Fig. 4.1
Sequence of events following radiation exposure . The chart is divided into three parts by dashed lines suggesting events and reactions that might be modified by radiation protectors (top), radiation mitigators, and treatment (bottom). Reproduced from Citrin et al. [5]
Radioprotectors
A radioprotector is an agent that prevents the damage caused by radiation, generally, by scavenging the free radicals that cause DNA oxidation and DNA double strand breaks [4]. Because these agents prevent the damage from occurring, they must be given before or at the time of the radiation exposure. Free radicals have an extremely short half-life and, as a result, a limited range for diffusion. Thus, radioprotectors must have the ability to cross the nuclear membrane and accumulate near DNA. This accumulation allows scavenging of radicals that would otherwise lead to lethal DNA damage.
In order for a radioprotector to enhance the therapeutic ratio, the agent must selectively protect normal tissues from irradiation (Fig. 4.2). If the agent protects both normal and tumor tissues, there is no change in the ratio and hence, no benefit to the delivery of the agent [5]. The agent may selectively protect normal tissue through a variety of mechanisms. For example, the radioprotector may be activated or taken up by normal tissue more effectively than tumor tissue, leading to a higher concentration of the agent in normal tissue . Conversely, the agent may be cleared or metabolized more rapidly by tumor tissue, also leading to a higher concentration of the agent in normal tissue.
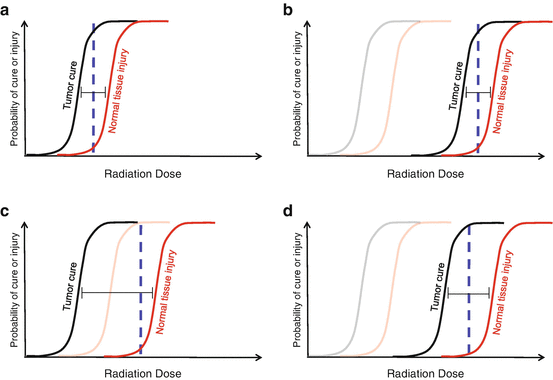
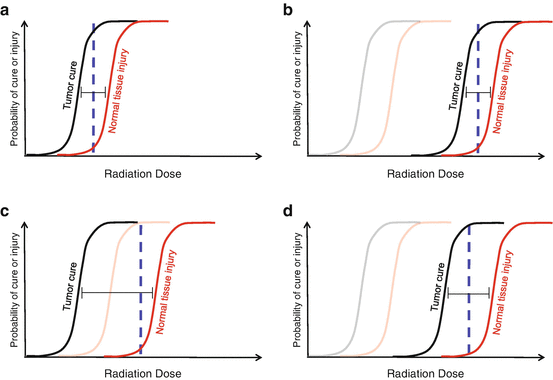
Fig. 4.2
Effects of radioprotectors on the therapeutic window . (a) The chosen treatment dose (blue vertical line) delivers a high chance of tumor cure (black) with a small chance of normal tissue injury (red). (b) A nonselective radioprotector indiscriminately protects tumor and normal tissue shifting both the tumor cure and normal tissue injury curve to the right. Consequently, the therapeutic window remains unchanged. Shifted curves are shown in red and black. The original curves are shown in gray and pale red. (c) A true selective radioprotector exclusively protects normal tissue and, thus, shifts only the normal tissue injury curve to the right. This affords a larger therapeutic window such that a higher dose can be given to achieve increased tumor cure with equal or less injury. A lower dose producing the same tumor probability can also be given with less tissue injury. (d) Some nonselective radioprotectors can protect the normal tissue to a greater extent shifting the normal tissue curve further to the right than the tumor cure curve and resulting in an increased therapeutic window
Antioxidants are molecules that reduce cellular damage caused by free radicals . Some examples of antioxidants are ascorbic acid, polyphenols, and thiols. These low molecular weight antioxidants produce a more stable reactive species by donating a hydrogen atom to free radicals. Most radioprotectors are also antioxidants [5]. Of note, however, not every antioxidant has radioprotective effects [6, 7], as they are not all reactive toward the secondary species generated by radiation [7]. In addition to small molecule antioxidants, some antioxidants exist in the form of enzymes, such as superoxide dismutase, catalase, and glutathione peroxidase [5].
Amifostine
Amifostine is a thiol compound that scavenges free radicals, and it is the only FDA-approved radioprotector . Clinically, it has been administered to head and neck cancer patients receiving radiotherapy to prevent xerostomia (dry mouth) [8]. Amifostine is a prodrug that is only activated when dephosphorylated by alkaline phosphatase, a cell membrane protein [9]. It selectively protects normal tissue as it preferentially accumulates in normal tissue rather than tumor tissue [10]. It is thought that the hypovascularity and low pH of the tumor microenvironment limits the activation of amifostine. Furthermore, tumors have lower levels of alkaline phosphatase than normal tissues [11]. In addition to scavenging free radicals, amifostine metabolites induce hypoxia by increasing oxygen consumption [12, 13], which further protects tissues in which the metabolites concentrate.
