1 Radiosurgery was initially introduced as a new therapeutic modality for the noninvasive treatment of small intracranial benign vascular lesions. Subsequently, the technology was applied to the treatment of malignant lesions of the brain, including primary and metastatic tumors. More recently, extracranial radiosurgery has been expanded to treat tumors outside the brain, such as those in the spine, head and neck, lung, abdomen, and pelvis. When target lesions are relatively small (i.e., < 2 cm in diameter), large single doses are used to capitalize on the inherent geometric advantage of radiosurgery to limit normal tissue radiation exposure. Target volumes for extracranial radiosurgery often are larger than 2 cm in diameter, resulting in a reduction in the inherent geometric advantage. Like conventional radiotherapy, normal tissue tolerance limits the maximum dose and volume of large single-dose radiosurgery. In an attempt to improve the therapeutic ratio and increase the normal tissue tolerance, delivery of radiosurgery in multiple fractions separated in time may improve efficacy by decreasing late effects of critical normal structures while maintaining superior radiation dose distribution within the tumor tissue. This chapter is primarily devoted to the cellular and molecular processes involved in the killing of tumor and adjacent critical normal tissues after either single or fractionated high-dose radiation. Mammalian cells die through different molecular and cellular mechanisms following exposure of cells to ionizing radiation.1,2 Depending on cell types, irradiated cells undergo (1) reproductive death (mitotic catastrophic death), (2) apoptosis (usually in interphase cell death), or (3) terminal growth inhibition (metabolic death). Reproductive cell death (sometimes called mitotic cell death) becomes the predominant mode of cellular loss in most human tumors (other than lymphoid and germinal tumors) following x-irradiation. The reproductive capacity of tumor cells is quantified using a cell survival curve, a clonogenic assay of single cells’ capacity to undergo multiple replications. Apoptosis is an important mode of cell death in normal tissues and in some tumors, particularly during the acute phase of radiation response. Stem cells of self-renewal normal tissues such as hematopoetic and intestinal crypt cells undergo apoptosis following a moderate dose of radiation exposure. Apoptosis occurs within a few hours to days and can be detected using an array of assays, including observing deoxyribonucleic acid (DNA) laddering on a Western blot; antibody detection in situ or with flow cytometry using any of various DNA fragment labels, such as TUNEL (terminal uridine deoxynucleotidyl transferase mediated dUTP [2′-deoxyuridine 5′-triphosphate] nick end labeling) and Annexin V; and caspase kits, such as caspase 3. In contrast to the significance of apoptosis to the manifestation of the acute phase of tissue response to radiation, the late phase is a result of terminal or permanent growth inhibition of either self-renewing or differentiating and metabolically active cells. Are cell survival curves of human tumor cells in culture relevant to the radiobiology of single high-dose radiosurgery? Tumor physiology is usually more complex than simple in vitro models can reasonably characterize. Nonetheless, some human metastatic lesions in the brain or other organs are well circumscribed without significant infiltration into the adjacent normal tissues and with tumor vasculature less developed than the primary tumors arising in the brain or other organs from which they derive. In fact, cell culture models such as single plated monolayer cells and multicellular spheroids have considerable value in modeling pertinent radiobiological parameters important to the tumor control probability in a wide range of tumors, even though they are not perfect representations of human tumors. Two mathematical constructs widely used to model the relationship between cell survival and radiation dose are the single-hit, multitarget (SHMT) and the linear-quadratic models (also called the alpha/beta [α/β] model). The model characterizes