Fig. 1.1
Role of LDL, monocyte, macrophage, and chemokines for atherogenesis. ICAM intercellular adhesion molecule, LDL low-density lipoprotein, MCP monocyte chemoattractant protein, MPO myeloperoxidase, sPLA2 secretory phospholipase A2, TNF tumor necrosis factor, VCAM vascular cell adhesion molecule
The recruitment of monocytes and lymphocytes to the artery wall also contribute to the atherogenesis [11]. A triggering event for this process is the accumulation of minimally oxidized LDL, which stimulates the overlying endothelial cells to produce a number of pro-inflammatory molecules, including adhesion molecules and growth factors [12]. Oxidized LDL can also inhibit the production of nitric oxide, a chemical mediator with multiple anti-atherogenic properties including vasorelaxation [13]. The entry of specific types of leukocytes into the artery wall is mediated by adhesion molecules and chemotactic factors including P- and E-selectin, intercellular adhesion molecule 1 and vascular cell adhesion molecule 1 [14, 15].
Through reactive oxygen species (ROS) and enzymes including myeloperoxidase (MPO), sphingomyelinase, and secretory phospholipase [16, 17], LDL is extensively modified in atherosclerotic lesions. The rapid uptake of highly oxidized LDL particles by macrophages leads to foam-cell formation which is derived by a group of receptors such as scavenger receptors, scavenger receptor A and CD36 [18, 19]. The expression of scavenger receptors is regulated by peroxisome proliferator-activated receptor-γ, a transcription factor whose ligands include oxidized fatty acids, and by cytokines such as tumor necrosis factor-α and interferon-γ [20, 21].
Any process that decreases the synthesis of fibrous cap collagen by intimal fibromyoblast-like smooth muscle cells (SMCs) and/or contributes to cap collagen degradation would be expected to promote the formation of plaques prone to rupture [22]. Vulnerable plaques show evidence of SMC death and decreased numbers of SMCs, and in vitro data show that macrophages can trigger apoptosis in SMCs by activating their Fas apoptotic pathway and by secreting proapoptotic tumor necrosis factor-α and nitric oxide [23, 24]. Macrophages may also decrease collagen synthesis in intimal SMCs without actually killing the cells. Macrophage-derived matrix metalloproteinases (MMP), which are a family of protease-activated enzymes, can degrade various types of extracellular matrix proteins and may also be involved in thinning of the fibrous cap [25, 26].
Residual Risk Factors: Potential New Targets
Over the course of the last two decades, large randomized controlled trials have consistently demonstrated that lowering levels of LDL cholesterol reduce cardiovascular event rates across the full spectrum of risk [27–30]. As a result, LDL-C lowering has become an integral component of therapeutic strategies in the prevention of cardiovascular diseases. However, despite statins therapy, substantial amount of cardiovascular events is still observed (Table 1.1). The Scandinavian Simvastatin Survival Study (4S) reported a 20 % cardiovascular events occurring in statin-treated patients although statin treatment was associated with a significant reduction of cardiovascular events compared to placebo [27]. The presence of residual cardiovascular risk was similarly observed in other major trials of statin therapy, which prompted the focus on optimal statin use [31, 32]. Even in three landmark trials focusing on the effect of high-dose statins, residual cardiovascular risk was still apparent in the intensive statin arms [33–35]. Thus, analyses of clinical trial data indicate the need to retool our cardiovascular risk reduction algorithms beyond the focus on LDL cholesterol levels.
