Quality of X-Ray Beams
In Chapter 5, we described an x-ray beam in terms of photon fluence and energy fluence. Such a description requires the knowledge of the number and energy of the photons in the beam. In this chapter, we will characterize an x-ray beam in terms of its ability to penetrate materials of known composition. The penetrating ability of the radiation is often described as the quality of the radiation.
An ideal way to describe the quality of an x-ray beam is to specify its spectral distribution, that is, energy fluence in each energy interval as shown in Figure 3.9. However, spectral distributions are difficult to measure and, furthermore, such a complete specification of the beam quality is not necessary in most clinical situations. Since the biologic effects of x-rays are not very sensitive to the quality of the beam, in radiotherapy one is interested primarily in the penetration of the beam into the patient rather than its detailed energy spectrum. Thus, a crude but simpler specification of the beam quality is often used, namely the half-value layer.
7.1. HALF-VALUE LAYER
As defined earlier (Chapter 5), the term half-value layer (HVL) is the thickness of an absorber of specified composition required to attenuate the intensity of the beam to half its original value. Although all photon beams can be described in terms of their HVL, the quality of a γ-ray beam is usually stated in terms of the energy of the γ rays or its nuclide of origin, which has a known emission spectrum. For example, the quality of a γ-ray beam emitted from a 60Co source can be stated in terms of 1.17 and 1.33 MeV (average 1.25 MeV) or simply cobalt-60 beam. Because all x-ray beams produced by radiation generators are heterogeneous in energy (i.e., possess continuous energy spectra that depend on the peak voltage, target material, and beam filtration), they are usually described by the HVL, a single parameter specifying the overall penetrating ability of the beam.
In the case of low-energy x-ray beams (below megavoltage range), it is customary to describe the quality in terms of HVL together with kVp, although HVL alone is adequate for most clinical applications. On the other hand, in the megavoltage x-ray range, the quality is specified by the peak energy and rarely by the HVL. The reason for this convention is that in the megavoltage range the beam is so heavily filtered through the transmission-type target and the flattening filter that any additional filtration does not significantly alter the beam quality or its HVL. Thus, for a “hard” beam with a fixed filtration, the x-ray energy spectrum is a function primarily of the peak energy and so is the beam quality. The average energy of such a beam is approximately one-third of the peak energy.
7.2. FILTERS
In Section 3.5, we briefly discussed the energy spectrum of an x-ray beam. The x-rays produced by an x-ray generator show a continuous distribution of energies of bremsstrahlung photons on which are superimposed discrete lines of characteristic radiation (Fig. 7.1). The curve A in Figure 7.1 schematically represents the energy spectrum of a 200-kVp x-ray beam filtered by a 1-mm-thick aluminum filter. This distribution includes the effects of attenuation in the glass envelope of the x-ray tube, the surrounding oil, and the exit window of the tube housing as well. This so-called inherent filtration is equivalent to approximately 1-mm Al in most x-ray tubes.
The K-characteristic x-rays produced in the tungsten target possess discrete energies between 58 and 69 keV (Table 3.1). Other emission lines of tungsten, however, have much lower energies
and are not shown in Figure 7.1 because they are effectively removed by the inherent filtration as well as the added filtration.
and are not shown in Figure 7.1 because they are effectively removed by the inherent filtration as well as the added filtration.
![]() Figure 7.1. Schematic graph showing changes in spectral distribution of a 200-kVp x-ray beam with various filters. Curve A is for Al, curve B is for Sn + Al, and curve C is for Sn + Cu + Al. |
The energy fluence of the K lines of tungsten can be preferentially reduced using a tin filter. Because the K absorption edge of tin is at about 29.2 keV (Table 3.2), it strongly absorbs photons above 29.2 keV by the photoelectric process. However, lower-energy photons cannot eject the K electrons. As seen in curve B of Figure 7.1, the energy fluence in the region from 30 to 70 keV is considerably reduced relative to either the higher-energy part of the spectrum or the spectrum below 29 keV. Because the L absorption edge of tin is only 4.5 keV, there is little reduction in the spectrum below 29 keV. In addition to the above effects, tin produces its own characteristic radiation by the photoelectric process involving the K shell, and these lines are superimposed on the spectrum below the tin absorption edge.