Amifostine has been tested extensively in clinical trials in various cancer types for the prevention of both acute and late injury. At least 30 different studies have evaluated the use of amifostine in preventing oral mucositis, and the results from these studies have been conflicting. A systematic review of these studies found that data supporting the use of amifostine for oral mucositis was inconclusive [14]. In non-small lung cancer , several small studies showed that amifostine minimized esophagitis [15–17]. However, a study with a larger number of patients receiving chemoradiotherapy was unable to support a reduction in physician-assessed esophagitis; however, amifostine was reported to ameliorate patient-reported swallowing impairment and pain [18, 19]. Amifostine has been shown in some series to be effective against proctitis and dermatitis in patients with pelvic malignancies who underwent radiotherapy [20–22]. It has also been effective in reducing soft tissue [23] and lung fibrosis [23, 24] in patients receiving radiation.
Despite a large number of trials that have been conducted to evaluate amifostine, its usefulness is limited. Many of the studies were conducted with a small and heterogeneous patient population, and dosing schedules differed between studies. In addition, there are several limitations associated with the use of the drug. To be effective, amifostine must be given 15–30 min before radiation and is only approved for intravenous delivery, which may be logistically challenging. Systemic delivery of amifostine is also associated with several side effects, including nausea, vomiting, sleepiness, and low blood pressure. Finally there is much debate over the use of amifostine with radiotherapy and chemoradiotherapy not only because of its side effects but because there are concerns that it may reduce the effectiveness of radiation treatment.
Nitroxides
Nitroxides are recycling antioxidants that have been shown to prevent cytotoxicity induced by oxidative stress as well as by radiation. Nitroxides interconvert between the oxidized and reduced form. In their oxidized state, nitroxides are a stable free radical referred to as a nitroxide radical. These radicals undergo hydrogen reductions to generate hydroxylamine. Both nitroxide radicals and hydroxylamine have antioxidant functions [7, 25]; however only nitroxides exhibit radioprotective effects. In vitro studies using various cell types have shown that nitroxides can reduce DNA damage and cell death induced by radiation [26, 27]. More importantly, systematic administration of nitroxides to mice resulted in decrease lethality after total body irradiation exposures, further substantiating their therapeutic potential [28, 29].
One of the more clinically promising nitroxides is tempol (4-hydroxy-2,2,6,6-tetramethylpiperidine-1-oxyl). Tempol has been studied as a radioprotector in both topical and systemic applications. Topical application of tempol to the skin of guinea pigs exposed to single and fractionated doses of radiation was capable of ameliorating alopecia [30, 31]. Systemic administration of tempol was capable of reducing the lethality of total body irradiation exposures [28, 29]. Systemic administration of tempol has also been shown to protect salivary glands from radiation [32].
As mentioned previously, to enhance the therapeutic ratio, a radioprotector must selectively protect normal and not tumor tissue. The selectivity of tempol for normal tissues has been addressed in several preclinical studies. Systemic administration of tempol in tumor-bearing mice had no effect on tumor growth, and administration with radiation had no impact on the dose of radiation that results in cure in 50 % of tumors at 30 days after treatment [33]. However, systemic administration of tempol was capable of protecting salivary glands and the skin from irradiation [32].
The differential effect of tempol in normal and tumor tissue has been hypothesized to relate to faster reduction to the hydroxylamine metabolite in tumor compared to normal tissues [33]. This hypothesis was evaluated by temporally tracking nitroxide levels with magnetic resonance imaging (MRI) . Tempol in its oxidized form acts as contrast agent and can be imaged by MRI. As tempol reduces into hydroxylamine , the contrast enhancement decreases because in its reduced form, it does not provide T1 contrast. By following the intensity of contrast enhancement over time, it was deduced that tempol is reduced faster into its non-radioprotective form in the tumor compared to surrounding normal tissue (Fig. 4.3) [34]. The unique ability to image tempol may allow a determination of the optimal time of tempol delivery and may further allow the unique opportunity to test this relationship in each patient to be treated.
