Luca Saba, João Miguel Sanches, Luís Mendes Pedro and Jasjit S. Suri (eds.)Multi-Modality Atherosclerosis Imaging and Diagnosis201410.1007/978-1-4614-7425-8_20© Springer Science+Business Media, LLC 2014
20. Relationship Between Plaque Echogenicity and Atherosclerosis Biomarkers
John D. Kakisis1 , Efthimios Avgerinos1, Nikolaos Kadoglou1, George Sfyroeras1, Konstantinos Moulakakis1 and Christos D. Liapis1
(1)
Department of Vascular Surgery, Athens University Medical School, “Attikon” Hospital, Rimini 1, 12462 Athens, Haidari, Greece
Abstract
An interesting link between biomarkers and carotid plaque echogenicity has been studied over the past few years, with biomarkers representing a part of the pathophysiologic process that leads to the development of a plaque with the respective morphological characteristics. The role of cytokines, vascular calcification markers, HbA1c, MMPs, soluble CD36 and CD40 ligand, RBP 4, OxLDL, and CRP is reviewed.
1 Introduction
Prediction of the outcome of carotid stenosis is currently based on two main factors: the history of neurologic events and the degree of stenosis. However, even for the highest risk group of recently symptomatic patients with severe carotid stenosis, the majority of the patients will remain asymptomatic, indicating that our ability to predict future strokes needs further improvement. Several imaging modalities have been used for the identification of the vulnerable carotid plaque, including duplex ultrasound, computed tomography, magnetic resonance imaging, fluorine-18-labeled fluorodeoxyglucose positron emission tomography, optical coherence tomography and laser-induced fluorescence spectroscopy [1]. The most widely used of all these techniques is duplex ultrasound, with the prognostic significance of plaque echolucency having been demonstrated in more than 8,000 patients [2–6]. The underlying mechanism is plaque rupture, with subsequent embolization to the brain, which is more likely in a lipid-rich, fragile, echolucent plaque.
Advances in cellular and molecular pathophysiology and the demand to accurately predict stroke risk and choose the optimal prevention strategy (carotid endarterectomy, carotid stenting, or medical treatment) have stimulated great interest in the development of novel diagnostic markers [7]. Serum biomarkers have emerged as potential predictors of carotid artery disease natural history and perioperative risk, while recent data suggest that they may guide carotid intervention and bio-oriented therapies [8].
Serum biomarkers are molecules produced and secreted by several cells that are present in the atherosclerotic plaque such as monocyte-derived macrophages, T-lymphocytes, activated endothelial cells, and proliferating smooth muscle cells. Such molecules include C-reactive protein (CRP), matrix metalloproteinases and their inhibitors, soluble CD40 ligand, cytokines, oxidized LDL, lipoprotein-associated phospholipase A2, type II secretory phospholipase A2, myeloperoxidase, monocyte chemoattractant protein-1, etc. [1, 9, 10]. These molecules induce a local inflammatory response, activation and proliferation of smooth muscle cells, lesion progression, and matrix degradation of the fibrous cap [9]. In this context, there is an interesting link between biomarkers and carotid plaque echogenicity, with biomarkers representing a part of the pathophysiologic process that leads to the development of a plaque with the respective morphological characteristics.
2 Cytokines
Serum IL-6 levels have been found to be significantly higher in patients with symptomatic compared with those with asymptomatic carotid disease. Symptomatic patients have also more intense macrophage infiltration of the atherosclerotic plaque suggesting that inflammatory process may contribute to the destabilization of the carotid plaque [11]. Intracellular cytokine expression in peripheral blood has also been found to be significantly higher in patients undergoing carotid endarterectomy who had previous stroke (IFN-g, IL-1b, IL-6, IL-8, IL-4, and IL-10) or amaurosis fugax (IFN-g, IL-6, IL-4, and IL-10) than in patients not undergoing endarterectomy [12].
