The thyroid produces thyroid hormones that regulate metabolism and are essential to proper development and differentiation of the cells in the body. Iodine is an essential element in thyroid hormone synthesis. The thyroid has the ability to concentrate and incorporate iodine into thyroid hormone. Incorporation of iodine is essential to the formation of thyroid hormone. Thyroid disorders may be imaged and treated with radioiodine. Iodine uptake by thyroid follicular cells lends itself to imaging of functional characteristics of the gland. Gamma camera and, increasingly, PET imaging of thyroid disorders permit delineation of thyroid functional status. Iodine uptake also permits treatment, using I-131, of hyperthyroid disease and cancer, for which I-131 is the primary treatment.
The thyroid is one of the largest endocrine glands. It produces thyroid hormones that regulate metabolism and are essential to proper development and differentiation of the cells in the body. Iodine is an essential element in thyroid hormone synthesis. The thyroid has the ability to concentrate and incorporate iodine into thyroid hormone. Incorporation of iodine is essential to the formation of thyroid hormone.
Radioactive iodine is one of the first radioisotopes to be used in medicine. Radioactive iodine is taken up and incorporated in an identical manner as nonradioactive iodine by the thyroid gland. It has been used to evaluate and treat benign and malignant thyroid diseases for more than 50 years.
The following discussions refer to the patient population in the United States.
Instrumentation
Most radioiodine imaging is currently performed using a gamma camera. The most commonly used radioactive isotopes of iodine are iodine-123 (I-123), which emits only gamma rays (also called photons) with an energy of 159 KeV, and iodine-131 (I-131), which has a complex decay scheme that includes photons of 364 KeV. Radioactive iodine is usually administered orally. It accumulates in the thyroid and is best visualized 4 hours or more after administration. Photons emitted from the thyroid enter the gamma camera and interact with the crystal to produce scintillations that are detected, amplified, and converted into light. An image of radioactive iodine distribution is thus created. Modern cameras have designs that are digital, with improved efficiency in photon capture. Because the photon energies of I-123 and I-131 are different, they require different collimators—low energy collimation for I-123 and high energy for I-131.
Fig. 1 illustrates the basic principles of a gamma camera.
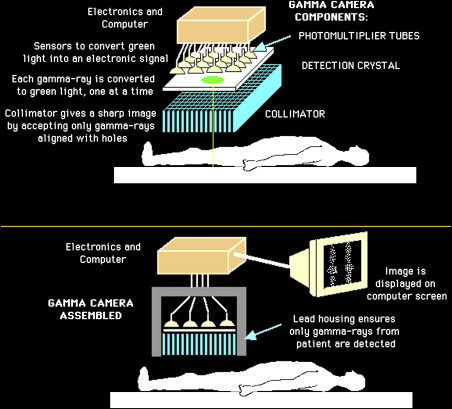
Patient preparation
All patients presenting for radioiodine imaging or therapy should discontinue use of iodine-containing supplements or medications that can potentially affect the ability of iodine uptake and incorporation by the thyroid gland. Most centers also recommend a low iodine diet for 7 to 14 days before radioiodine.
For patients who have thyroid cancer, the optimal condition for imaging and treatment is elevation of serum thyrotropin (TSH) level. This elevation can be achieved endogenously by withdrawing thyroid hormone and waiting until the serum TSH is 30 mIU/L or greater. Elevation of TSH can also be achieved exogenously by administration of recombinant human TSH (rhTSH), given intramuscularly 0.9 mg on two consecutive days. It is indicated in patients who cannot be prepared by total thyroidectomy, or in the presence of sufficient functioning tumor, or with pituitary insufficiency, or in patients who cannot tolerate prolonged hypothyroidism. The recent FDA approval of rhTSH for the diagnosis and treatment of patients who have thyroid cancer has increased its use, although the cost is high. It is also increasingly used routinely for follow-up imaging in low-risk patients.
