7 In the 50-plus years since it was first introduced, stereotactic radiosurgery, high-dose irradiation of cranial neoplasms delivered in a single fraction, has become a standard of care in the treatment of brain tumors, vascular malformations, functional disorders, and pain. Modern radiosurgery can be performed noninvasively yet with an extremely high degree of accuracy and on an outpatient basis. Within the past 10 years, the success of radiosurgery as a clinical treatment has given rise to optimism that similar gains may be achievable in extracranial sites. In particular, several parallels exist in the nature and management of neoplasms involving the spine. Histologies commonly treated with stereotactic radiosurgery, including schwannomas, meningiomas, arteriovenous malformations, and metastatic disease, also occur frequently in the spine. Management of both cranial and spinal disease is a standard part of the neurosurgical training curriculum. Radiosurgery for spinal disease poses several challenges that are unique. Since its inception, the clinical practice of clinical radiosurgery has relied on rigid skull fixation for localization of intracranial structures. Targets outside the skull, however, are not readily amenable to fixation using rigid frames. Thus, issues of patient positioning, immobilization, reproducibility, and potentially even intrafraction motion present significant challenges in accurate localization and subsequent dose delivery. These practical challenges are exacerbated by the close proximity of the spinal cord, a particularly radiation-sensitive structure in which radiation-induced myelopathy has profound consequences. As late-responding tissues, spinal myelopathies may not manifest themselves for months after treatment. Additionally, although there are 50 years’ worth of clinical data covering the cranial radiosurgery experience, the total number of spinal cases treated worldwide is less than 5000. All of these factors highlight the importance of targeting and dosimetric accuracy and place a high burden on the quality assurance (QA) process. A methodology for clinical radiosurgery for targets involving and adjacent to the spine was first described by Hamilton and Lulu.1 The system consisted of a shallow rigid box, with lateral dimensions compatible with computed tomography (CT) imaging. Patients were placed within the box in a prone position, and under anesthesia, small clamps were attached to one or two spinous processes adjacent to the intended target. These clamps were rigidly attached to two semicircular metal arches secured to the box. The stereotactic space was defined relative to a small radio-opaque sphere using the coordinate system of the CT scanner. Treatment room lasers were subsequently used to align the sphere with the isocenter of the linear accelerator (linac). Imaging, planning, and treatment were performed in a single setting with rigid fixation for the duration of the procedure. The authors used radio-opaque spheres hidden within phantoms and animal cadavers to assess localization uncertainties, reported to be 2.0 mm in a worst case scenario. The “hidden target” test, commonly referred to as a Winston-Lutz test after the authors who first described the procedure in cranial radiosurgery applications, remains the gold standard for assessing target localization.2 This prototype spinal system was subsequently used in the treatment of nine patients, all of whom had recurrent spinal disease.3,4 Doses delivered were understandably conservative, ranging from 8 to 10 Gy, with distributions constructed in such a way that no portion of the spinal cords received > 3 Gy. An attempt to commercially market the “Arizona” spinal radiosurgery system proved unsuccessful. Several specialized systems for performing spinal radiosurgery have been described since the initial Arizona experience. Ryken et al modified an infrared (IR)-based navigation system to facilitate ultrasound-guided targeting of paraspinal tumors.5 System applicability was limited to soft tissue tumors located on the dorsal aspect of the spinal column; disease involving the bony vertebrae, the most common site for metastatic spread, could not be localized due to inherent limitations of ultrasound imaging. The authors subsequently described the treatment of a single patient presenting with a recurrent metastatic squamous cell carcinoma at the level of T11; a dose of 15 Gy was delivered to the 80% isodose line. To date, this is the only publication on this particular technique. Medin et al proposed a minimally invasive localization technology that allowed for high-dose, single-fraction irradiation of soft tissue or bony spinal tumors.6 Briefly, under local anesthesia, three small radio-opaque markers were permanently affixed within the vertebral and spinous processes. Patients received a subsequent CT scan in which the target was identified and contoured. Simultaneously, biplanar radiographs were obtained in which the implanted markers were identified. Both imaging procedures utilized an external localization box from which a coordinate system was established. Within this coordinate system, the locations of the implanted fiducials were determined from the biplanar radiographs, and the location of the target isocenter was determined from the CT scan. Prior to treatment, biplanar radiographs were repeated with the patient in the treatment position. The implanted fiducials were identified, and the isocenter position was calculated based on the geometric relationship between the target and implanted markers obtained at the time of CT imaging. In this manner, accurate target localization could be performed despite the fact that (1) the patient had moved from the time of the initial CT and (2) the target could not be directly visualized in the treatment room. The authors went