The theory of the link between cytokine expression and plaque vulnerability has been verified by an ultrasonographic study demonstrating that elevated serum IL-6 levels are associated with lower echogenicity of carotid plaques as quantified by integrated backscatter analysis [13]. The association remained significant when traditional atherosclerotic risk factors, plaque thickness, and medication use were controlled for.
IL-6 is also released from carotid stenotic lesions after stenting, with the level of local IL-6 release being higher in plaques with lower echogenicity and less plaque calcification [14]. Prominent secretion of IL-6 from symptomatic lesions and lower echogenic lesions support the close association of plaque instability and IL-6 production in vulnerable plaques. IL-6 release after carotid stenting has also been found to be associated with periprocedural new ischemic lesions [15]. On the other hand, carotid plaques retrieved at endarterectomy from patients taking statins showed significantly reduced concentrations of IL-6, suggesting a lesser degree of localized inflammation within the plaques of patients on statin therapy [16].
Although the association between IL-6 and plaque vulnerability has been proven, it is not clear whether IL-6 is the cause or the effect of this vulnerability. High levels of IL-6 in peripheral blood may reflect the increased production of IL-6 by inflammatory cells in unstable plaques and, thus, be a simple marker of increased inflammatory activity within the plaque [17]. On the other hand, IL-6 may itself facilitate the formation of unstable plaques through:
Activation of mononuclear cells to secrete monocyte chemotactic protein 1 (MCP-1), a chemokine pivotal for monocyte recruitment [18]
Stimulation of the synthesis of all the acute phase proteins involved in the inflammatory response (CRP, serum amyloid A, fibrinogen, α1-chymotrypsin, and haptoglobin) [19, 20]
3 Vascular Calcification Markers
Atherosclerotic plaque calcification enhances plaque stability and decreases the likelihood of clinical events. A growing number of stimulatory and inhibitory molecules suggest that vascular calcification is an actively regulated process. Among these molecules, osteopontin (OPN), an acidic phosphoprotein, and osteoprotegerin (OPG), a member of the TNF-a receptor superfamily, have recently been demonstrated to inhibit mineral deposition as well as osteoclastogenesis, and they are constitutively expressed by a wide range of cell types in vasculature [23]. Although the large prospective study by Nybo et al. found no association of baseline OPG with ischemic stroke [24], a recent comparative study (symptomatic vs. asymptomatic patients with carotid stenosis) showed a strong relationship of serum OPN and OPG levels with low carotid plaque echogenicity and symptomatology [25].
These findings support the notion that OPN downregulates plaque calcification and may promote plaque instability [25]. Aside from direct effects, OPN may facilitate plaque destabilization indirectly. Available data suggest that OPN may induce matrix metalloproteinases release and angiogenesis within the atherosclerotic plaque leading to fibrous cap degradation and hemorrhage, respectively [26–28]. Taken all together, OPN seems to assume a key role in atherogenesis and cerebral ischemic events occurrence, but the underlying mechanisms require further research.
OPG comprises a crucial inhibitor of vascular calcification. OPG-deficient mice exhibit severe osteoporosis and vascular calcification of the aorta and renal arteries [29]. This phenotype can be prevented by delivery of the recombinant OPG and transgenic overexpression of OPG [30]. Consequently, the elevated OPG levels in symptomatic patients and their independent relationship with GSM suggest less calcification and a contributory role of OPG to plaque vulnerability [25]. Probably targeting OPG may provide a novel therapeutic strategy against carotid plaque rupture.
4 Glycated Hemoglobin
HbA1c is an indicator of average glucose levels over the previous 6–8 weeks. Apart from its common use as an indicator of intermediate-term glycemic control, HbA1c can also be used as a marker of increased mortality and cardiovascular disease in both diabetic and nondiabetic persons [31–36].