For diagnostic purposes, radioactivity is given to women of childbearing age only if the patient reports not being pregnant. Therapeutic radioactivity is given to women of childbearing age within 48 hours of a negative serum β-HCG test. Pregnancy status does not need to be checked if the woman is postmenopausal (defined by convention as no menstrual period for at least a year), or the woman has been rendered incapable of childbearing surgically or medically. Following radioiodine therapy, women should not become pregnant until the medical condition has been optimized. Some centers suggest a waiting period of 6 months and 12 months for hyperthyroidism and thyroid cancer, respectively, after radioiodine treatment. Because iodide is also a component of milk, lactating women undergoing radioiodine therapy should be asked to stop breastfeeding until accumulation in the breasts is minimal. Therapy should be delayed whenever possible until lactation ends, failing which frequent expression of milk is recommended to minimize radiation to the breasts.
Elderly or cardiac patients who have Graves disease may require antithyroid medications before radioactive iodine treatment so as to minimize the risk for excessive hormone release due to radiation thyroiditis.
Patient preparation
All patients presenting for radioiodine imaging or therapy should discontinue use of iodine-containing supplements or medications that can potentially affect the ability of iodine uptake and incorporation by the thyroid gland. Most centers also recommend a low iodine diet for 7 to 14 days before radioiodine.
For patients who have thyroid cancer, the optimal condition for imaging and treatment is elevation of serum thyrotropin (TSH) level. This elevation can be achieved endogenously by withdrawing thyroid hormone and waiting until the serum TSH is 30 mIU/L or greater. Elevation of TSH can also be achieved exogenously by administration of recombinant human TSH (rhTSH), given intramuscularly 0.9 mg on two consecutive days. It is indicated in patients who cannot be prepared by total thyroidectomy, or in the presence of sufficient functioning tumor, or with pituitary insufficiency, or in patients who cannot tolerate prolonged hypothyroidism. The recent FDA approval of rhTSH for the diagnosis and treatment of patients who have thyroid cancer has increased its use, although the cost is high. It is also increasingly used routinely for follow-up imaging in low-risk patients.
For diagnostic purposes, radioactivity is given to women of childbearing age only if the patient reports not being pregnant. Therapeutic radioactivity is given to women of childbearing age within 48 hours of a negative serum β-HCG test. Pregnancy status does not need to be checked if the woman is postmenopausal (defined by convention as no menstrual period for at least a year), or the woman has been rendered incapable of childbearing surgically or medically. Following radioiodine therapy, women should not become pregnant until the medical condition has been optimized. Some centers suggest a waiting period of 6 months and 12 months for hyperthyroidism and thyroid cancer, respectively, after radioiodine treatment. Because iodide is also a component of milk, lactating women undergoing radioiodine therapy should be asked to stop breastfeeding until accumulation in the breasts is minimal. Therapy should be delayed whenever possible until lactation ends, failing which frequent expression of milk is recommended to minimize radiation to the breasts.
Elderly or cardiac patients who have Graves disease may require antithyroid medications before radioactive iodine treatment so as to minimize the risk for excessive hormone release due to radiation thyroiditis.
Isotopes
The most common radioactive iodine isotopes in clinical use today are I-123 and I-131. I-123 is produced in a cyclotron and decays by electron capture with a half-life of 13.6 hours. It emits gamma ray photons at 159 keV. I-131 is produced in a generator and has a half-life of 8.05 days. It emits 364 keV photons and beta particles with an average energy of 192 keV. I-131 can thus be used for both imaging and therapy. I-123 has better imaging characteristics for gamma camera imaging; however it is more expensive.
Tc-99m pertechnetate is also trapped by thyroid follicular cells and can be used for thyroid scintigraphic imaging. Unlike radioactive iodine, Tc-99m pertechnetate is not incorporated into thyroid hormone. It is therefore not used for functional evaluation of the thyroid. In rare situations, a hypofunctioning nodule on radioactive iodine imaging may have normal uptake of Tc-99m pertechnetate, creating a “discordant nodule.”