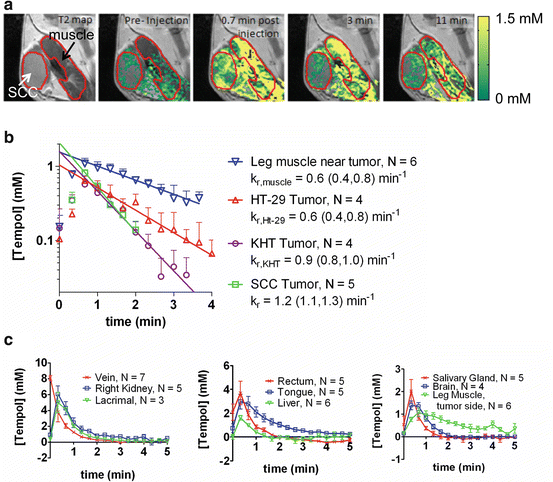
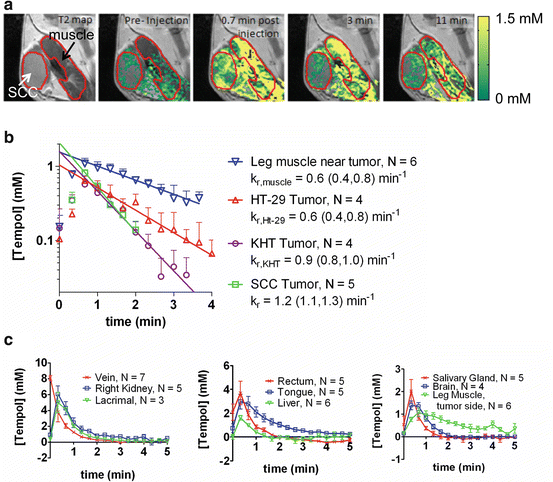
Fig. 4.3
Results from a redox imaging experiment of tumor and salivary glands. (a) Concentration maps overlaid on T2-weighted images corresponding to the hind leg region of a mouse. The tumor and the adjacent leg muscle are outlined in red. (b) The average tempol concentration inside the muscle and tumor was plotted as a function of time after injection. The concentration of tempol was determined in three different tumor models: SCCVII, KHT, and HT-29. For each time point after injection (20 s intervals), the average concentration was determined for each tissue. (c) Using the same technique as used in a and b, the concentration of tempol was determined in nine noncancerous tissue compartments. The error bars represent the standard error of the mean, and the lines connect the data points. Note the difference in tempol concentration in tumor compared to normal tissues as a function of time. Reproduced from Davis et al. [34]
Clinical translation of tempol has met with initial success. In a phase I clinical trial, tempol was effective at reducing alopecia in patients who underwent whole-brain radiotherapy [35]. Pharmacokinetic studies found that tempol was only detectable in 50 % of plasma samples after topical application and that the levels were minimal in those in which it was detected, suggesting that tumor protection via systemic leak was not a major concern in patients treated with topical tempol.
Other Candidate Radioprotectors
Naturally occurring antioxidants have also been tested for their efficacy as radioprotectors. Antioxidants such as α-tocopherol (vitamin E) and β-carotene have been demonstrated to reduce various radiation-induced injuries including xerostomia [36] and mucositis [37, 38], and in combination with pentoxifylline , α-tocopherol has been shown to reduce lung fibrosis [39, 40]. The use of these nutritional antioxidants as radioprotectors has come under question due to concerns that these agents may also interfere with tumor control either through radioprotection or via enhancement in the rate of second malignancies. Combined α-tocopherol and β-carotene supplementation given during and after radiation was also shown to increase the local recurrence rate of head and neck tumors [37]. These findings highlight the need to consider the possibility of tumor radioprotection carefully.
One promising antioxidant that could be an effective radioprotector is superoxide dismutase (SOD) . SOD is an endogenous enzyme that converts superoxide into oxygen and hydrogen peroxide. Transgene expression of SOD in animal models via gene therapy has been shown to protect against mucositis [41], esophagitis [42], and pneumonitis [43, 44]. Furthermore in animal models , SOD was demonstrated to selectively protect normal and not tumor tissue [45]. The major concern with this approach is the ability of SOD to access the primary target of radaition, DNA.
Melatonin is a hormone that has been shown in a number of studies to have radioprotective effects . It has the ability to directly scavenge free radicals and to increase the expression levels of antioxidant enzymes, including SOD, glutathione peroxidase, and catalase. It also has been reported to increase the efficiency of mitochondrial function, thereby reducing ROS levels generated by the electron transport chain [46]. In animal models, melatonin has been demonstrated to protect mice against lethal total body exposures [47, 48] and to protect a number of organs from radiation injury [49]. In vitro studies have demonstrated that melatonin sensitizes cancer cell lines to irradiation [50, 51].
Despite the fact that preclinical data supports that melatonin is a selective radioprotector, a phase II trial did not confirm activity in this regard. A Radiation Therapy Oncology Group (RTOG) trial randomized patients with brain metastases into two groups: morning versus nighttime high-dose melatonin. Melatonin was given to the patients during and following radiation . Results from this study were compared to historical controls who received only whole-brain radiotherapy. It was concluded that melatonin improved neither overall survival nor neurocognitive function after radiotherapy [52]. More recently, several in vitro studies have shown that melatonin at pharmacologic doses may actually have oxidant effects [53–55]. This finding has yet to be confirmed in vivo; nevertheless, it suggests caution and careful consideration of dosing is necessary when combining melatonin with radiotherapy .