With regard to carotid disease, increasing levels of HbA1c are associated with both IMT [37–39] and carotid plaque prevalence [40]. In the Tromsø study, HbA1c level was found to be strongly related to the prevalence of echogenic and predominantly echogenic plaques but not, or if anything negatively, associated with the risk of echolucent plaques in nondiabetic individuals [41]. The authors postulate that the high prevalence of echogenic plaques may reflect the fact that subjects with high HbA1c levels have had plaques for a longer period than euglycemic persons at the same age, or that the progression from soft to hard plaque is accelerated in hyperglycemic subjects [41]. Another possible mechanism is that activation of transforming growth factor-β occurs at an early stage of diabetes mellitus triggering fibrotic changes in the tissue and, thus, leading to a high content of fibrous tissue within the developing plaque [41, 42].
5 Matrix Metalloproteinases
Matrix metalloproteinases are an ever-expanding family of zinc-dependent endopeptidases with proteolytic activity toward one or more components of the extracellular matrix [43]. Growing evidence supports the strong relationship of MMPs with plaque instability and consequent cardiovascular events. Insights from human pathological studies and experimental models of atherosclerosis have revealed the overproduction of MMPs by inflammatory cells in the rupture-prone regions of atherosclerotic plaques [44, 45].
In a follow-up study, the MMP-9 plasma concentrations predicted stroke and cardiovascular death in patients with ≥50% carotid stenosis, although not independently [45]. These findings were further confirmed by two nonprospective studies comparing symptomatic and asymptomatic patients [46, 47]. The positive predictive value of MMP-9 was significantly enhanced when combined with other members of the MMP family (MMP-7 and MMP-8 and their tissue inhibitor of metalloproteinases-1) [47] or with plaque echolucency [27].
Pregnancy-associated plasma protein A (PAPP-A) is a high-molecular-weight metalloproteinase that is typically measured during pregnancy in maternal blood for the fetal diagnosis of Down syndrome [48]. However, circulating PAPP-A is physiologically present in both men and women; moreover, it is abundantly expressed in plaque cells and extracellular matrix of ruptured and eroded unstable coronary plaques, but not in stable plaques [49]. In patients with carotid artery disease, circulating levels of the metalloproteinase PAPP-A were found to be significantly higher in those who exhibited hyperechoic or isoechoic, echogenic plaques compared with patients with hypoechoic plaques [48]. The significant positive correlation observed between CRP and PAPP-A levels supports the notion that PAPP-A is produced by activated cells and released into the extracellular matrix during the local inflammatory process occurring within the arterial wall [48].
6 Soluble CD36
CD34 is a surface glycophosphoprotein expressed on lymphohematopoietic stem and progenitor cells, small-vessel endothelial and embryonic fibroblasts [50]. It has been shown to be involved in angiogenesis, inflammation, lipid metabolism, platelet activation, and atherosclerosis. CD36 is present in lipid-laden macrophages in atherosclerotic lesions [51–53], whereas CD36-apo E double-null animals have been found to be markedly resistant to lesion formation even in areas of enhanced predisposition [54]. These findings underscore the essential role of CD34 in the atherogenic process.
The association between CD36 and advanced atherosclerosis is also supported by the finding of strong immunostaining of CD36 in symptomatic as compared with asymptomatic carotid plaques, primarily located to lipid-loaded macrophages in the fatty core of the atherosclerotic plaque [55]. Plasma levels of soluble CD36 were markedly elevated in those with recently symptomatic carotid plaques, within the last 2 months, as compared with other patients. Furthermore, patients with echolucent carotid plaques tended to have higher soluble CD36 levels in plasma compared with those with echogenic/heterogenic plaque [55]. It is speculated that apoptosis of lipid-loaded macrophages may lead to enhanced release of CD36. In this context, soluble CD36 can be used as a marker of plaque echolucency and destabilization.
7 Soluble CD40 Ligand
CD40 ligand (CD40L) is a transmembrane protein and a member of the TNF superfamily. It is expressed by all major cells implicated in inflammation and atherosclerosis, including activated T-lymphocytes, vascular endothelial cells, smooth muscle cells, monocytes/macrophages, and platelets [56, 57]. Ligation of CD40 enhances the expression of proinflammatory and atherogenic molecules such as cytokines, chemokines, matrix metalloproteinases, growth factors, and adhesion molecules, as well as prothrombotic mediators such as tissue factor [10].
