Introduction and Background
Although different in appearances on imaging, radiation and drug-induced lung diseases share several similarities. Both entities may present with a variety of appearances, both in the acute and subacute or chronic settings, and require a high level of suspicion to make the correct diagnosis. Most importantly, these processes share a temporal relationship with the causal event. Furthermore, the two processes may coexist in oncologic patients being treated with radiation and chemotherapy. The imager should always consider both radiation or drug reaction as a cause of otherwise unexplained imaging findings.
Radiation-Related Lung Disease
Radiation therapy is a common treatment for many thoracic malignancies and is, therefore, encountered frequently by the thoracic imager, especially in busy oncologic practices. Many of the side effects of radiation are permanent and, because radiation is often administered with intent to cure, imaging abnormalities may be encountered years or decades after treatment. It is important for the imager to understand the basic treatment strategies used and recognize different patterns of radiation pneumonitis and fibrosis so that these entities can be confidently differentiated from infection, tumor recurrence, or another primary tumor.
Purpose of Radiation Treatment
Radiation therapy is an effective treatment option for many intrathoracic malignancies but is most commonly used in the treatment of lung cancer. The purpose of radiation, and the type of radiation used, depends largely on the stage of disease. For example, in stages I and II lung cancers, radiation is potentially curative and may be the only treatment option in patients who are not surgical candidates (e.g., those with severe lung or heart disease). Locally advanced lung cancers with unresectable primary tumors or nodal involvement (stage IIIA) may undergo radiotherapy with the hope of cure or as an adjunct therapy. Patients with metastatic disease may receive radiation as palliation to relieve pain and improve quality of life.
Radiation therapy aims to kill tumor cells by delivering as high a dose as possible to the cancer cell while limiting damage to adjacent normal tissue. Historically, conventional radiation therapy has used parallel radiation beams with opposite orientation (anteroposterior and posteroanterior) to deliver treatment doses of approximately 60 gray (Gy) in daily doses of about 2 Gy each. This technique was limited by the large amount of normal tissue exposed within the radiation fields, including the mediastinum and spinal cord. Oblique beams can be used to boost the dose and reduce exposure to these important structures.
Conventional radiation therapy has largely been replaced by several modern techniques, most notably three-dimensional conformal radiation therapy (3D-CRT) and stereotactic beam radiation therapy (SBRT), that deliver large doses of radiation to a tumor while minimizing adjacent tissue injury. 3D-CRT uses CT images to create a 3D tumor volume, and complex computer algorithms generate multiple oblique radiation fields that deliver the dose to the tumor from many different angles. This technique allows even higher doses of radiation (≥70 Gy) to be delivered to a tumor while limiting the dose to adjacent normal tissues. SBRT is a conformal technique for the treatment of nonoperable stage I lung cancers, with local control rates reported of 80% to 100%. A stereotactic body frame is used to localize the tumor and focus high doses of radiation on a small field.
Many other malignancies are also treated with radiation, and the effects are frequently encountered on thoracic imaging. These include hematologic malignancies such as Hodgkin lymphoma or leukemia, breast cancer, esophageal cancer, and head or neck cancer. The patterns of radiation-related findings in each of these diseases will be discussed later in this chapter.
Pathophysiology and Timeline of Radiation-Related Lung Disease
The lung parenchymal response to radiation is fairly limited and may manifest as one of two patterns—radiation pneumonitis or radiation fibrosis—and there are many factors that contribute to the severity of injury suffered by a particular patient. Technical factors include the volume of lung irradiated, total dose, dose fractionation, and time frame over which the radiation is delivered. Lung injury rarely occurs when total doses are below 20 Gy, but some degree of injury is usually present if more than 40 Gy is administered. Concurrent chemotherapy or withdrawal of steroids may also worsen the lung injury. Individual susceptibility, preexisting lung disease, or prior radiation may also play a role.
The last day of radiation treatment is usually used as a reference point to assess the timeline of radiation pneumonitis and radiation fibrosis. Radiation injury to the lung results in diffuse alveolar damage that evolves through three phases: (1) acute exudative phase, (2) organization and proliferation phase, and (3) chronic fibrotic phase.
Radiation Pneumonitis
The acute exudative phase and organization and proliferation phase correspond to the time frame of radiation pneumonitis and occur within the first 1 to 6 months after completion of therapy. Clinical symptoms include dyspnea, cough, low-grade fever, and/or chest discomfort. The incidence of symptomatic radiation pneumonitis for 3D-CRT is approximately 13% to 37% and much less (4%) for SBRT. Symptoms will usually resolve spontaneously but, in some cases, may require treatment with steroids.
Characteristic imaging findings of radiation pneumonitis on CT include ground-glass opacities (GGOs) and/or consolidation that are almost always confined to the radiation field ( Fig. 25.1 ). The radiation fields are nonanatomic, so the parenchymal abnormalities often cross fissures and are not confined to a single lobe or segment. In the organization and proliferation phase, imaging findings may evolve as volume loss and bronchiectasis start to develop. Sterile pleural effusions may be present, and pleural thickening in the radiation field may also be observed. These findings may also be visible on chest radiographs, although subtle GGOs may be below the limits of detection.