Radiation Mitigators
Radiation mitigators reduce normal tissue damage after exposure to radiation through a variety of mechanisms. Unlike radioprotectors, which prevent damage from occurring, radiation mitigators minimize damage by acting upon physiologic processes that occur after radiation exposure but before the clinical manifestation of injury [4]. Radiation mitigators may be used to ameliorate both acute and late toxicities. Acute radiation toxicities, such as dermatitis and mucositis, are often caused by the death of rapidly dividing cells [56]. Damage to the rapidly proliferating stem cell compartment of organs, such as the skin and small intestine, eventually leads to loss of differentiated, specialized cells and results in the manifestation of tissue damage [57, 58]. Therefore, mitigators that effectively prevent acute injury often promote stem cell survival and proliferation.
Although the cellular response to radiation is almost immediate, the expression of late toxicities may be delayed for months to years after radiation exposure. Radiation activates a myriad of different signaling pathways that initiate pro-inflammatory, profibrotic, and vascular injury responses. These responses continue long after the initial radiation exposure and result in altered tissue homeostasis, fibrosis, vascular damage, atrophy, and necrosis [59]. Many radiation mitigators target key molecules or processes in the pathways that lead to late radiation injury. It is impossible to review every radiation mitigator identified in the space allowed. Thus, we have highlighted clinically approved or notable examples in the sections below.
Total Body Exposures
Normal tissue stem cells are responsible for regenerating tissues damaged by radiation and other processes. Stem cells are highly sensitive to radiation and are typically depleted in the radiation-damaged tissues [58, 60, 61]. Thus, a great deal of research has evaluated cytokines and growth factors that have the capacity to promote stem cell survival and proliferation. These approaches have been explored primarily as a method to reduce acute toxicity after localized and total body exposures.
Total body exposures can cause death through bone marrow failure and loss of intestinal integrity (gastrointestinal syndrome) [56]. At extreme doses death results from damage to the central nervous system and vascular collapse [56]. Intestinal damage is also a cause of major morbidity among transplant patients treated with total body irradiation. Many mitigant strategies for total body exposures are therefore geared toward preventing bone marrow failure and gastrointestinal syndrome by stimulating stem cell function. Intestinal injury is also a major dose-limiting factor during abdominal and pelvic radiation.
One example of this approach is the use of granulocyte colony-stimulating factor (G-CSF) as a radiation mitigant. In nonhuman primates , administration of G-CSF at 6 h after a total body exposure led to increased hematopoietic recovery [62]. In mice, administration of two separate doses of G-CSF at 24 h and 30 min before total body irradiation increased survival rates. Interestingly, there was no effect on survival when G-CSF was given 24 h after irradiation [63] suggesting that for the G-CSF to be effective, it must be given close to when radiation DNA damage occurs. This type of treatment may be useful in the setting of accidental total body exposures or in therapeutic exposures that require irradiation of extensive marrow compartments that are uninvolved by tumor . The use of G-CSF with therapeutic radiation must be approached with caution as G-CSF has been reported to drive epithelial to mesenchymal transition and tumor progression [64]. G-CSF is considered an effective treatment for accidental total body exposures and is part of the US Strategic National Stockpile [65].
Another agent in the stockpile, entolimod , is a toll-like receptor 5 (TLR5) agonist that has been shown to have mitigant efficacy in animal models of lethal total body exposures , dermatitis, and mucositis [66, 67], with no evidence of impaired tumor response [66, 67]. Entolimod, formerly known as CBLB502 , is a Salmonella flagellin derivative that activates the NF-kB pathway by binding toll-like receptor 5 (TLR5). It is more potent but less immunogenic and toxic compared to purified flagellin [67]. At high radiation doses, delivery of entolimod in mice shortly before irradiation led to reduced gastrointestinal and hematological injury and subsequent improvement in survival. Enhanced survival was also observed with postirradiation delivery of entolimod but only at low radiation doses [67]. Efficacy of entolimod was further tested in nonhuman primates, and data showed that entolimod protected primates from hematopoietic and lymphoid organ damage and lethality incurred by radiation [67]. G-CSF and interleukin-6 (IL-6) are two potential biomarkers for entolimod efficacy in mitigating radiation-induced injury . In irradiated and nonirradiated animals, levels of these cytokines were stimulated by entolimod in a TLR5- and dose- dependent manner. Furthermore, inhibiting G-CSF and IL-6 with neutralizing antibodies blocked the radiation mitigating effects of entolimod suggesting that the two cytokines are major mediators of entolimod’s mechanism of action [68]. The discovery of these two biomarkers may aid in determining the most optimal, efficacious dose to use in humans. Currently, entolimod is being evaluated in clinical trials for its capacity to mitigate radiation injury.