Table 1.1
Residual risks observed in clinical trials under statins therapy
Trials | Subjects | Therapy | Follow-up period (years) | Outcome after therapy | Annual residual risks a |
---|---|---|---|---|---|
Statin vs. placebo | |||||
4S [27] | 4,444 patients with CAD or previous MI | Simvastatin vs. placebo | 5.4 | 33 % reduction of CV events | 3.5 % |
AFCAPS/TexCAPS [28] | 6,605 patients without CAD | Lovastatin 20–40 mg vs. placebo | 5.2 | 25 % reduction in CV events (MI, cardiac cause death, revascularization, unstable angina) | 2.2 % |
CARE trial [29] | 4,159 patients with MI | Pravastatin 40 mg vs. placebo | 5 | 24 % reduction in CV events (MI, cardiac-cause death) | 1.5 % |
CARDS trial [30] | 2,838 diabetic patients without CAD | Atrovastatin 10 mg vs. placebo | 3.9 | 37 % reduction in CV events (MI, cardiac-cause death, revascularization, arrest, unstable angina, stroke) | 1.5 % |
LIPID Study [31] | 9,014 patients with CAD | Pravastatin 40 mg vs. placebo | 6.1 | 29 % reduction in CV events (MI, cardiac-cause death, revascularization, stroke) | 2 % |
PROSPER trial [32] | 5,804 elderly patients with cardiovascular risk factors | Pravastatin 40 mg vs. placebo | 3.2 | 13 % reduction in CV events (MI, cardiac-cause death, stroke) | 4.4 % |
High-dose statin | |||||
PROVE IT-TIMI 22 trial [33] | 4,162 patients with ACS | Pravastatin 40 mg vs. atorvastatin 80 mg | 2 | 16 % reduction in CV events (MI, any-cause death, revascularization, unstable angina, stroke) | 11.2 % (atrovastatin) 13.2 % (pravastatin) |
IDEAL study [34] | 8,888 patients with MI | Atrovastatin 80 mg vs. simvastatin 20 mg | 4.8 | 12 % reduction in CV events (MI, cardiac-cause death, revascularization, arrest, unstable angina, stroke) | 2.5 % (atrovastatin) 2.9 % (simvastatin) |
TNT study [35] | 10,001 patients without CAD | Atrovastatin 10 mg vs. 80 mg | 4.9 | 22 % reduction in CV events (MI, cardiac-cause death, arrest, stroke) | 1.8 % (80 mg) 2.2 % (10 mg) |
Atherogenic Lipoproteins
The deposition of atherogenic lipoproteins in the vessel wall is the major driver for atherosclerosis (Table 1.2). In the recent years, there has been increased recognition of the relationship between cardiovascular risk and other lipid parameters, most notably high-density lipoprotein (HDL) cholesterol and triglycerides (TG).
Table 1.2
Lipid markers for the Prediction of Residual Risks
Study population | Outcome | Follow-up periods (years) | Findings | |
---|---|---|---|---|
HDL-C | ||||
Gordon et al. [36] | 15,252 patients without CAD | CAD | 2 | Each 1 mg/dL increase in HDL-C is associated with a 2 % decrease in CAD risk in men and a 3 % decrease in women |
Castelli et al. [37] | 1,605 patients without CAD | CHD | 12 | HDL-C <40 mg/dL exhibits the highest risk of developing CHD |
Barter et al. [38] | 9,770 patients with CAD | CHD | 5 | Patients with HDL-C <38 mg/dL had a highest incidence of CHD despite low LDL-C level |
Jafri et al. [39] | 543,210 patients | MI | 3.9 | 10 mg/dL increase in HDL-C was associated with higher incidence of MI under statin therapy |
TG | ||||
Sarwar et al. [41] | 9,757 men with CHD | CHD | 12 | Odds ratio: 1.7 (1.5–1.9) for CHD under an increase in triglyceride |
Miller et al. [42] | 4,162 patients with ACS | CHD | 2 | 10 mg/dL decrease in triglyceride reduces the incidence of CHD by 1.6 % under statin therapy |
Assmann et al. [43] | 4,849 men without CAD | CHD | 8 | Patients with TG <200 mg/dL exhibited the lowest incidence of CAD |
Non-HDL-C | ||||