the shape of the best-fit curve using two of three related parameters, Do, Dq, and N, which are related by ln N = Dq/Do. Do is a measure of the slope of the log-linear plot of cell survival and radiation dose that reflects the intrinsic radiation sensitivity of the cells. Dq is almost a threshold dose, below which radiation purportedly has no effect, and is a measure of the breadth of the low-dose shoulder region that reflects the cells’ capacity to repair radiation damage. N, the extrapolation number, is a measure of the number of targets in the cell and also reflects the cells capacity for repair. The SHMT model describes the relationship between the radiation dose, d, and the surviving fraction of cells, SF = S(d)/So, where S(d) is the number of cells that survive a dose of radiation, d, and So is the initial number of cells, SF = 1 – [1 – exp(-d/Do)]N. The linear-quadratic model characterizes the best-fit relationship between SF and d using two parameters, α and β, SF = exp(-αd — βd2). Cell death that is “linearly” proportional to dose (on a semi-logarithmic plot of SF vs d) has been interpreted to be a result of double-strand DNA breaks because such aberrations are lethal and may be caused by the same particle (i.e., photon). Consequently, an increase in dose “linearly” decreases surviving fraction. Cell death that is “quadratically” proportional to dose (on a semi-logarithmic plot of SF vs d) has been interpreted to be a result of single-strand DNA breaks because two such aberrations in close proximity are lethal (more probable at high doses). Consequently, an increase in dose “quadratically” decreases surviving fraction, and the quadratic mode of cell killing dominates at high doses. Interestingly, the ratio of α over β has found utility in characterizing the response of tissues as either acutely responding, those having large magnitudes of the order of 10, and late responding, those having smaller magnitudes of the order of 2. Radiation survival curves of most human tumor cells other than lymphoma or germinal tumors are well fit by the SHMT model with a shoulder region at low doses, and at higher doses the survival curve decreases exponentially with dose. It is interesting to note that most of the heterogeneity of cellular response to radiation is seen at the low-dose region (maximum variation is 10- or 20-fold), but at the higher dose region, that is, doses higher than 8 to 10 Gy, the differences among cell lines become smaller (maximum variation is 2fold). This is an important consideration in the difference between the radiobiology of conventional radiation therapy and the radiobiology of radiosurgery. For example, in the conventional 2 Gy dose fractionation radiotherapy, the total cell kill will be determined with the surviving fraction at the 2 Gy level and magnified to 30 or 35 times, depending on the total dose of radiotherapy, whereas in single-dose radiosurgery, the surviving fraction is mostly determined at the high-dose fraction region. Hence, variation in the total surviving fraction from cell line to cell line may be less with radiosurgery. In fractionated radiotherapy, radiobiological parameters such as repair capacity from the sublethal injury and the rate of reoxygenation after each dose fraction are important determinants to the eventual radiocurability of large solid tumors. On the other hand, important radiobiological determinants in radiosurgery are tumor clonogen numbers and intrinsic radiosensitivity of tumor cells. These two parameters are readily calculated from the cell survival curves. Figure 1.1 shows representative survival curves having different N and Do. It is readily apparent from Fig. 1.1 that the higher the single dose, the more tumor cells killed and that the magnitude of cell kill depends on the intrinsic radio-sensitivity of cells. Table 1.1
Radiobiology of Radiosurgery
Tumor Cell Killing after Single High Dose of Radiation
Cell Survival Curves of Established Human Tumor Cells in Culture
Stay updated, free articles. Join our Telegram channel

Full access? Get Clinical Tree
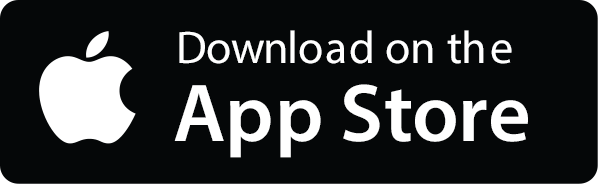
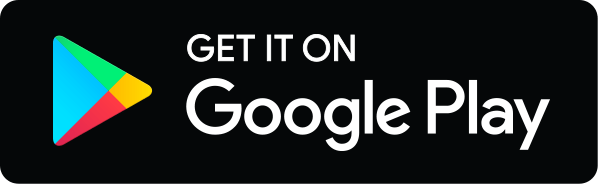