Palifermin is a truncated recombinant human keratinocyte growth factor (KGF) . KGF is a mitogenic factor with diverse functions in proliferation, survival, differentiation, DNA repair, and reactive oxygen species detoxification [69]. Animal studies have shown that administration of palifermin can reduce xerostomia [70], mucositis [71], and gastrointestinal injury [72, 73] induced by radiation. Palifermin is FDA approved for prevention of severe mucositis in hematologic cancer patients who receive chemoradiotherapy prior to stem cell transplant [74]. Additionally, a phase II clinical trial for head and neck cancer found that delivery of palifermin to patients receiving concurrent chemotherapy and hyperfractionated radiotherapy minimized the incidence, severity, and duration of oral mucositis [75]. In the same study, it was concluded that palifermin had no effect on survival or progression-free survival [75], suggesting an absence of tumor-promoting effects . The selectivity of palifermin for normal tissue may depend on whether or not the fibroblast growth factor receptor 2b (FGFR2b) , a cognate receptor for KGF, is expressed in tumor tissues. Preclinical studies have shown that KGF-FGFR2b signaling can promote tumor and metastatic phenotypes in breast, lung, and gastric cancer [76].
Because inflammation and vascular damage are two immediate radiation responses that can persist and cause late tissue damage, there has been much interest in mitigators that target inflammatory pathways. One such pathway is the thrombomodulin (THBD)-activated protein C (APC) . THBD is a transmembrane glycoprotein that binds thrombin and activates thrombin activatable fibrinolysis inhibitor (TAFI) [77]. In addition, it cleaves and activates the protein C zymogen , which in turn inactivates blood coagulation factors V and VIII via proteolysis [78]. The anticoagulant APC also promotes fibrinolysis and induces anti-inflammatory and cytoprotective activities in endothelial, neuronal, and innate immune cells [78]. In clinical and preclinical studies, it has been shown that THBD is significantly reduced and never recovered in intestinal endothelial cells after radiation [79–81]. More interestingly, systematic delivery of soluble THBD and APC in mice exposed to total body irradiation resulted in protection from hematological injury and lethality [82]. Numerous candidate mitigants that expand hematopoiesis or gastrointestinal recovery after total body exposures have been studied, such as metformin, lysophosphatidic acid mimics, genistein, and GSK-3 inhibitors [83–89].
Dermatitis
Radiation dermatitis is an acute toxicity of radiotherapy that can cause pain, increase the risk of infection, and result in the need for treatment breaks. Radiation dermatitis typically begins as erythema and progresses with increasing skin dose to induration, dry desquamation, moist desquamation, and finally to ulceration [56]. A number of agents have been tested as treatment for radiation dermatitis in small randomized trials. Several of these agents have been found to reduce the severity of radiation dermatitis to some degree [90–94]. Despite these findings, these agents are not in widespread clinical use at this time, and many patients are only treated with topical emollients for symptomatic relief. Mometasone furoate and betamethasone , topical steroids , have been shown in randomized trials to reduce the severity of radiation dermatitis [95, 96].
Fibrosis
Fibrosis is a common type of late tissue injury associated with radiation. TGF-β is the predominant signaling pathway that drives fibrosis [59], and accordingly, many radiation mitigators that target this pathway have been evaluated. Neutralizing antibodies against TGF-β have been shown to minimize fibrosis in animal models following radiation [97]. Halofuginone , a small molecular inhibitor that targets TGF-β signaling, also had similar protective effects in mice and showed normal tissue selectivity as it had no effect on tumor radiosensitivity [98]. Downstream molecules of the TGF-β signaling pathway, such as Smad3, have also been demonstrated to be possible therapeutic targets [99, 100].
It is noteworthy to mention that TGF-β has opposing, dual roles in cancer. In the early stages of cancer, it inhibits tumorigenesis but promotes metastasis as the disease progresses [101, 102]. Therefore, determining the appropriate dosing schedule for anti-TGF-β agents may be crucial in achieving therapeutic gain. Currently, there are no TGF-β-targeting agents in clinical trials specifically for mitigation of radiation-induced fibrosis, although there are several for fibrotic disease and scarring [103].
Statins are HMG-CoA reductase inhibitors that are traditionally used in clinic to lower cholesterol levels. Data from preclinical studies however suggest that statins may also be effective as a radiation mitigator for late and acute injury. In mice that received whole-lung irradiation, lovastatin was shown to reduce thrombopenia and mRNA levels of several pro-inflammatory and profibrotic genes including TNFα, IL-6, TGF-β, and connective tissue growth factor (CTGF) [104]. Lovastatin in a separate study was also shown to attenuate lung fibrosis and increase survival [105]. Moreover, other statins such as pravastatin were shown to attenuate CTGF expression and intestinal radiation fibrosis [106]. The mechanism by which statins regulate late tissue injury is unclear, but it has been hypothesized that it may involve the Rho pathways as inhibition of this pathway resulted in similar protective effects as seen with pravastatin [107, 108]. Statins are also known to inactivate Rho-GTPases further supporting the hypothesis [109]. Whether or not statins are safe to use during tumor radiotherapy remains to be determined. Several reports have shown that statins enhance the radiation cytotoxic effects in tumor tissue [110, 111], but further evaluation is needed.