To absorb preferentially the energy fluence below the K edge of tin, including the characteristic x-rays of tin, a copper filter is quite efficient. The K edge of copper is at 9 keV, and therefore, the photons below 29 keV are strongly absorbed by the copper filter as seen in curve C of Figure 7.1. The very-low-energy characteristic x-rays produced by copper can be effectively absorbed by adding an aluminum filter next to the copper filter.
Combination filters containing plates of tin, copper, and aluminum have been designed to increase the resulting HVL of the orthovoltage beams without reducing the beam intensity to unacceptably low values. Such filters are called Thoraeus filters (1) and are described in Table 7.1. It is important that the combination filters be arranged in the proper order, with the highest-atomic-number material nearest the x-ray target. Thus, a Thoraeus filter is inserted with tin facing the x-ray tube and the aluminum facing the patient, with the copper sandwiched between the tin and the aluminum plates.
In the diagnostic and superficial x-ray energy range (Section 4.1), primarily aluminum filters are used to harden the beam. The HVLs of these beams are also expressed in terms of millimeters of aluminum. In the orthovoltage range, however, combination filters are often used to obtain HVLs in the range of about 1 to 4 mm Cu. For cesium and cobalt teletherapy machines, on the other hand, filters are not needed because the beams are almost monoenergetic.
Although a megavoltage x-ray beam has a spectrum of energies, the beam is hardened by the inherent filtration of the transmission target as well as by transmission through the flattening filter. Thus, no additional filtration is required to improve the beam quality. The primary purpose of the flattening filter is to make the beam intensity uniform in cross section rather than to improve the beam quality. It may also be mentioned that the flatness of the beam is irrelevant if patients are treated with some form of intensity-modulated radiation therapy (IMRT). Accordingly, some vendors are moving toward flattening-filter free (FFF) machines.
TABLE 7.1 Thoraeus Filters Used with Orthovoltage X-rays | ||||||||
---|---|---|---|---|---|---|---|---|
|
7.3. MEASUREMENT OF BEAM QUALITY PARAMETERS
A. HALF-VALUE LAYER
As discussed in Section 5.3, the HVL of a beam is related to the linear attenuation coefficient (µ) by the following equation:

Like the attenuation coefficient, the HVL must be measured under narrow-beam or “good” geometry conditions. These conditions can be achieved by using a narrow beam and a large distance between the absorber and the detector (Fig. 5.1). Under good geometry conditions, the exposure reading is mainly a result of the photons that are transmitted through the absorber without interaction and practically no scattered photons are detected by the chamber. The attenuation data are obtained by measuring transmitted exposure through absorbers of varying thickness but constant composition. These data are then plotted on a semilogarithmic graph to determine HVL. If the beam has a low filtration or contains an appreciable amount of low-energy component in the spectrum, the slope of the attenuation curve decreases with increasing absorber thickness (Fig. 5.3). Thus, different HVL beams can be obtained from such a beam by using different filters. In general, the HVL increases with increasing filter thickness as the beam becomes increasingly “harder,” that is, contains a greater proportion of higher-energy photons. Beyond a certain thickness, however, additional filtration may result in “softening” of the beam by Compton scattering.
Because an increase in filtration is accompanied by a reduction in the available exposure rate, the filtration is carefully chosen to obtain a suitable HVL as well as acceptable beam output. In addition, as discussed in the previous section, certain filters are more efficient than others in selectively removing low-energy photons from the beam, including characteristic x-rays that are undesirable for therapy because of their low energy.