on to experimentally evaluate the overall system accuracy. Phantoms were constructed to evaluate the integrity of absolute spatial dimensions and to study the effect of marker and target spacing. The largest targeting error observed was 1.17 mm, with most combinations tested resulting in targeting errors well below 1 mm. The methodology was subsequently evaluated in a swine model. Results indicated that (1) the implant procedure was simple to perform, requiring < 30 minutes time to complete; (2) implanted markers were readily distinguishable from normal anatomy on radiographs; (3) once implanted, markers stayed fixed and did not move relative to the intended target or to one another; (4) with the swine in a supine position, markers did not move with normal or forced respiration; and (5) there was little loss in targeting accuracy when markers associated with one vertebra were used to target adjacent vertebrae. This system represented a true milestone in accurate, minimally invasive stereotaxis of a wide range of neoplasms associated with the spine. Two additional single-institution systems for spine radiosurgery have been described in the literature. Yenice et al constructed a stereotactic body frame to facilitate immobilization and localization.7 The frame incorporates CT localization fiducial plates that define a coordinate system to localize patient anatomy with respect to the frame. Unique to the design, the patient is initially set up in a vertical standing position, after which the frame and patient are tilted backward into a horizontal treatment position. It is believed that this manner of setup provides for better daily reproducibility of internal anatomy. The system is also designed to facilitate daily CT imaging, which is performed just prior to each treatment. The authors were able to demonstrate a localization accuracy of within 1 mm in any direction, which they concluded was sufficient to ensure that the maximum dose to the spinal cord was within 10 to 15% of the planned value. Daily CT imaging was eventually replaced with localization based on electronic portal imaging, with little loss of targeting accuracy.8 A similar system for spine radiosurgery was described by Shiu et al.6,9 Patients were immobilized in a full-body stereotactic frame and received localization/verification CT scans immediately prior to treatment. This was facilitated by a CT on rails installed in the treatment room. With daily CT imaging, the authors evaluated the overall deviation from the intended isocenter was within 1 mm for each treatment. Furthermore, they determined the effect of respiration on spinal targeting accuracy and concluded that, for supine patients, there was no significant movement. Since these initial attempts, two commercial systems have been developed and widely adopted for spine radiosurgery. The first, the CyberKnife system (Accuray Inc., Sunnyvale, California), is a robotic radiosurgery system originally designed to treat cranial tumors without a stereotactic head frame.10–12 The CyberKnife consists of a 6 MV X-band linear accelerator attached to a robotic arm that can move about the patient with six degrees of freedom, coupled with two diagnostic x-ray units projecting on two opposing amorphous silicon detectors. The biplanar imaging system provides the capabilities for frameless stereotactic radiosurgery.13 Because the imaging system is permanently mounted in the treatment room, targeting can be performed without the need for additional “localization boxes.” The integrated image guidance system employed by the CyberKnife provides the capabilities for stereotactic irradiation of extracranial sites.14 Murphy et al described modifications to the CyberKnife to facilitate stereotactic irradiation of spinal tumors.15 For the cervical spine, image registration based on bony anatomy is performed between in-room images and digitally reconstructed radiographs (DRRs) obtained from the planning CT. In the thoracic and lumbar spine, however, much of the bony anatomy is superimposed upon itself in the oblique projections, making image-based registration difficult. For these anatomical sites, the authors implant fiducial markers in the bony spine adjacent to the target of interest. The fiducial markers are readily localized on x-ray images from which the target position can subsequently be determined. In contrast to the methodology described by Medin et al,6 markers were inserted in the vertebral bodies (as opposed to the spinous and vertebral processes), and the reference marker locations were derived from CT images (as opposed to radiographs). Yin et al have described a methodology for stereotactic spinal irradiation using the second commercial system, the Novalis system (BrainLab AG, Feldkirchen, Germany).16 The Novalis system incorporates an IR component for patient monitoring and a stereoscopic x-ray component for localization of extracranial targets. The IR component includes two ceiling-mounted cameras that detect the three-dimensional (3D) positions of IR reflective markers placed on the surface of the patient. The IR signal is continuously updated every 50 msec. Although studies by Wang et al have demonstrated that the position of each IR-reflecting sphere can be determined to be < 0.3 mm, with overall CT-defined targeting accuracy in rigid objects on the order of 3 mm at the 95% confidence level, issues of marker reproducibility and patient motion preclude the use of the IR system for accurate stereotaxis.17 The kV x-ray component consists of two floor-mounted x-ray tubes and two opposing amorphous-silicon (a-Si) flat-panel detectors mounted to the ceiling. Each x-ray tube/detector pair is configured to image through the linac isocenter with a coronal field of view of about 18 cm in both the superoinferior and left-right directions at the isocenter.