Activation of the PDGF pathway has been implicated in the progression of radiation fibrosis. PDGFR inhibition via imatinib and other small molecule inhibitors has been shown to be effective in preclinical models of pulmonary and dermal fibrosis [112, 113]. One of the targets of imatinib , c-Abl , is known to be a downstream signaling intermediate of TGF-β in fibroblasts [114]. Furthermore, morphologic transformation and activation of gene expression in TGF-β-stimulated fibroblasts are dependent on the activation of c-Abl, suggesting that reduction in fibrosis with imatinib treatment may partially involve inhibition of TGF-β signaling.
Another group of agents thought to have efficacy in fibrosis and nephropathy are angiotensin-converting enzyme (ACE) inhibitors , agents that are widely used to treat hypertension and heart failure. These agents act by preventing the generation of Angiotensin II which is a vasoconstrictor [115]. More recently, animal studies have shown that ACE inhibitors can ameliorate radiation-induced normal tissue injury. For example, captopril, an ACE inhibitor, reduced pulmonary endothelial dysfunction in irradiated rats [116]. Captopril and other ACE inhibitors were also shown to minimize lung fibrosis caused by radiation [117, 118]. In addition to lung injury, ACE inhibitors have been reported to protect other organs such as the kidney [119] and skin [120, 121] from radiation injury. Importantly, ACE inhibitors have been shown to reduce radiation lethality from lung and kidney toxicity after total body exposures [119]. As for the effects of ACE inhibitors on tumors , a study of ramipril in mice bearing A549 xenografts had no effect on tumor response [121]. The mechanism by which captopril mitigates radiation injury is unclear. It has been hypothesized that it maybe through inhibition of angiotensin II production as angiotensin II is known to promote the expression of TGF-β , a known pro-fibrogenic factor [122, 123].
Small molecule inhibitors account for many of the agents thought to be effective as radiation mitigators. Another area of growing interest for radiation mitigation is cell-based therapies . Cell-based therapies can be used to inhibit inflammatory processes or to repopulate the damaged organ. The infusion of mesenchymal stem cells , an expandable, multipotent stem cell found in mesenchymal tissues, has been reported to mitigate radiation fibrosis in the lung and skin [124, 125]. These cells may be derived from a number of mesenchymal tissues such as bone marrow and adipose tissues . The use of these cells as a therapeutic option is of particular interest given that they can be harvested, expanded in vitro, and stored for future use. Further, these cells are considered immune privileged due to low expression of MHC-II, allowing the use of donor-derived mesenchymal stem cells as therapy. Importantly, the timing of delivery of these cells may be important in their efficacy. Evidence from skin fibrosis models suggests that the interaction of bone marrow stromal cells with activated macrophages results in macrophage repolarization , with elaboration of anti-inflammatory IL-10 [125]. Thus, it is possible that effective treatment with bone marrow stromal cells for mitigation requires a substantial accumulation of macrophages in the irradiated tissues, which is often not seen until several weeks after treatment . Stem cell-based therapies for radiation-induced organ dysfunction have also been studied for other types of radiation injury, such as liver injury and osteonecrosis [126–128].
Central Nervous System Injury
Patients with multiple brain tumors that cannot be surgically resected are commonly treated with whole-brain radiotherapy and/or stereotactic radiosurgery . Unfortunately, patients can experience cognitive impairments from tumor progression or radiation-induced demyelination, vascular damage, and white matter necrosis [129]. Although oxidative stress and inflammation have been implicated in promoting radiation-induced brain injury [130], more recent data suggests that brain tumors themselves may produce inflammatory changes in the CNS microenvironment that are independent of radiation [131]. Accordingly, agents that inhibit these cellular processes including ACE inhibitors , peroxisome proliferator-activated receptor-у (PPARу) agonists , and vascular endothelial growth factor (VEGF) inhibitors have been evaluated in animal models, and preclinical evidence suggests that it may be effective as radiation mitigators for brain injury [130, 132–137].
Clinical trials to mitigate radiation toxicity in the CNS have largely focused on technical approaches to reduce radiation dose to critical structures or off-label use of pharmacotherapies commonly used to treat dementia. RTOG 04 utilized intensity-modulated external beam radiation planning techniques to reduce the dose of radiation delivered to the hippocampus of patients requiring whole-brain radiation for treatment of brain metastases, with the hippocampal sparing technique producing improved performance on a neurocognitive evaluation at 4 months following treatment [138]. Acetylcholinesterase inhibitors , such as donepezil , have been evaluated for differences in neurocognition, mood, and quality of life outcomes in adult and in pediatric patients with some improvement compared to baseline at 24 weeks after radiation treatment [139, 140]. NMDA receptor antagonists such as memantine have produced some improvements in cognitive function over time and delayed neurocognitive decline 24 weeks after whole-brain radiotherapy [141].