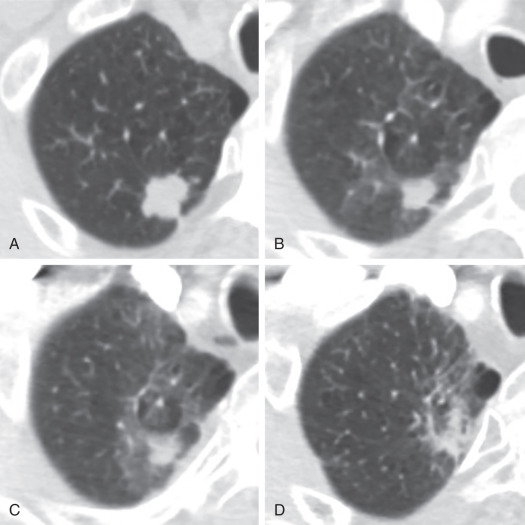
It is very important to realize that parenchymal abnormalities may develop outside of the radiation field during the radiation pneumonitis time frame; these usually represent an organizing pneumonia (OP), which may be a direct effect of radiation or an immune reaction to radiation injury. This has been reported in up to 20% of patients who have undergone radiation therapy and should not be mistaken for tumor recurrence. Imaging findings of OP outside of the radiation field include GGOs or consolidation that is often migratory and may relapse if steroid therapy ceases.
Radiation Fibrosis
Radiation fibrosis represents the chronic fibrotic phase of lung injury and usually develops 6 to 12 months after the completion of radiation therapy. Symptoms are less frequent than with radiation pneumonitis but, when present, include dyspnea and persistent dry cough. Radiation fibrosis may develop with or without clinically appreciable antecedent pneumonitis.
Histologically, collagen deposition and fibrosis are present in the lung parenchyma. Similar to radiation pneumonitis, the imaging findings may be visible on both radiographs and CT scans, although CT is more sensitive. The main imaging findings include architectural distortion, consolidation with air bronchograms, and traction bronchiectasis with sharp borders that cross fissures and other anatomic landmarks, depending on the radiation field. The fibrosis results in volume loss and corresponding ipsilateral mediastinal shift ( Fig. 25.2 ). Radiation fibrosis should stabilize by 24 months after the completion of therapy. Changes outside this time frame (e.g., especially increased soft tissue, filling in of previously patent bronchi, new nodular borders) should raise suspicion of tumor recurrence, a new tumor, or another process such as infection.
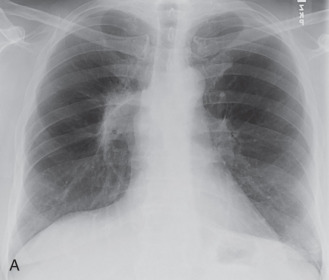
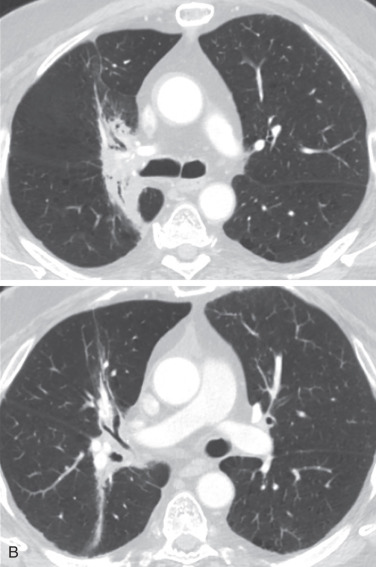
Patterns of Radiation Treatment Seen in the Chest
It is important for the thoracic imager to recognize the different distributions of radiation pneumonitis and radiation fibrosis that may be encountered. Knowing the type of primary tumor (e.g., lung cancer, esophageal cancer, lymphoma) and time since completion of therapy can improve diagnostic confidence and prevent misinterpretation. Radiation pneumonitis occurs within the first 6 months, whereas radiation fibrosis may develop up to 2 years after completion of therapy (see Fig. 25.1 ).
Knowing the type of radiation that was administered can help predict where radiation-induced abnormalities occur. Usually, these findings will be seen in the lungs, but any tissues in the radiation field (e.g., skin, soft tissue, bone, vessel, heart) may suffer injury. Conversely, when a pertinent history is not available to the imager, the distribution of abnormalities may allow one to surmise the treatment that was administered. Table 25.1 lists the types of radiation and expected distribution of findings. Examples of different treatment patterns are shown in Fig. 25.3 .