In a prospective, nested case–control evaluation of healthy middle-aged women, mean concentrations of soluble CD40L at baseline were significantly higher among the participants who subsequently developed myocardial infarction, stroke, or cardiovascular death, compared with the age- and smoking-matched women who remained free of cardiovascular disease during a 4-year follow-up [58]. These findings, however, were not verified by a recent study, in an older (aged 60–69 years) general population cohort [59].
In an MRI study of carotid plaques in patients with carotid atherosclerosis on ultrasonography, baseline levels of soluble CD40L were higher among patients with evidence of intraplaque lipid than among those without it [60]. In contrast, soluble CD40L levels were not correlated with percent diameter stenosis. The relative risk for intraplaque lipid associated with soluble CD40L levels above the median was 6.0, with the magnitude of this predictive effect remaining practically the same after adjustment for traditional cardiovascular risk factors.
There are several ways by which soluble CD40L may participate in the destabilization of atheromatous plaques. CD40L induces apoptosis and the formation of necrotic core in atheromatous plaques [61]. In addition, CD40L stimulates endothelial cell proliferation and promotes in vivo angiogenesis, thus representing a major determinant of intraplaque neovascularization and plaque vulnerability [62].
In mice, inhibition of CD40 signaling reduced the size of aortic atherosclerotic lesions by 59% and their lipid content by 79% [63]. Furthermore, atheroma of mice treated with anti-CD40L antibody contained significantly fewer macrophages and T lymphocytes, more smooth muscle cells and collagen and exhibited decreased expression of vascular cell adhesion molecule-1 [63, 64]. Similarly, in an animal model of progressive atherosclerosis, a pronounced increase in collagen content, vascular smooth muscle cell/myofibroblast content, and fibrous cap thickness was observed, while the lipid core and macrophage content decreased [65].
8 Retinol-Binding Protein 4
Retinol-binding protein 4 (RBP4) is a recently identified adipokine suggested to link obesity with its comorbidities, especially insulin resistance, type 2 diabetes, and certain components of the metabolic syndrome [66]. RBP4 may also elicit subclinical inflammation leading to cardiovascular disease [67]. A strong correlation between RBP4 and inflammation markers, such as CRP, monocyte chemoattractant protein-1, and CD68, has been found [67, 68]. Studies in patients with type 2 diabetes or coronary artery disease have also revealed an association between RBP4 levels and markers of lipid metabolism, such as total cholesterol, LDL-cholesterol, VLDL cholesterol, triacylglycerol levels, and hepatic lipase activity [69].
The lipid-modulating activities of RBP4 may explain the finding that circulating RBP4 concentrations are inversely associated with intima media and plaque echogenicity in carotid arteries [70]. The higher fat content in the vessel wall and in atherosclerotic plaques of subjects with higher RBP4 levels implies that RBP4 could be involved not only in the development of atherosclerosis but also in the development of vulnerable atherosclerotic plaques as well. Interestingly, in the Uppsala Longitudinal Study of Adult Men, which recruited among all 50-year-old men living in Uppsala in 1970–1974, RBP4 concentrations were significantly associated with prior cerebrovascular disease and any prior hospitalization for cardiovascular disease in age-adjusted analyses, whereas no significant associations were found between RBP4 concentrations and prior myocardial infarction [71].
9 Oxidized LDL
LDL cholesterol is one of the major risk factors for atherosclerosis, since it is causally associated with the development of atherosclerosis, whereas a reduction in LDL levels leads to a reduction of atherosclerosis progression or even regression of atherosclerosis, lower incidence of cardiovascular events (myocardial infarction and stroke), and even reduced total mortality [72–74]. Plasma LDL is transported across the endothelium to the subendothelial space where it is subjected to oxidative modifications to produce highly oxidized LDL (OxLDL). OxLDL is a potent inducer of inflammatory molecules and is believed to be the most atherogenic form of LDL.