Despite the current data demonstrating short-term benefits with some radiation mitigators in the CNS, there is a severe lack of understanding about the underlying biological processes which produce acute and long-term neurodegenerative changes. Most clinical strategies have relied on the assumption that the underlying biological process of neurocognitive decline seen in other conditions such as dementia also holds true for radiation-induced CNS damage. However, this assumption may be misplaced or may not reflect the entire spectrum of radiation-induced changes. The goal of current scientific investigations is to determine how the DNA damage response from radiotherapy to the CNS compartment translates to altered structural and biochemical changes so that more effective, targeted therapies may be developed to afford patients long-term, sustained benefits after radiation.
Treatment
Agents that are given after the development of radiation-induced symptoms are characterized as treatments. In general, preventing normal tissue injury with the use of radioprotectors and mitigators is preferable since some of these toxicities are irreversible and only limited treatments of variable efficacy exist. Treatments for radiation injury may be used for a short duration, or ongoing treatment may be required for prolonged periods to maintain clinical benefit. A comprehensive discussion of treatment of radiation injury is outside the scope of this section; however a few examples are highlighted below. In addition to the examples provided, a number of treatments have been studied for gastrointestinal toxicity, dermatologic toxicity, and mucositis with varying degrees of efficacy.
Fibrosis is a particularly challenging toxicity of radiotherapy, often considered to be irreversible. One treatment that has shown efficacy in this setting is pentoxifylline combined with vitamin E. A double blind, placebo-controlled trial of pentoxifylline and vitamin E in patients with radiation fibrosis of the skin and subcutaneous tissues after treatment for breast cancer found a marked regression in fibrotic surface area with treatment [142]. These findings were confirmed in a study of patients with radiation fibrosis at multiple sites, who were found to have improved range of motion and reduced pain with pentoxifylline treatment [143]. Importantly, long-term treatment with pentoxifylline and vitamin E appears to be necessary, with the possibility of rebound effects if treatment is discontinued too early [144]. Studies of pentoxifylline have also been completed for patients suffering from radiation injury in a number of organs with variable success [145–149].
Radiation pneumonitis may occur in up to 15 % of patients treated with thoracic radiotherapy [150]. Pneumonitis is characterized by fever, cough, and dyspnea with radiographic changes corresponding to the radiated field and may occur within the first 18 months after irradiation. Radiation pneumonitis is a diagnosis of exclusion, meaning that infection, tumor spread, and other causes of lung inflammation must be excluded. Radiation pneumonitis evolves into radiation fibrosis over time, although symptomatic pneumonitis is not a prerequisite for developing radiation fibrosis. Glucocorticoids remain the most effective treatment for radiation pneumonitis but are not considered an effective treatment for radiation fibrosis [151, 152]. Oxygen is also used as needed to limit hypoxia.
In patients receiving radiotherapy to the brain or head and neck, radiation brain necrosis may occur as a side effect. Traditionally, radiation necrosis has been managed with glucocorticoids, with surgical resection reserved for patients in whom radiation necrosis causes persistent symptoms. More recently, bevacizumab, a VEGF inhibitor, has been reported to substantially ameliorate radiation-induced brain necrosis [153–156]. In a randomized trial of bevacizumab versus placebo in 14 patients with biopsy or radiographically confirmed radiation necrosis, treatment with bevacizumab improved neurologic symptoms and signs in all patients [154]. All patients treated with placebo were allowed to cross over into the bevacizumab arm. No response was seen after treatment with the placebo; however, after bevacizumab treatment , only two patients developed a recurrence of radiation necrosis .
Challenges
A number of challenges exist in the effective preclinical and clinical development of the agents for use as radioprotectors and radiation mitigators. One major concern is the ability of animal models to predict the behavior of the human condition. Animals may have different pharmacokinetic responses to drugs, which may alter the appropriate timing and dosing of agents. Conventional radiotherapy often continues daily for up to 8 weeks, a condition challenging to replicate in animal models of injury in which one to ten fractions are typically delivered. Animals also tend to be of similar genetic background with no comorbidities that may affect drug metabolism, drug penetration, and susceptibility to radiation toxicity. And it cannot be forgotten that mice have fundamental biological differences from humans.
Another factor complicating the study of these agents is the use of chemotherapy concurrently with radiotherapy in a growing number of cancers. As a result, testing of these agents as radioprotectors or mitigators often requires assessment of effect in the context of combined radiation and chemotherapy, not just radiotherapy alone. This increases the level of complexity of preclinical studies, increases cost substantially, and complicates analysis of efficacy. To ensure that candidate radiation protectors or mitigators do not impair tumor control, additional studies should always be completed in tumor models before clinical translation if it is expected that they will be delivered in close proximity to radiotherapy.