EXPECTED DISTRIBUTION OF ABNORMALITIES | TYPES OF RADIATION |
---|---|
Anteroposterior or oblique; extends from one pleural surface to another, may cross fissures | Conventional radiation therapy (usually for lung cancer); 3D-CRT may have a similar distribution but not as severe |
Focal, isolated to one region of lung parenchyma | SBRT (possibly 3D-CRT) used to treat a localized lung cancer |
Paramediastinal, bilateral | Mantle radiation for Hodgkin disease (or some other types of lymphoma); radiation for thymic tumors |
Paramediastinal, unilateral | Radiation for lung cancer nodal disease or esophageal cancer |
Unilateral, tangential to chest wall | Radiation for breast cancer |
Apical, unilateral | Axillary nodal metastases (usually breast cancer) |
Apical, bilateral | Head and neck cancer |
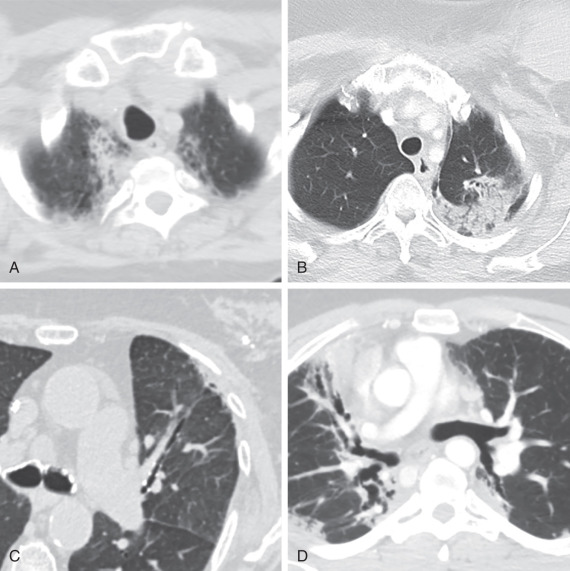
If lung parenchymal abnormalities are present, the imager should interrogate the entire radiation field to look for complications, particularly in the setting of lung fibrosis from remote radiation. These findings are discussed in further detail later (see “ Long-Term Effects of Radiation ”).
Differentiating Radiation Pneumonitis and Fibrosis From Other Processes
The primary differential diagnosis for radiation pneumonitis or fibrosis includes tumor recurrence, infection, and a radiation-induced secondary neoplasm. In many cases, the diagnosis may be obvious based on imaging or the clinical presentation alone, but in many cases a multimodality and multidisciplinary approach may be necessary to reach a confident diagnosis. Comparison to prior imaging examinations and knowledge of the treatment regimen are essential.
Tumor Recurrence
Recurrence usually occurs within the first 2 years after completion of therapy, but it can occur after this window. Modern 3D-CRT and SBRT offer lower recurrence rates than conventional treatments because of the higher doses that can be delivered to tumors. Tumors may relapse outside the radiation field and present at imaging as new nodules in the lung, new lymphadenopathy, or lymphangitic carcinomatosis. Tumor recurrence within the radiation field or along the margin of radiation may be more difficult to detect. Clues of recurrence on CT are often subtle and include the following: new soft tissue nodularity or convexity along a radiation field margin; new mass within the radiation field; and secondary signs of soft tissue infiltration, including filling in of previously demonstrated air bronchograms or a new mass effect or compression on vessels and airways ( Fig. 25.4 ). A new pleural effusion long after completion of therapy (months to years) should also raise suspicion of recurrence.
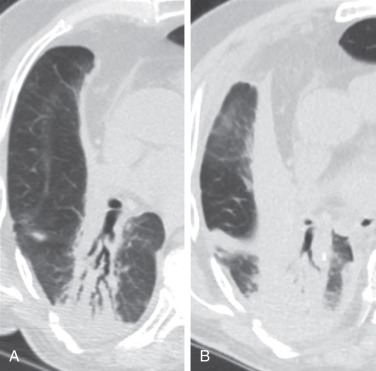
Because recurrence may occur during the first 2 years after completion of therapy, while radiation pneumonitis and fibrosis are evolving, PET-CT may be useful for differentiating the two entities. Although radiation pneumonitis represents a metabolically active response to lung injury, the maximum standard uptake value (SUV max ) is usually higher in tumors. However, because of the false-positive potential, PET-CT should generally not be performed in the first 3 months after the completion of radiation therapy. In some cases, tissue biopsy or short-term follow-up imaging is necessary to confirm a diagnosis of recurrence.
Infection
Infection may be particularly difficult to differentiate from radiation pneumonitis because clinical symptoms and imaging findings overlap. Imaging findings that are unusual as a result of radiation pneumonitis, and should prompt evaluation for infection, include tree-in-bud nodules, cavitation, diffuse bilateral abnormalities that extend far beyond the radiation field, or GGOs or consolidation that develop before the completion of radiation therapy. The rapid development of a new abnormality may also be a clue of infection, although steroid withdrawal can incite fairly rapid development of radiation pneumonitis.
Radiation-Induced Tumor
Secondary tumors are a late consequence of radiation therapy (median time, ≈10 years). This should be included on the differential in the appropriate clinical context and is discussed in more detail in the following section.
Long-Term Effects of Radiation
Non-Neoplastic Extrapulmonary Effects
All tissues in the body have varying susceptibilities to radiation-induced damage. In the thorax, this includes the tissues superficial to the lung parenchyma (e.g., subcutaneous tissues, bones, pleura) and mediastinal structures (e.g., vessels, cardiac valves, pericardium, lymph nodes, esophagus, thymus). The most important effects are listed in Table 25.2 , and inspection of these structures should be part of a search pattern when they fall within a radiation field.
