21 The concept of intraoperative magnetic resonance imaging (MRI) guidance was first envisioned in 1990 in the Image-Guided Therapy (IGT) Program at the Brigham and Women’s Hospital, Harvard Medical School, Boston, MA.1 This program, a collaboration with General Electric (General Electric Medical Systems, Waukesha, WI), introduced the Signa SP MRI with the nickname “double-doughnut” that refers to its special open configuration.2 The first of its kind, this scanner was located in a fully functional operating room (OR) suitable for open neurosurgery. During interventional procedures and surgeries the patient stayed on the MRI table that also served as an OR table. This MRI configuration based on novel magnet design and technology complemented and augmented the neurosurgeons’ vision, enabling them to see beyond and “under” the exposed surfaces and to discriminate tumors from normal tissue. MRI guidance not only improves localization and targeting of tumors, but also, within the limits of MRI’s contrast and spatial resolution, identifies tumor margins—a critical step to ensure complete tumor removal.3–5 Since then real-time or iterative intraoperative MR imaging (iMRI) at the Brigham has guided over 3,000 surgical and interventional procedures.6 The procedures, among others, have included open brain surgeries, MRI-guided thermal ablations, and brain biopsies as well as nonneurosurgical procedures such as endoscopic sinus surgery and prostate brachytherapy.7–11 Coupled with new therapy devices and surgical navigation, these procedures have become systems that have taken on a pivotal role in opening new directions in the surgical management of cancer in general and in neurosurgery of primary brain tumors in particular.3 Within a short period, many new interventional and/or intraoperative MRI centers were established around the world, and iMRI became widely accepted in neurosurgery.12–17 The fundamental goal of neurosurgery is to target, access, and address the lesion without causing significant damage to normal brain tissue or vascular structures. The overall concern is the preservation of neurologic function, an outcome that requires precise delineation of functional anatomy and correct definition of the resection target volume. To improve outcomes and decrease invasiveness, neurosurgical treatment is increasingly taking advantage of rapid developments in imaging and data presentation. The combining of imaging and neurosurgery has a long history. In fact, neurosurgical progress parallels the developments of improved visualization. From early efforts with ventriculography and angiography to the introduction of the operating microscope to the development of cross-sectional imaging and the recent widespread adoption of neuronavigation, neurosurgeons have dramatically improved the precision, safety, and effectiveness of brain surgery for the treatment of many conditions. iMRI, for example, provides unprecedented intraoperative visualization capabilities. It has enhanced the surgeon’s ability to accurately define margins and their relationship to critical structures during the resection of brain tumors. Over the past 20 years, specialized neuronavigational tools have been developed to assist the surgeon in these endeavors; the development of MRI-guided navigation systems, for instance, represents a significant improvement in the surgical treatment of various intracranial lesions. In recent years, several groups have proposed integrating functional data into the neuronavigation system.18,19 Since its introduction in the early 1990s, the field of intra-operative and interventional MRI has grown and changed significantly. It has become increasingly obvious that full access open MRI, like the Signa SP, is not the only solution for guiding procedures. Indeed, numerous procedures require higher-field strength and more advanced image acquisition technology than can be accomplished with an open-magnet configuration. Several groups using iMRI for neurosurgery have moved to a higher-field strength using closed-bore 1.5 tesla (T) and even 3.0 T MRI systems.20,21 Such a configuration does lack direct access, prohibiting real-time intraoperative imaging for open surgeries and certain minimally invasive procedures. Nevertheless, when the patient is moved in and out of the closed-bore magnet, image updates can be obtained during open procedures. In addition, within the closed bore of the high-field magnet, patients can be monitored in real-time during certain catheter-based applications and most thermal ablations. The trend in iMRI, especially in neurosurgery, is a move toward more widespread deployment of the high-field magnet as well as use of more advanced imaging techniques presently not feasible for less-expensive low- and midfield systems. Activities in the field of iMRI focus on moving most of the procedures (open surgeries, thermal ablations) to the advanced 3.0 T imaging platform to achieve faster and more flexible image acquisition that, in turn, will improve localization, targeting, monitoring, and therapy control for those procedures adapted to this new application environment. The major goal of interventional or intraoperative MRI is to provide near real-time dynamic, interactive image guidance for surgical and percutaneous interventional procedures. Today, this type of image guidance is not available for open surgeries but can be provided