In accordance with this notion, the plasma OxLDL level has been found to be significantly higher in patients with carotid stenosis than in controls, suggesting that OxLDL is involved in carotid artery disease, as is the case in coronary artery disease [75]. The plaque OxLDL level was nearly 70 times higher than plasma OxLDL. There was also a strong correlation between the plaque OxLDL level and the extent of macrophage infiltration. The macrophage-rich plaques were associated with plaque rupture, fibrous cap thinning, and a large-sized lipid core and correlated with ultrasonographic echolucency but not with angiographical carotid stenosis [75].
Similarly, in a cross-sectional population-based study of 61-year-old men, plasma OxLDL turned out to be independently associated with the occurrence of echolucent plaques both in the femoral and the carotid artery [76, 77]. The source of OxLDL in plasma could be the direct release of OxLDL from ruptured or permeable plaques, ischemic injury to damaged cell membranes, or a turnover of OxLDL in newly formed or progressing lesions in the arterial tree [76].
10 C-Reactive Protein
CRP is currently the most studied biomarker used in clinical practice. In several prospective studies, baseline CRP has been related to the risk of cerebrovascular events among healthy adults, patients with asymptomatic carotid lesions, and other patient cohorts [78–85]. A meta-analysis of studies with a long-term follow-up showed that the risk of stroke in healthy individuals with the highest quartile of CRP concentrations increased by 70% compared with those in the lowest quartile [86]. Nonprospective studies comparing symptomatic vs. asymptomatic patients with carotid stenosis advocate that CRP may identify carotid plaque at risk of symptomatic conversion [87, 88].
Despite these findings that support an association between CRP levels and plaque instability, there have not been any data, so far, correlating CRP with plaque echogenicity. CRP has been found to be associated with total carotid plaque area among men in models controlling for traditional atherosclerotic risk factors, but did not discriminate echolucent from echogenic carotid plaques in neither women nor men [89]. In another study, high-sensitivity CRP concentrations were negatively correlated with carotid plaque echogenicity in univariate analysis, but when traditional atherosclerotic risk factors, plaque thickness, and medication use were controlled for, the association was of borderline significance [13]. Other reports have further supported the notion that carotid plaque echolucency is not related to systemic levels of CRP [90, 91].
References
1.
2.
Nicolaides AN, Kakkos SK, Griffin M, Sabetai M, Dhanjil S, Thomas DJ et al (2005) Asymptomatic Carotid Stenosis and Risk of Stroke (ACSRS) Study Group. Effect of image normalization on carotid plaque classification and the risk of ipsilateral hemispheric ischemic events: results from the asymptomatic carotid stenosis and risk of stroke study. Vascular 13:211–221PubMed
3.
4.
