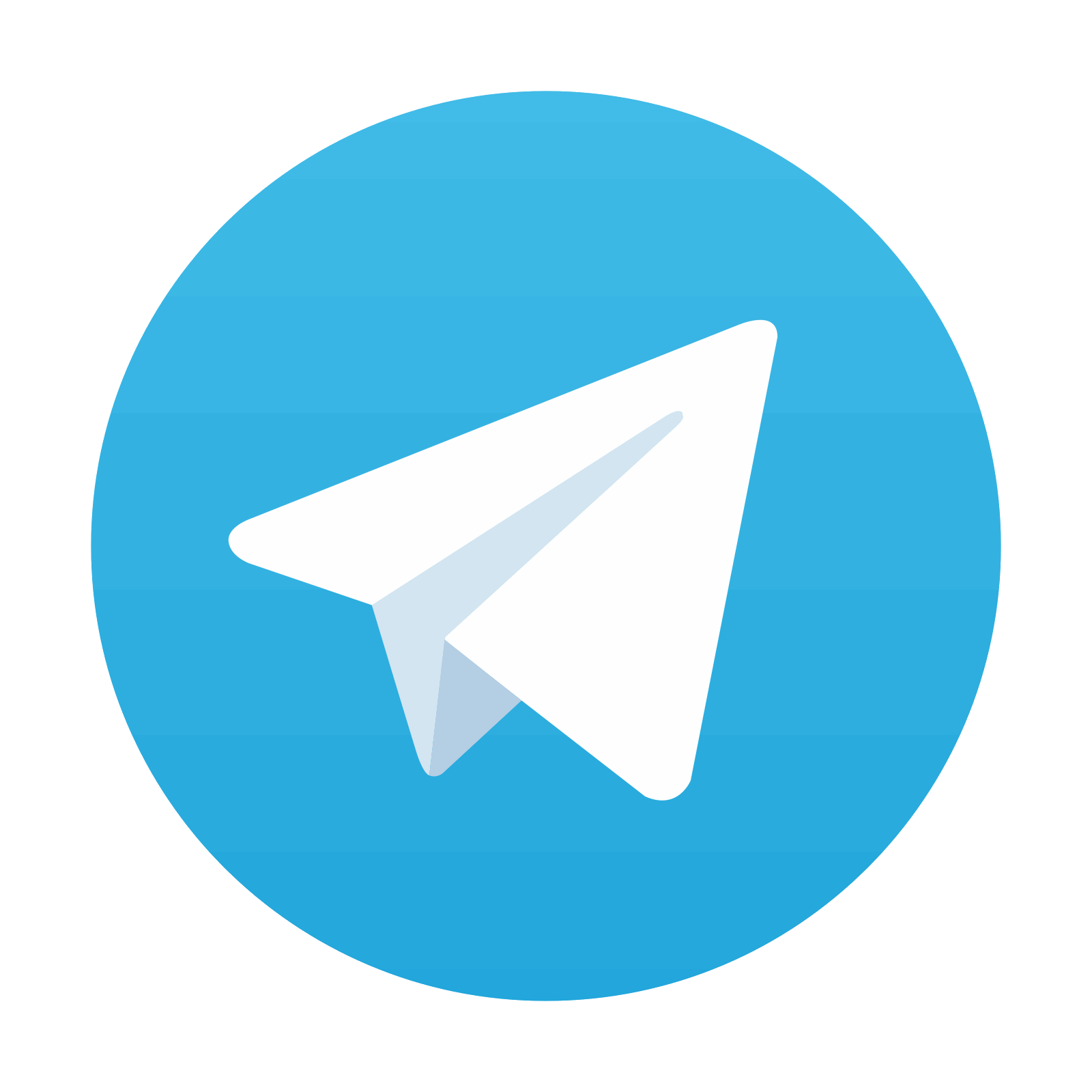
Stay updated, free articles. Join our Telegram channel

Full access? Get Clinical Tree
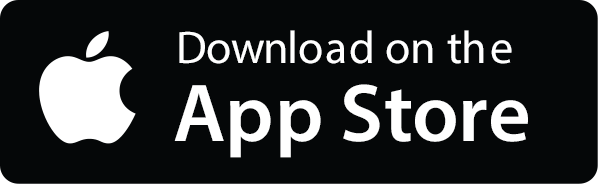
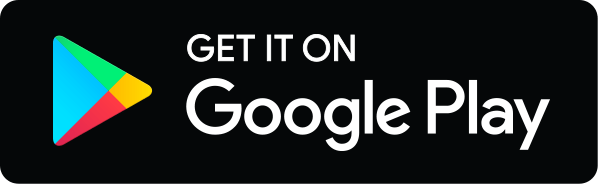