for catheter-based or endoscopic procedures. In neurosurgery, iMRI has been successfully developed and implemented for multiple interventional and surgical procedures, including biopsies and the placement of electrodes, craniotomies for image-guided resection, or the treatment of various intracranial tumors and epileptogenic foci, intracranial cyst drainages, and thermal ablations for malignant and benign tumors.3–5,7,16,22–33 In the future, potential applications will expand, and the use of iMRI guidance will be utilized for functional neurosurgery and for the treatment of benign brain tumors, especially those at the skull base as well as neurovascular abnormalities and various diseases of the spine. As far as the original use of iMRI for glioma surgery is concerned, that surgery’s goal of obtaining as complete a resection as possible can only be accomplished with better techniques to detect the full extent of infiltrative tumor and more detailed information about the functional and structural anatomy of the brain tissue that surrounds the tumor. As interventional MRI matures, its improved visualization opens doors to new treatment approaches. Once tools, tissue, and treatment effects can be monitored in near-real or real-time, numerous treatment approaches can be developed that do not require direct visualization and that represent a move away from open surgery. This major progress and expansion requires the development of new imaging methods, navigational techniques, surgical instruments, and interventional tools that will spawn other approaches to transform open surgeries into less-invasive procedures. One of the most promising, noninvasive thermal ablation using MRI-guided focused ultrasound (US), or MRgFUS as it has been named, has great potential to change neurosurgical practice. The use of new molecular imaging techniques may improve the detection of tumor margins, but that development requires the use of modalities other than MRI. This multimodality environment suited to molecular imaging-guided surgeries should include nuclear and optical imaging modalities and/or navigation-assisted handheld probes that have higher sensitivity for tumor detection. The integration of functional (fMRI) and diffusion tensor imaging (DTI) into surgical planning and intraoperative guidance systems will further improve the preservation of neurologic functions and, ultimately, the completeness of resections. Most open neurosurgery, whether using iMRI or not, aims to remove brain tumors. The original, and still compelling, reason for using intraoperative imaging during neurosurgery is to compensate for brain-shift. Navigational systems in the operating room acquire preoperatively a single three-dimensional (3D) image database, limiting the detection time window. As surgery progresses from craniotomy to resection, this preoperative information becomes less and less representative of the anatomy. An iMRI can update this preoperative dataset and provide more accurate guidance based on the actual anatomy. Although this improved navigation can be helpful for tumor removal, in some cases, intraoperative imaging may be restricted to the end of the surgery, when, before closing, a single imaging dataset is acquired to check the completeness of resection by looking for residual, MRI-visible tumor tissue. This so-called tumor control method is a practical and well-accepted use of iMRI. However, such a single imaging session at the completion of surgery cannot take full advantage of the information from iMRI that can provide more frequent correct brain-shift-compensated guidance for the safe removal of tumors.33 The early success of iMRI was due in large part to its ability to detect residual tumor prior to the end of surgery rather than postoperatively. At that time, most intraoperative sites did not have the computerized tools necessary to use the platform as a complex navigational system. In the future, iMRI will be extensively used as a de facto image-guidance and navigation system to capitalize on the benefits of image guidance to most tumor surgeries. Eventually, iMRI will fundamentally change the way surgery is performed by allowing the adoption of less-invasive surgical approaches that use novel technologies. Given its capabilities, iMRI can benefit patients who have cerebral gliomas for whom neurosurgical resection is the primary therapeutic intervention.34 Gliomas are the most common types of primary malignant brain tumors; rarely circumscribed or well-defined, they grow diffusely within the brain. An interventional MRI scanner allows for the near-total removal of this type of tumor. That outcome can be achieved, although it is difficult due to the inherent uncertainty in visually distinguishing glial tumor tissue from adjacent brain tissue. The goal of minimizing the number of infiltrating glioma cells in the adjacent brain tissue is desirable for several reasons: prolonged survival, prolonged time to malignant progression, decreased risk of seizures, and eligibility for future oncologic therapies. Use of iMRI can achieve more complete tumor removal than surgery using just the human eye due to the higher sensitivity of the MRI in detecting and differentiating low-grade glial neoplasms from surrounding, often gliotic brain tissue.3–5 Maximizing the resection of glial tumor tissue while minimizing neurologic deficits is challenging in part