Cui et al. [43] | 4,462 patients without CHD | CHD | 19 | Non-HDL-C >220 mg/dL was associated with high mortality |
Boekholdt et al. [44] | 38,153 patients | CAD | 4.6 | The adjusted HR for CVD per 1-SD increase was 1.16 (1.12–1.19) for non-HDL-C. This HR was significantly higher than LDL-C and apoB |
Liu et al. [45] | 19,381 patients without CAD | CAD | 13 | Each increase by 1 mg/dL of non-HDL cholesterol was associated with a 5 % increased risk for cardiac-cause death |
Lp (a) | ||||
Danesh et al. [50] | 5,436 patients with CHD | CAD | 10 | The adjusted HR for CHD was 1.7 (1.4–1.9) for Lp (a) |
The Emerging Risk Factors Collaboration [51] | 126,634 patients without CHD | CHD | 10.2 | The adjusted risk ratio for CHD was 1.2 (1.1–1.2) for Lp (a) |
Nicholls et al. [52] | 2,769 patients with CAD | CHD | 3 | Lp(a) ≥ 30 mg/dL was associated with a greater rate of MACE in patients with LDL-C > 70 mg/dL |
Oxidized LDL | ||||
Melsinger et al. [55] | 346 patients without CAD | CVD | 5.6 | Plasma oxLDL was the strongest predictor of CHD events compared with a conventional lipoprotein profile and other traditional risk factors for CHD |
Shimada et al. [56] | 246 patients with CVD | CVD | 3 | The adjusted hazard ratios for CVD were 3.15 (95 % CI 1.47–6.76, P = 0.003) in patients with the highest quartile of oxLDL levels than in thosewithin the lowest quartile |
HDL Cholesterol
Epidemiological studies have shown that, in addition to elevated LDL cholesterol levels, low levels of HDL cholesterol are an independent predictor of the risk of coronary heart disease [36, 37]. Furthermore, the ability of a low level of HDL cholesterol to predict an adverse clinical risk persists in patients who achieve very low levels of LDL cholesterol on statin therapy [38, 39]. As HDL contains the potential atheroprotective properties such cholesterol mobilization, promotion of nitric oxide bioavailability, and inhibition of inflammatory activity [40], lower level of HDL has been considered to induce and progress atherosclerosis.
Triglycerides
Numerous studies have demonstrated an association between high TG level and cardiovascular risks [41–43]. The meta-analyses of data in 29 prospective studies have demonstrated that the TG level is a strong and independent predictor of cardiovascular events [41]. In addition, the PRavastatin Or atorVastatin Evaluation and Infection Therapy-Thrombolysis In Myocardial Infarction (PROVE IT-TIMI) 22 trial revealed that TG levels, independent of LDL cholesterol levels, had a substantial impact on the cardiovascular outcomes in patients with acute coronary syndromes [42]. Notably, among statin-treated patients, on-treatment TG level <150 mg/dL was associated with reduced cardiovascular risk. These findings underscore the optimal control of TG level for the prevention of future cardiac events.
Non-HDL Cholesterol
Non-HDL cholesterol has been shown to predict cardiovascular events as well as correlate with most lipid parameters associated with future outcomes [43, 44]. Evidence for the association between non-HDL cholesterol and cardiovascular risk has also come from epidemiologic data reported by Liu et al. [45]. In their analyses of the Framingham study cohort, a strong association between non-HDL cholesterol and cardiovascular risk was noted within all strata of LDL cholesterol values. In this study, non-HDL cholesterol was apparently a stronger predictor of cardiovascular events than LDL cholesterol, and this finding was independent of whether the TG level was <200 or >200 mg/dL.