Mathiesen EB, Bonaa KH, Joakimsen O (2001) Echolucent plaques are associated with high risk of ischemic cerebrovascular events in carotid stenosis: the tromso study. Circulation 103:2171–2175PubMedCrossRef
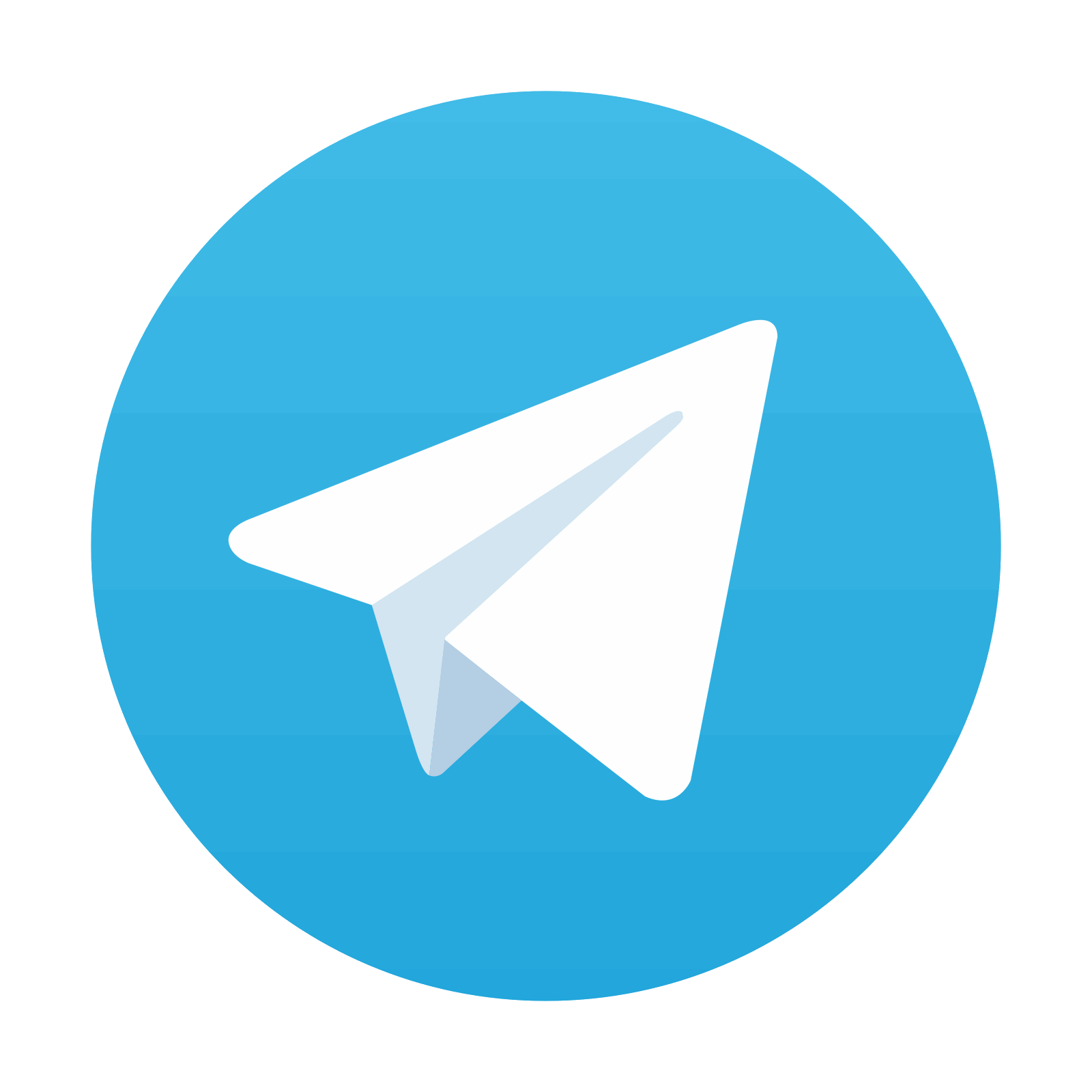
Stay updated, free articles. Join our Telegram channel

Full access? Get Clinical Tree
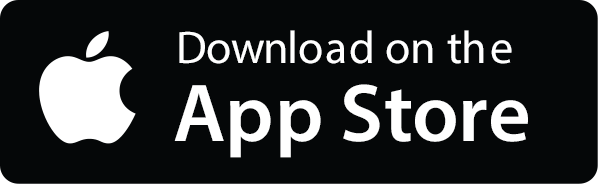
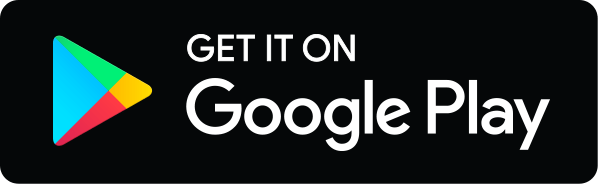
