and Lori Ann Lewis1
(1)
Clinical Research Center of North Texas, Denton, TX, USA
Abstract
X-ray densitometers expose patients to extremely small amounts of radiation in comparison to plain X-ray techniques. These amounts are often so small that they are biologically insignificant. Similarly, the technologist operating an X-ray densitometer on a regular basis is extremely unlikely to be exposed to a significant amount of radiation. Nevertheless, no amount of radiation should be considered inconsequential. The principle of “as low as reasonably achievable” (ALARA) should always be given the highest priority in the operation of these devices.
X-ray densitometers expose patients to extremely small amounts of radiation in comparison to plain X-ray techniques. These amounts are often so small that they are biologically insignificant. Similarly, the technologist operating an X-ray densitometer on a regular basis is extremely unlikely to be exposed to a significant amount of radiation. Nevertheless, no amount of radiation should be considered inconsequential. The principle of “as low as reasonably achievable” (ALARA) should always be given the highest priority in the operation of these devices.
Radiation Basics
X-rays are a form of electromagnetic energy. Other forms of electromagnetic energy are radio and television waves, microwaves, radar, infrared, visible and ultraviolet light, and gamma (γ) radiation. These types of energies form the electromagnetic spectrum of energy. When energy is released and then transmitted through a substance, it is called radiation. The substance through which the radiation has passed is said to have been “irradiated” or “exposed” to radiation. Ionizing radiation is radiation that causes the release of an electron from its orbit around an atom when the radiation passes through the substance containing that atom [1]. X-rays and γ-rays are also forms of ionizing radiation.
Radiation Quantities
In X-ray densitometry, the technologist must be concerned with the amount of ionizing radiation to which both the patient and technologist are exposed. A review of the terminology describing these quantities is necessary before discussing the potential effects of ionizing radiation on living tissue and the exposure levels produced during various densitometry exams.
The Curie
The most basic unit of radiation is the Curie (Ci). This is used to quantify the amount of a radioactive material, not the radiation emitted by the material. The SI1 unit equivalent to the Ci is the becquerel (Bq). The formula for converting Ci to Bq is shown in (5.1):
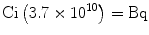
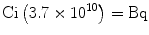
(5.1)
To use this equation, multiply the number of Ci by 3.7 × 1010 to determine the number of Bq. Amounts of radioactive material are often described as being a certain number of millicurie (mCi) or even microcurie (μCi).
The Roentgen
The Roentgen (R), named for Wilhelm Roentgen who discovered X-rays, is used to describe a quantity of radiation exposure, but it is only used to describe the interaction of X-rays and γ-rays with air. The roentgen is based on the electrical charge created by the liberation of electrons that occurs during ionization. In densitometry, the Roentgen is rarely used except to describe measured amounts of scatter radiation in the air when the devices are in use. This quantity is quite low and generally expressed in milliRoentgens (mR). The Roentgen also has an SI counterpart, called the coulomb per kilogram (C/kg). The mathematical conversion of Roentgens to coulombs per kilogram is shown in (5.2).
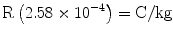
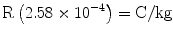
(5.2)
The Rad
Rad is both an abbreviation and acronym for radiation absorbed dose. It is used in conjunction with any kind of ionizing radiation and any type of substance that has been exposed to ionizing radiation. The rad is commonly used to express the quantity of radiation received by a patient. The biologic effects of radiation are often associated with various quantities of radiation given as rads. The SI rad equivalent is the Gray2 (Gy). The mathematical relationship between the rad and Gy is simple; 100 rads equal 1 Gy. This is expressed in (5.3).
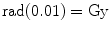
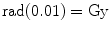
(5.3)
For medical X-rays, 1 R is considered to be approximately equal to 1 rad because the radiation exposure to human tissue from 1R is only about 5 % more than 1 rad.
The Rem
Rem is also both an abbreviation and acronym for rad equivalent man. The rem expresses the quantity of radiation received by a patient, but unlike the rad, the quantity has been adjusted to reflect the type or quality of radiation involved [2]. This recognizes that different types of ionizing radiation have different potentials to do harm. The conversion of rads to rems is expressed in (5.4):


(5.4)
Medical X-rays are assigned a quality factor of 1. As a consequence, multiplying the number of rads by a medical X-ray quality factor of 1 does not change the value. For medical X-rays then, in the context of whole body exposure, a rad is equal to a rem. By extension, for medical X-rays, 1 R is also approximately equal to 1 rem. This is not true for all types of ionizing radiation however. For example, alpha particle radiation as seen with radon exposure has a quality factor of 20. Because radon is a gas, this exposure reflects the dose to the lungs. The exposure from medical X-rays in rads or rems is often called the skin dose.