Lipoprotein (a)
Lp (a) is a unique lipoprotein particle consisting of a moiety identical to LDL to which the glycoprotein apolipoprotein (a) that is homologous to plasminogen is covalently attached [46, 47]. Lp (a) has been considered to promote atherosclerosis through an increased Lp (a)-associated cholesterol entrapment in the arterial intima, inflammatory cell recruitment, carrying of proinflammatory oxidized phospholipids, impairing fibrinolysis by inhibition of plasminogen activation, and enhancing coagulation by inhibition of the tissue factor pathway inhibitor [48, 49]. Evidence from epidemiological studies continues to accumulate that elevated plasma concentrations of Lp (a) are a risk factor for a variety of atherosclerotic and thrombotic disorders. Recent data from genetic and epidemiological studies strongly support a causal relationship between elevated Lp (a) concentrations and the development of atherosclerosis and cardiovascular disease [50–52]. This relationship is independent of LDL cholesterol and HDL cholesterol levels.
Oxidized LDL
Oxidized LDL is generated during lipid peroxidation when oxygen-free radicals abstract a hydrogen atom from a methylene carbon on polyunsaturated fatty acids. It results in generation of reactive species that modify both the lipid and protein components of LDL [53, 54]. Oxidation of LDL occurs primarily in the vessel wall, activating many inflammatory and atherogenic pathways. The data from two population-based MONICA/KORA Augsburg surveys showed that plasma-oxidized LDL was the strongest predictor of cardiovascular events compared with a conventional lipoprotein profile, and other traditional risk factors [55]. Similar result was observed in another study of patients with CAD [56].
Biomarkers for the Prediction of Residual Risks
The need to improve the risk prediction has prompted the search for novel markers of cardiovascular risk (Table 1.3). The clinical utility of these biomarkers will depend on their ability to provide a reflection of the underlying atherosclerotic burden or activity and the ability to predict future events. Several emerging plasma biomarkers may ultimately prove useful in risk stratification and prognosis of cardiovascular disease.
Table 1.3
Nontraditional biomarkers for the prediction of residual risks
Biomarkers | Study population | Outcome | Follow-up periods | Risk estimates (95 % CI) |
---|---|---|---|---|
hs-CRP | ||||
Ridker et al. [57] | 27,939 women without CAD | CVD | 8 years | 2.3 (1.6–3.4) |
Haverkate et al. [58] | 2,121 patients with angina pectoris | CVD | 2 years | 1.4 (1.1–1.8) |
Rutter et al. [59] | 3,037 patients without CAD | CVD | 7 years | 1.9 (1.2–2.9) |
Ridker et al. [60] | 3,745 patients with acute coronary syndrome | MI or death | 2 years | 1.7 (1.1–2.5) |
Lp-PLA2 | ||||
Koenig et al. [66] | 934 men without CAD | CHD | 14 years | 1.2 (1.0–1.5) |
Oei et al. [67] | 2,199 elderly patients without CAD | CHD | 10 years | 1.8 (1.1–2.9) |
sPLA2 | ||||
Mallat et al. [74] | 25,663 patients with CAD | CAD | 6 years | 1.6 (1.2–2.0) |
Kugiyama et al. [75] | 142 patients with CAD | CAD | 2 years | 3.5 (1.4–8.3) |
MPO | ||||
Baldus et al. [79] | 1,090 patients with ACS | CAD | 6 months | 2.1 (1.2–3.7) |
Meuwese et al. [80] | 1,138 patients without CAD | CAD | 8 years | 1.4 (1.1-1.7) |
Tang et al. [81] | 1,895 patients with suspected CAD | CVD | 3 years | 1.7 (1.3–2.3) |
High-Sensitivity C-Reactive Protein
High-sensitivity c-reactive protein (hs-CRP) provides a stable plasma biomarker for low-grade systemic inflammation. Recent evidence suggests that hs-CRP may have direct proinflammatory effects, and contribute to the initiation, and progression of atherosclerotic lesions. Epidemiological studies have provided strong evidence for CRP to predict future cardiovascular risks in a variety of clinical settings [57–59]. Moreover, recent studies reported that an elevated hs-CRP level is associated with clinical events under optimal LDL-C control [60]. Thus, to date, of all biomarkers investigated in cardiovascular disease, the most extensive and robust database exists for hs-CRP. Still, its incremental predictive value above and beyond traditional risk factors, based on any of the available scores has not been definitely proven.