Table 1 summarizes the salient physical and radiation absorbed dose characteristics of the commonly used isotopes. The radiation absorbed dose to patients varies depending on thyroid uptake.
Radionuclide | Half-life | Primary Photon (KeV) | Beta Energy (Average) | Radiation Absorbed Dose (cGy/μCi) |
---|---|---|---|---|
Tc-99m | 6 h | 140 | None | 0.0002 |
I-123 | 13 h | 159 | None | 0.013 |
I-131 | 8 d | 364 | 192 KeV | 0.13 |
I-124 is a positron emitter. It emits positrons in approximately 23% of decays, with an average energy of 819 keV. It has a half-life of 4.18 days. Imaging of thyroid disorder with I-124 positron emission tomography (PET) is still experimental but seems extremely promising. Its cost is also currently high, precluding routine use.
Imaging and treatment of benign thyroid disorders
Thyroid nodules, signs and symptoms of hyper- or hypothyroidism, are the most common benign thyroid disorders evaluated using nuclear medicine techniques.
A sensitive serum TSH assay is an integral part of such evaluation, being the most sensitive test for confirming clinically apparent and mild subclinical thyroid hormone excess or deficiency. This test is usually a component of thyroid function tests, which typically include serum thyroxine (T4) measurements. Most thyroxine is protein-bound. Other laboratory tests may include free T4 and triiodothyronine (T3) and free T3, especially if the patient is clinically hyperthyroid and has low/undetectable serum TSH. Thyroid autoantibodies, such as TSH receptor antibodies, thyroid-stimulating immunoglobulins, and antithyroperoxidase, are also sometimes obtained to determine possible autoimmune causes for thyroid disorders.
Radioiodine imaging for benign thyroid disorders is performed with 0.2 to 0.5 mCi I-123 or 0.02 to 0.05 mCi I-131. Some nuclear medicine physicians use 2 to 5 mCi Tc-99m pertechnetate for imaging the thyroid, whereas thyroid radioiodine uptake is measured with I-123 or I-131.
Diffuse Goiter
Hyperthyroid
Graves disease
The most common cause for hyperthyroidism is Graves disease, also called toxic diffuse goiter. Autoantibodies against TSH receptors on cells of thyroid develop in patients who have Graves disease, thereby stimulating the thyroid cells and increasing thyroid hormone production. The patients usually have symptoms associated with hyperthyroidism and some patients may also develop thyroid ophthalmopathy. Laboratory abnormalities include suppressed TSH and elevated T3 and T4. As seen in Fig. 2 , radioiodine imaging shows a diffusely enlarged thyroid gland with increased uptake. A pyramidal lobe can also be seen sometimes. Some patients may have a normal-sized gland with increased uptake.
I-131 is the treatment of choice for Graves disease. Physicians usually prefer to treat the patients with I-131 with a dose to render the patients hypothyroid, because calculation of a dose that would result in a euthyroid state is difficult; treatment effects can be prolonged, with a variable time to euthyroid or hypothyroid state, and it is easier to treat hypothyroidism than hyperthyroidism. The patients subsequently require thyroid hormone replacement for life.
Subacute and silent thyroiditis
Patients who have subacute or silent thyroiditis can present with diffuse goiter and thyrotoxicosis during the inflammatory phase. They are distinguished from Graves disease by decreased radioactive iodine uptake and minimal thyroid activity on radioiodine imaging ( Fig. 3 ). The thyrotoxicosis results from release of stored thyroid hormone secondary to infiltration and disruption of the thyroid follicles by the giant cells in subacute thyroiditis and lymphocytes in silent thyroiditis. These two disorders can be further distinguished by their different clinical presentation. Patients who have subacute thyroiditis often present with neck pain and report a history of preceding viral (usually upper respiratory) infection. Patients who have silent thyroiditis usually do not have gland tenderness. Postpartum thyroiditis is a subtype of silent thyroiditis in the postpartum period.
Subacute thyroiditis is treated with nonsteroidal anti-inflammatory drugs and steroids in more severe cases. Silent thyroiditis is a self-limiting disorder that usually does not require treatment.