The Effective Dose Equivalent
The effective dose equivalent (HE) is a concept, rather than a particular unit of measure. The concept was introduced in 1987 in an attempt to relate the magnitude of an exposure in rems or Sv to the risk created by that exposure [3]. In other words, the effective dose equivalent is a measure of risk from an exposure and not a measure of the exposure itself. As noted in the discussion of the rem, the dose in rads must be multiplied by a quality factor for the type of ionizing radiation, recognizing that different types of radiation have different potentials to do harm. Similarly, different tissues or organs within the human body have different sensitivities to radiation. Some tissues are more sensitive than others. It matters then what tissues are being irradiated in determining what the risk of that irradiation truly is. This is the concept behind the effective dose equivalent. Tissue weighting factors (w T) are assigned to the various tissues in the body. The HE is determined by multiplying the value in rems or Sv by the w T. Because the w T has no units of its own, the HE is still expressed in rems or Sv.
The International Commission on Radiological Protection (ICRP) determines the various w T’s. The body as a whole is assigned a w T of 1. Individual tissues or organs have w T’s less than 1 and vary widely. In the most recent recommendations [4] from the ICRP, the ovaries and testes were assigned a value of 0.08, although in prior recommendations, they had been assigned a much higher value of 0.20 [5]. The thyroid and liver are each assigned a w T of 0.04 [4]. The red bone marrow is assigned a w T of 0.12 as are the breasts, colon, and lung. The HE that is calculated for the exposure of any given area of the body is an expression of the risk that would result if the entire body was exposed to the same amount of radiation. For example, the HE for a radiographic absorptiometry study of the phalanges on the Metriscan™ is stated as being less than 0.0001 mrem or 0.012 μSv [6]. This means that the risk of the radiation exposure from such a densitometry study of the phalanges is the same as if the entire body was exposed to less than 0.0001 mrem. This is not a measure of the amount of radiation exposure to the phalanges. The effective dose equivalent is an expression of the biologically important risk associated with any given amount of radiation exposure.
Harmful Effects of Ionizing Radiation
Ionizing radiation has the potential to harm living tissue. In addition to medical X-rays, there are other sources of ionizing radiation. One important source found naturally in the environment is radon. Radon is a gas formed by the decay of uranium, which is normally found in small amounts in the earth. Materials that are derived from the earth, like concrete and brick, will therefore contain small amounts of radon to which everyone is exposed. The largest source of man-made ionizing radiation is medical X-rays. Other man-made sources include nuclear power generators, consumer products like smoke detectors and televisions, and industrial sources. In comparison to natural environmental radiation, man-made sources of ionizing radiation contribute very little to the total annual radiation exposure of an individual. Nevertheless, ionizing radiation does have the potential to do harm. The decision to expose a patient to ionizing radiation, no matter how small in amount, should not be made lightly.
Although ionizing radiation can cause an increase in the expected number of mutations, the mutations that result are not unique. In spite of the frightening and bizarre images seen in movies of giant crickets devouring Chicago after being exposed to ionizing radiation, the types of mutations that are actually seen are those that occur in nature. They simply will occur more frequently. Similarly, cancers that can result from high doses of ionizing radiation are not unique. The incidence of almost all types of cancer is increased after exposure to high doses of ionizing radiation, but these are the same cancers seen in individuals who have not been exposed.
Acute Lethal Radiation Syndromes
Acute lethal radiation syndromes are mentioned here only for the sake of completeness. They cannot occur with the devices used in densitometry because the radiation doses required to produce them are thousands of times greater than those used in densitometry. DXA and SXA X-ray tubes are incapable of producing the high doses of radiation necessary to cause these syndromes because of their relatively low applied voltage and current. The peak kilovoltage (kVp) of the tube determines the amount of radiation that can be delivered by the tube. The X-ray tube current (mA) determines the number of X-rays that are produced which also affects the amount of radiation produced. In densitometry X-ray tubes, the kVp and mA are far too low to cause any of these syndromes. Nevertheless, the technologist should be aware of them, if only to reassure the anxious patient that they cannot occur with X-ray densitometry.
There are three different acute syndromes that ultimately result in death. The syndromes are called hematologic death, gastrointestinal (GI) death, and central nervous system (CNS) death. Doses of 200–1,000 rads can cause nausea, vomiting and diarrhea, hemorrhage, and a decrease in the white blood cell count leading to infection and fever. Hematologic death occurs within 10–60 days. Higher doses of 1,000–5,000 rads result in GI death in 4–10 days that is preceded by lethargy and shock as well as all the signs and symptoms seen with the hematologic death syndrome. Doses of more than 5,000 rads result in CNS death within 3 days of exposure. Loss of coordination, meningitis, and the signs and symptoms seen in the GI and hematologic syndromes are also present. These types of syndromes were seen in the unfortunate victims of the Chernobyl nuclear power plant accident in 1986.