because functional brain tissue may reside close to or even within gliomas, and the inadvertent removal of tumor-infiltrated, but functioning brain tissue can result in neurologic deficits.35 iMRI increases the chances of preserving this functional brain tissue. Tissue that remains functional despite being edematous and infiltrated to an unspecified extent by glioma cells may be seen on nonenhancing T1-weighted images as hypointense areas and on T2-weighted images as hyperintense areas. Thus, even when resections are limited to within the MRI abnormality, postoperative neurologic deficits can result, suggesting that functioning white matter fiber tracts may exist within the tumor. Therefore, maximal resection, complicated by brain-shift, requires precise understanding of the anatomic features of the tumor and the individual functional organization of adjacent brain tissue. The main predictor of an incomplete tumor resection was the proximity of the lesion to eloquent white matter tracts, such as the corticospinal tract or optic radiations.36 In the absence of fiber tract visualization, the surgeon tends to perform a more conservative resection to avoid postoperative neurologic deficits. The effect of glial tumors on the architecture of hemispheric white matter is still largely unknown. Clearly, to achieve the goal of maximal resection while preserving neurologic function, knowledge of white matter tract location and its relationship to the glioma is as important as defining the tumor’s relationship with the eloquent cortex. Information from multiple brain mapping modalities may provide optimal understanding of the complex structural and functional anatomy of glial tumors in eloquent brain areas, including fMRI and DTI and other preoperatively acquired brain mapping studies such as magnetoencephalography (MEG) or transcranial magnetic stimulation (TMS).37 To be maximally useful, information derived from all imaging modalities must be accurately coregistered with the patient’s brain during the operation and, importantly, updated to account for shifts in the brain shape as surgery proceeds. Whenever possible, most neurosurgeons recommend a near total or gross total resection of all enhancing tumor volume and regionally infiltrated brain as defined on MRI (T2, fluid attenuation inversion recovery [FLAIR]). However, given the infiltrative nature of most gliomas, a gross total tumor resection is, in most cases, not possible, even though it has been shown to increase survival and time to progression.34 To achieve the safest and most accurate resection of glial neoplasms, iMRI has become the method of choice because it allows the surgeon to more carefully delineate tumor margins as he or she performs a more aggressive and thorough tumor resection and to preserve surrounding regions of functioning normal brain. The development of techniques capable of accurately depicting tumor margins in vivo is important to determine the most appropriate surgical treatment for gliomas. To date, we at the Brigham and Women’s Hospital have performed more than 1,000 craniotomies in the magnetic resonance therapy (MRT) suite.5,6,26,38 In doing so, we assessed the main variables that affect the complete MRI-guided resection of low-grade gliomas.19 The statistical analyses identified tumor characteristics to be predictive of incomplete tumor resection: diffuse margin on T2-weighted MRI, histopathologic type, tumor volume, and involvement of the corticospinal tract. More radical resections did not increase the risk of postoperative neurologic deficits.28 An association between MRI-guidance and survival was also found.34,39 Statistical findings such as these underscore the importance of multiparametric imaging for surgical planning and guidance. Also, they evidence that a more complete, iMRI-guided resection can be obtained with high-field MRI and functional navigation. Then, if molecular imaging agents and/or methods are developed for the detection of tumor boundaries, even further improvements in glioma surgery can be expected. Such a step-up requires multimodality imaging in which MRI definition of tumor margins is complemented with optical or nuclear positron emission tomography (PET) or computed tomography (CT) imaging, molecular imaging, and more refined functional and anatomic mapping using fMRI and DTI. By integrating multimodality and multiparametric imaging, an even more complete resection without neurologic impairment can result. And although whether it is an independent predictor remains uncertain, a more complete resection offers a modest survival benefit even in high-grade glioma.34,40 In addition, glioma excision under MRI guidance offers other clinical benefits including limiting surgical morbidity and substantially debulking the glioma to improve the effectiveness of adjuvant therapies such as chemo- and radiation therapy. Should a trial be ethically possible, which it is not, a randomized controlled trial would be the ideal way to resolve the issue of whether (and to what extent) surgical resection leads to improved patient outcomes and survival. Well-controlled retrospective studies may also provide valuable data for a multivariate analysis of all potential confounding factors. Experience using iMRI for benign brain tumors such as meningioma, acoustic neuroma, and pituitary tumors is limited. Conventional surgery with the help of navigational