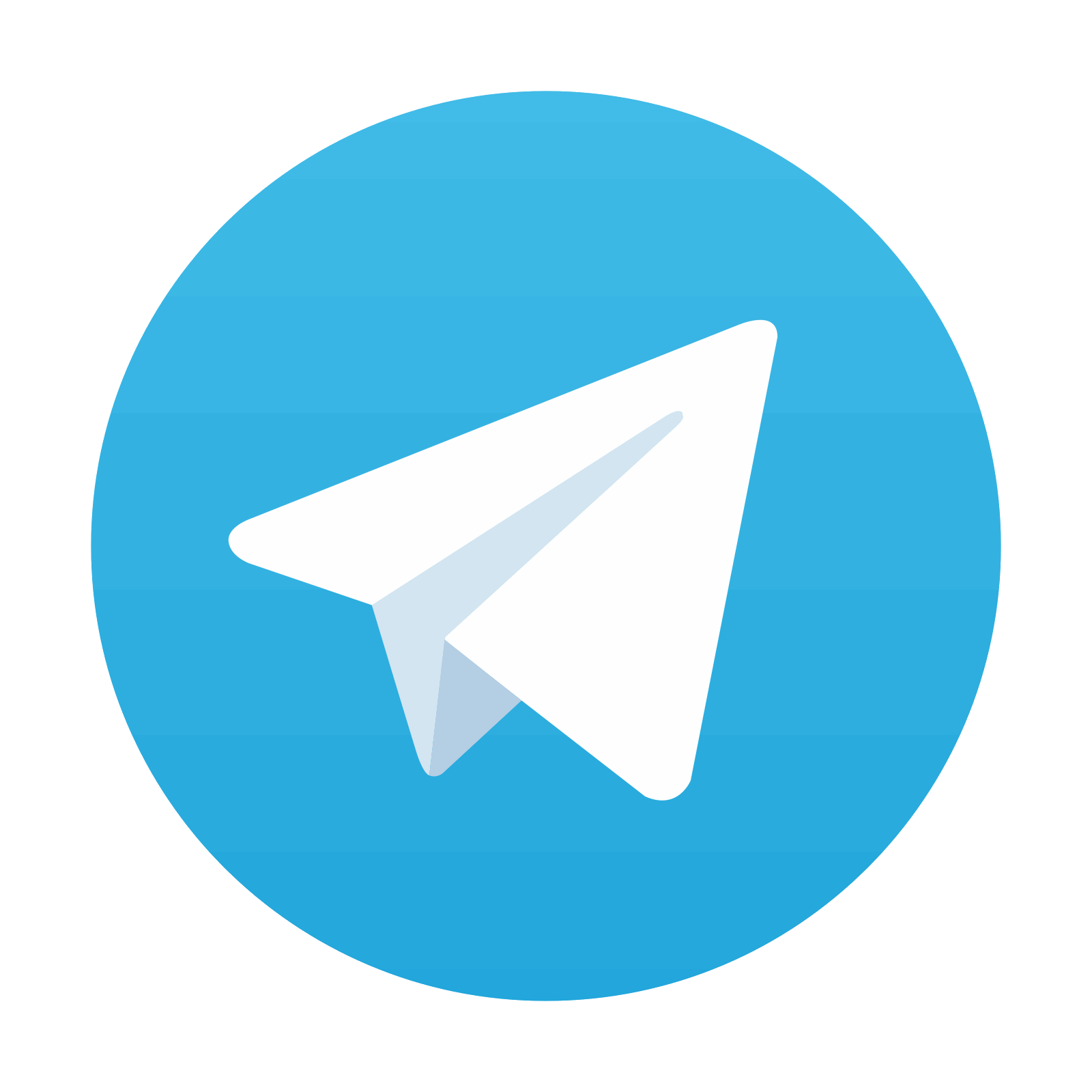
Stay updated, free articles. Join our Telegram channel

Full access? Get Clinical Tree
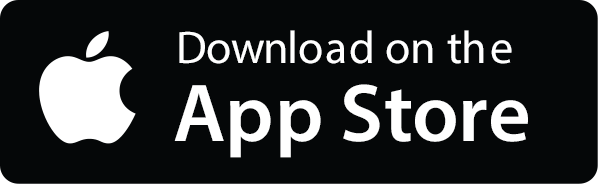
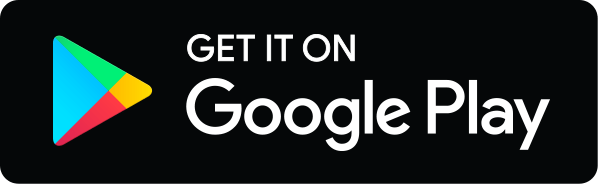