Local Tissue Damage from Radiation
Any tissue can suffer acute radiation damage if the dose is high enough. Like the acute lethal radiation syndromes, the doses employed in bone densitometry are much too low to cause immediate tissue damage, but it may be necessary for the technologist to reassure the patient that such damage cannot occur.
Radiation Damage to the Skin
Reddening of the skin, or erythema, can follow a single dose of 300–1,000 rads. Persons who have undergone radiation therapy for cancer may have experienced erythema in the course of their therapy. The erythema may be followed by a sloughing of the skin called desquamation. The dose that has been determined to cause erythema in about 50 % of the persons exposed is 600 rads (6 Gy) [7
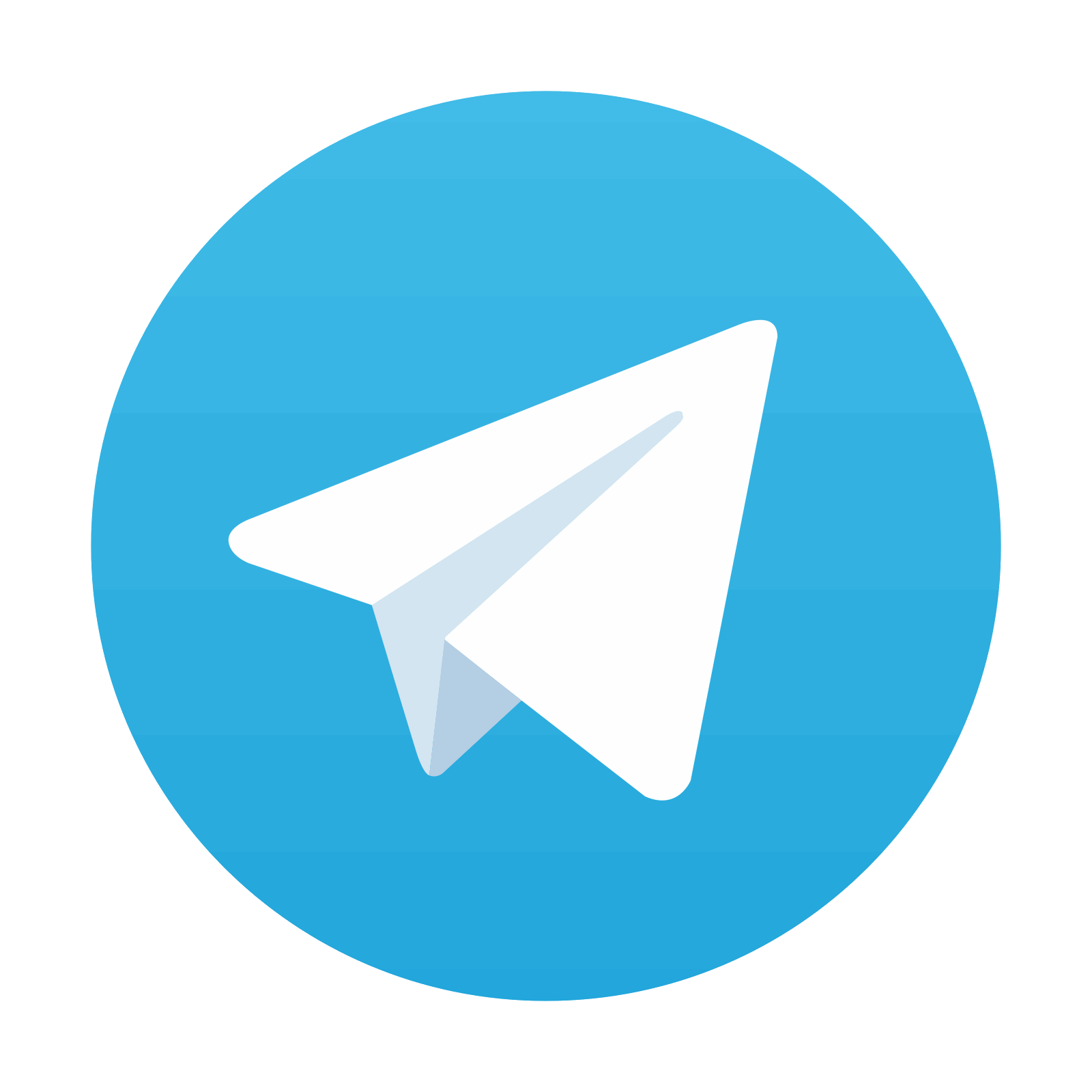
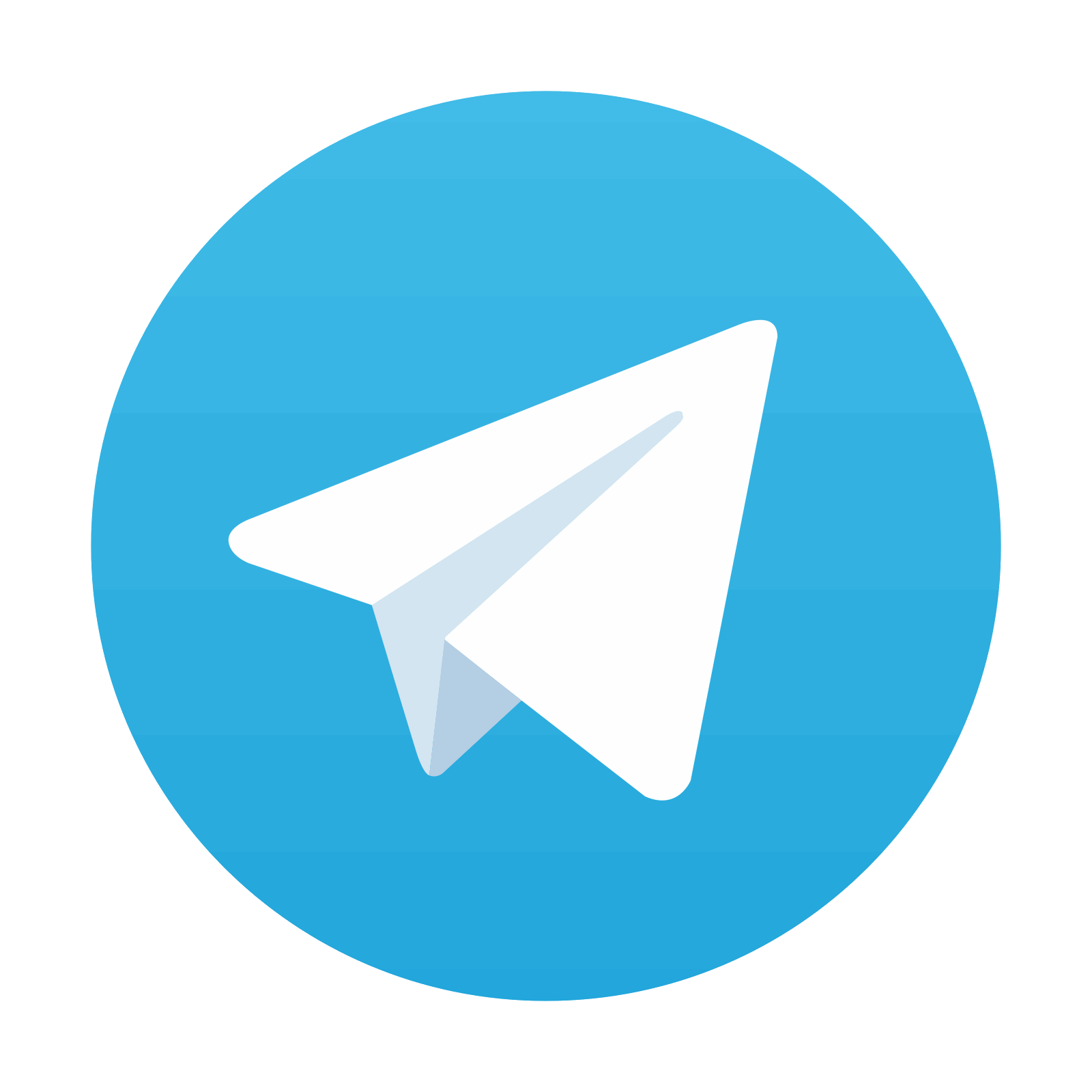
Stay updated, free articles. Join our Telegram channel

Full access? Get Clinical Tree
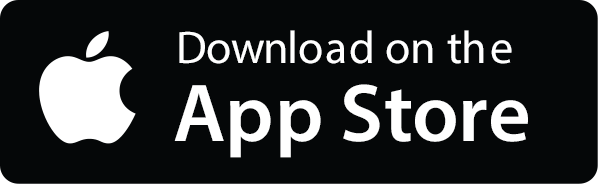
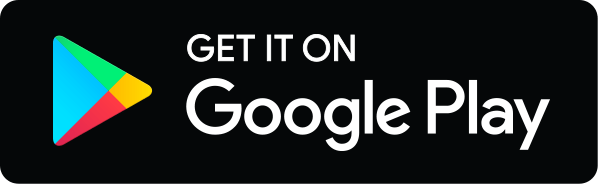