guidance in the operating room is usually successful in removing the tumor completely without complications. Nevertheless, situations arise when intraoperative imaging can help the surgeon to identify residual tumor or to provide essential intraoperative volumetric data, especially if the surgery is performed through a small craniotomy, a transsphenoidal approach, or through neuroendoscopy. In these situations, and when significant brain-shift is present, intraoperative imaging-based guidance can be extremely helpful. An early success in iMRI was the image guidance for the transsphenoidal removal of pituitary tumors.28 In addition to the suprasellar compartment, intra- and parasellar structures were also visualized intraoperatively in great detail. Such intraoperative imaging acts as an immediate “second look,” allowing not only an increase in the resection, but also an increase in the percentage of complete removals. Neurosurgeons are frequently surprised by the extent of residual tumor after an initial resection attempt and find the intraoperative images useful for guiding further resection.28 However, even high-field iMRI could not detect tumor remnants in every case. To identify localized tumor more precisely, dynamic contrasted-enhanced MRI can be used to distinguish residual tumor from normal gland and postoperative changes.41 Gadolinium-soaked cotton pledgets have also been used to identify residual tumor at low-field strength.42 To further improve intraoperative visualization of intra-and parasellar anatomy, provide the best surgical guidance, and facilitate a complete resection, iMRI and endoscopic transsphenoidal surgery can be combined.43 Each method provides complementary information to safely maximize the extent of resection. The evolution of the endoscopic endonasal transsphenoidal technique, which was exclusively used for sellar lesions through the sphenoid sinus, has opened a new access to large areas of the skull base from the nose. This path allows midline access and visibility without brain retraction to the suprasellar, retrosellar, and parasellar space as well as to the areas in the anterior and posterior cranial fossae to make possible the transsphenoidal treatment of a variety of skull base lesions that traditionally have been approached transcranially. Defining the future limits of these extended approaches that most likely require intraoperative image guidance using MRI is difficult. What is easier to predict? Real-time 3D information made available from iMRI may alter the way neurosurgeons approach skull base tumors.44 Intraoperative diffusion and/or perfusion MRI can also diagnose acutely developed vascular occlusion during surgeries 202 and/or endovascular procedures.45 In particular, MR venography (MRV), MR angiography (MRA), and diffusion-weighted imaging can identify vascular structures and functions that may prevent injury during surgery. iMRI may also be used for microsurgical treatment of various vascular lesions. In particular, image guidance has been beneficial for the location of small vascular lesions like cavernomas and for control to ensure a complete resection.46 A navigated approach to access deep-seated lesions is less accurate due to intraoperative brain-shift and brain retraction. During surgery, MRI can identify a patient’s vascular anatomy, including the feeding arteries and draining veins of an intracranial arteriovenous malformation (AVM). However, MR-based imaging’s usefulness as intraoperative angiography remains unknown. Any intraoperative assessment of technical results prior to wound closure does offer the neurosurgeon the opportunity to resect or obliterate a vascular malformation completely to obviate a second operation. Real-time perfusion and diffusion imaging monitors the condition of the brain. Such anatomic and functional monitoring of vascular surgeries and endovascular interventions could significantly improve the safety of planned embolizations or occlusions and clip placements, particularly those in the vicinity of critical perforating vessels. During embolization, diffusion and perfusion intraprocedural imaging is necessary to recognize changes in anatomy (feeding and draining vessel flow and perfusion) and to detect impending infarction. Today, perfusion measurements can be obtained in less than 1 minute of scan time, making this approach a clinically feasible potential adjunct to catheter angiography.47 So far, only a small number of spinal surgical procedures have been performed with iMRI including lumbar discectomies, anterior cervical discectomies with/without fusion, cervical vertebrectomies with fusion, two cervical foraminotomies, and cervical laminectomy. iMRI provides rapid and accurate localization and assessment of the adequacy of decompression. Future applications of iMRI to spine surgery may include intraoperative guidance for resection of the spine and spinal cord tumors and trajectory planning for spinal endoscopy or screw fixation. Certain approaches, such as the transoral route to the cervicomedullary junction, are compromised by a tight surgical corridor and narrow angle of view, making the satisfactory decompression/resection difficult to determine. iMR images can help to determine the adequacy of surgical decompression. The acquisition of iMR images does not adversely affect operative time or neurosurgical techniques, including instrumentation