B. PEAK VOLTAGE
Neither the HVL nor the tube potential nor both provide sufficient information regarding the spectral distribution of the radiation. However, for most clinical purposes, these two parameters give an appropriate specification of radiation quality. It has been recommended (2
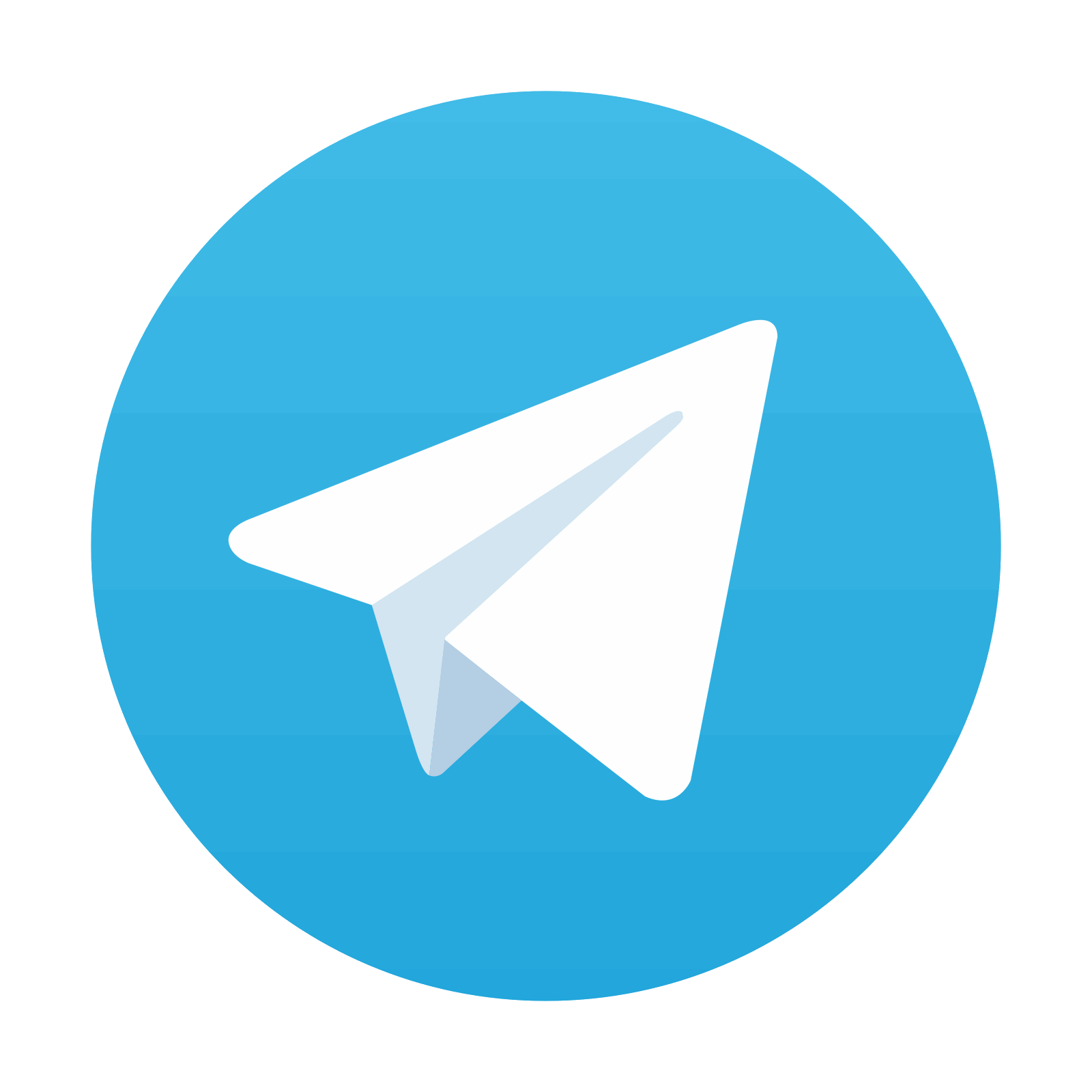
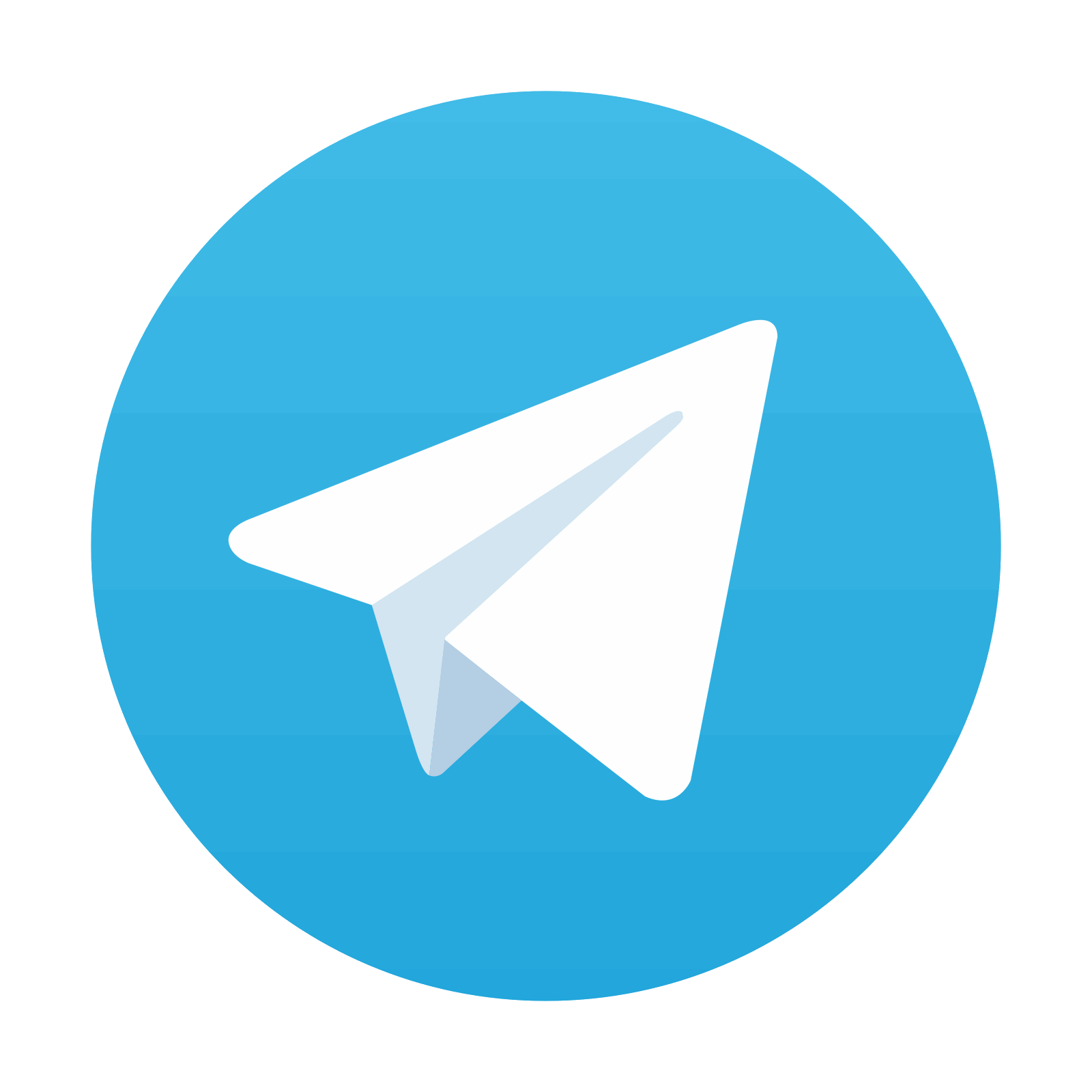
Stay updated, free articles. Join our Telegram channel

Full access? Get Clinical Tree
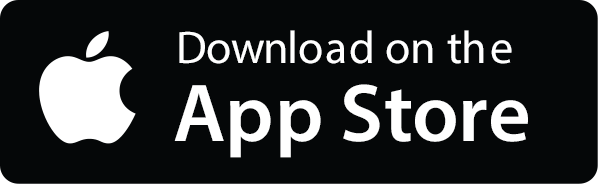
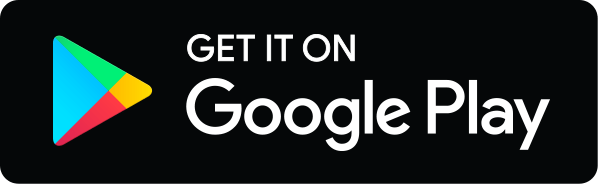