Hypothyroid
Chronic thyroiditis
Chronic thyroiditis is a common cause of hypothyroidism. The most common cause of primary hypothyroidism is chronic autoimmune thyroiditis, or Hashimoto thyroiditis. Hashimoto thyroiditis has a wide range of clinical presentations, laboratory results, and imaging findings. The symptoms are related to duration and severity of hypothyroidism, the time course of development of hypothyroidism, and psychologic characteristics of the patient. The thyroid may be enlarged, atrophic, or normal size, and it may contain nodules. The nodules may infrequently harbor thyroid cancer or lymphoma. Laboratory tests, sometimes discordant, may reveal increased TSH and decreased free T4 in overt or clinical hypothyroidism, or increased TSH and normal free T4 and T3 in subclinical hypothyroidism. Thyroid autoantibodies are positive in 95% of patients who have Hashimoto thyroiditis.
Clinical hypothyroidism is treated with levothyroxine replacement. Treatment of subclinical hypothyroidism with levothyroxine is controversial.
Subacute and silent thyroiditis
Subacute and silent thyroiditis often follow a triphasic course. They have a period of hypothyroidism following initial stage of thyrotoxicosis, which is then followed by recovery to euthyroid state. During the period of hypothyroidism, there is often discordance between the laboratory values and clinical status. Radioiodine thyroid uptake usually remains minimal and there is minimal thyroid activity on radioiodine imaging. During the recovery phase, however, there may be diffusely increased uptake that may mimic Graves disease. In thyroiditis, this period is transient and is followed by returning to baseline. Repeat imaging and discordant laboratory values help make this distinction.
Nodule Evaluation
Thyroid nodules are common. It is estimated that about 4% to 7% of the United States population aged 50 years and older have clinically apparent thyroid nodules. More than 50% of thyroids have nodules when examined on ultrasound. Thyroid nodules may be solitary or multiple. On ultrasound, the nodules may be cystic or solid or mixed cystic and solid. A thyroid with a single palpable nodule on clinical examination may contain multiple nodules on ultrasound or other imaging modalities.
A multinodular goiter is a clinical diagnosis made on physical examination. Multinodular goiter is found to be more prevalent in endemic areas, believed to be related to goitrogenic substance intake or iodine deficiency.
Most thyroid nodules are benign. About 5% of thyroid nodules are malignant. The risk for cancer is probably not significantly different between solitary nodule and multinodular goiter. The primary purpose of evaluation of the thyroid nodules is to exclude malignant lesions. Although ultrasound remains the mainstay of thyroid nodule evaluation, thyroid scintigraphy can be used in special situations to aid the evaluation.
According to the American Association of Clinical Endocrinologists and Association Medici Endocrinologi Medical Guidelines for Clinical Practice for the Diagnosis and Management of Thyroid Nodules, thyroid scintigraphy is indicated for the following:
Single thyroid nodule or multinodular goiter (MNG) and suppressed serum TSH
Large MNG, especially with substernal extension
In search of ectopic thyroid tissue (for example, struma ovarii or sublingual thyroid)
In subclinical hyperthyroidism to identify occult hyperfunctioning tissue
Some investigators suggest evaluation of follicular neoplasms with a scintiscan to identify a functioning cellular adenoma that may be benign; however, most such nodules are cold on a scintiscan. On thyroid scintigraphy, nodules can be hyperfunctioning (ie, “hot”) or can be hypofunctioning (ie, “cold”). Hot nodules are almost always benign. There is a 5% to 8% risk for malignancy in cold nodules
Solitary nodule
Hyperfunctioning nodule
A solitary hyperfunctioning nodule is frequently caused by hyperfunctioning thyroid adenoma. The risk for thyrotoxicity is increased with nodule size. Most hyperfunctioning nodules are autonomous (not under the regulation of TSH). An autonomously hyperfunctioning nodule can suppress iodine uptake by the rest of the gland, as seen in an example in Fig. 4 .