procedures.48 MRI temperature-sensitive imaging can also be used in laser discectomy and vertebroplasty to prevent thermal damage to nerve roots and the spinal cord and to make the procedure safer and better controlled.49 Because of the introduction of smaller endoscopes, neuroendoscopy has become an expanding field of neurosurgery. Neuroendoscopy is part of the trend in modern neurosurgery toward minimally invasive surgical procedures. These procedures can be accomplished by access and visualization through the narrowest entry and through maximum effective action at the target point with minimal disruption to normal tissue. The endoscopic removal of solid brain tumors from the intraventricular compartment would impose additional technical demands by requiring specialized instrumentation and image guidance preferably with iMRI. The first MRI-guided endoscopic application was endoscopic sinus surgery.9,10 The integration of endoscopy with optical tracking and intraoperative interactive imaging allows localization of anatomic landmarks during the procedure. Since that beginning, the endoscope has been used for all types of neurosurgery, including transventricular neuroendoscopy, a procedure aimed at several intracerebral pathologies inside the ventricular system, for which the endoscope is used in real-time iMRI guidance and/or preoperative image-based navigation. Neuroendoscopy has also been expanding beyond just ventriculostomy procedures to those that treat intraventricular tumors, skull base tumors, degenerative spine disease, intracranial cysts, and hydrocephalus. Especially if image guidance is available, the use of the endoscope in neurosurgery has enormous potential. Whereas neuroendoscopic images provide a direct high-resolution view of cavities and spaces in the intracranial compartment, they can be insufficient, without additional navigation capability, for the safe targeting of a lesion. More specifically, they cannot directly locate anatomic structures or pathologies that lie hidden beyond this surface and may only show the internal surface of a cavity. During endoscopic third ventriculostomy (ETV), neuroendoscopic imaging may not reveal the basilar artery that must be avoided to prevent life-threatening vascular damage. A navigation-enhanced neuroendoscope system can be developed that works in the MRI environment.50 Such enhanced neuroendoscopy will be both faster and safer than conventional neuroendoscopy with an even greater ability to minimize possible complications. The developed method will significantly improve the surgeon’s ability to navigate in the ventricles and access remote lesions with less tissue trauma. This new approach favors the use of a flexible endoscope as the use of a rigid endoscope is far from ideal. The rigid endoscope remains the standard due to the difficulties of orienting the flexible endoscope in the ventricle cavity, even though the flexible endoscope is superior to the rigid endoscope in accessibility and flexibility. The navigation-enhanced flexible endoscope should remove much of this difficulty as it will assist physicians in better comprehending the anatomic structures on an image. Once successfully developed, the MRI-guided endoscopic method will be applicable to the resection of intracerebral deep-seated tumors. For example, thalamic gliomas, that are difficult to access through open surgery or standard minimally invasive surgery, may be biopsied or even resected using MRI-guided endoscopes. To introduce MRI-guided endoscopy, a strong need exists to develop MRI-compatible flexible endoscopes equipped with trackable coils. Tracking sensors can detect the position of the tip of the flexible endoscope in the cerebral cavity and show it on 3D MRI models. Then, even in cases of severe anatomic anomalies, flexible neuroendoscopy is safe and accurate. When the images from the MRI are overlaid on the real endoscopic view, previously invisible structures critical in flexible neuroendoscopy can be made visible. MRI-guided neuroendoscopy is particularly useful in the management of complex cystic lesions of the brain when the normal anatomic landmarks on which conventional neuroendoscopy are based have been distorted by underlying pathology. Considering the separate benefits of the MRI depiction of anatomy, neuronavigation, and neuroendoscopy, MRI-guided neuroendoscopic surgery will play an increasingly important role in the future. In the closed-bore magnet, access to the patient is restricted. As a consequence, the main intervention takes place outside the magnet, and the surgical procedure must be interrupted by imaging to update changes related to intraoperative shifts and deformations.44 Mechanical factors, physiologic motions, and pathophysiologic processes such as edema or hemorrhage typically cause these displacements.44
Promising Advances in Intraoperative MRI-Guided Neurosurgery
Intraoperative MRI-Guidance for Brain Tumor Surgery
Intraoperative MRI Guidance for Primary Brain Tumors
Intraoperative MRI Guidance for Benign Brain Tumors
Intraoperative MRI Guidance for Vascular Neurosurgery
Intraoperative MRI Guidance for Spine Surgery
Technical Challenges
MRI-Guided Neuroendoscopy
Serial Imaging and Brain-Shift
Stay updated, free articles. Join our Telegram channel

Full access? Get Clinical Tree
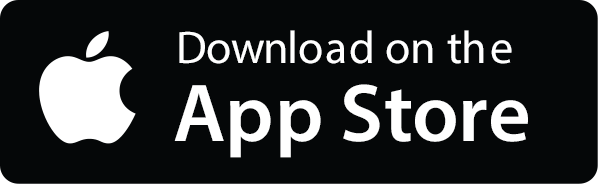
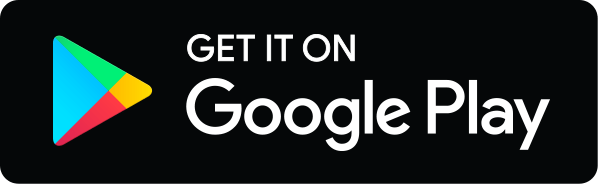