Quality Assurance in Spine Radiosurgery
Description of Spine Radiosurgery Systems
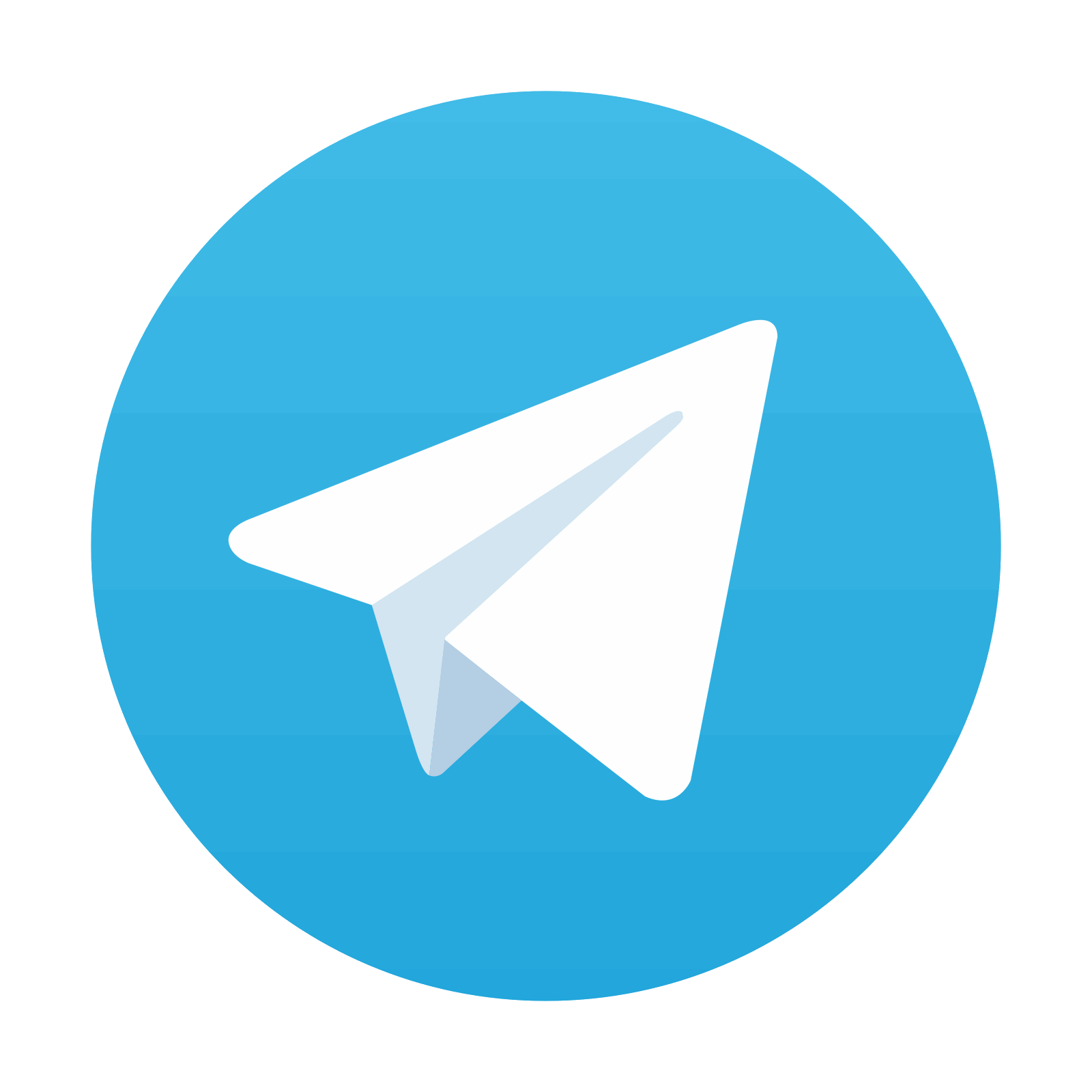
Stay updated, free articles. Join our Telegram channel

Full access? Get Clinical Tree
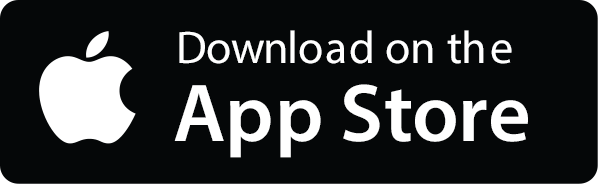
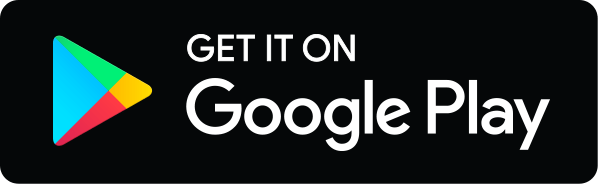