Lipoprotein-Associated Phospholipase A2
Lipoprotein-associated phospholipase represents another emerging biomarker for atherosclerotic disease. Lipoprotein-associated phospholipase A2 (Lp-PLA2) hydrolyzes sn-2 side chains of oxidized phospholipids to yield a free oxidized fatty acid and lysophosphatidylcholine [61]. It is produced mainly by monocytes, macrophages, T-lymphocytes, and mast cells and has been found to be upregulated in atherosclerotic lesions, especially in complex plaque, as well as in thin cap coronary lesions prone to rupture [62]. The weight of evidence suggests that this biomarker promotes vascular inflammation [63–65]. In recent studies of dyslipidemic patients, healthy people and patients with stroke, elevated baseline Lp-PLA2 levels were found to be associated with death, myocardial infarction and revascularization, independent of a variety of potential confounders [66–68]. Thus, measurement of Lp-PLA2 may have a potential to identify people at increased risk for cardiovascular disease. However, the sub-analysis of PROVE IT-TIMI 22 trial showed that measurement of Lp-PLA2 in the early phase of the acute coronary syndrome was not associated with increased risk for recurrent events [68]. Currently, no clear recommendation on its clinical usefulness can be given until further data document its incremental value in addition to traditional risk factors.
Secretory Phospholipase A2
Secretory phospholipase A2 (sPLA2) is another well-studied member of the phospholipase 2 family and is widely expressed in hepatocytes, macrophages, platelets, and vascular SMCs. It has been found to be involved in a range of pathways related to the development of atherosclerosis and cardiovascular disease [69]. The action of sPLA2 on LDL results in smaller particles that are more susceptible to oxidative modification and to uptake by macrophages, the seminal events in foam cell formation [70, 71]. Moreover, the hydrolysis of lipoprotein phospholipid by sPLA2 generates free fatty acids and lysophospholipid products that may independently promote inflammation and oxidative stress within the arterial wall [72, 73]. The potential role of sPLA2 in cardiovascular disease is supported by consistent demonstration of the direct relationship between sPLA2 mass or activity and prospective cardiovascular risk in a broad range of clinical settings. Case–control studies have demonstrated that sPLA2 levels predict cardiovascular risk in asymptomatic subjects and patients with stable or unstable angina pectoris [74, 75]. Although consistent, study population in all of the above studies was relatively small and results in healthy subjects have to be replicated in other cohorts until the clinical usefulness of sPLA2 in the prediction of CHD may be established.
Myeloperoxidase
MPO, a member of the heme peroxidase superfamily, is a leukocyte-derived enzyme that generates reactive intermediates, leading to oxidative damage of host lipids and proteins [16, 76]. MPO could also be involved in the development of endothelial dysfunction because MPO uses the atheroprotective endothelial-derived nitric oxide as a substrate [77]. It has been shown that MPO is present within atherosclerotic plaque in human arteries [78]. From the results of two prospective studies in patients with chest pain or acute coronary syndrome, increasing systemic levels of MPO predicted the presence of CAD and risks of subsequent major adverse cardiac events [79–81]. In addition, this relationship remained significant after statistical adjustments for Framingham risk score and hs-CRP. Thus, MPO might be a promising prognostic marker for cardiovascular events. However, further studies are needed to replicate these findings and to establish a potential role for MPO as a predictor of incident CHD in initially healthy subjects.
Plaque Imaging Modalities for the Assessment of Residual Risks
Over the past few years, considerable advances have been made in imaging techniques for the assessment of atherosclerotic changes. Recently, various noninvasive and invasive imaging modalities enable us to not only evaluate the natural history of atheroma burden but also refine cardiovascular risk assessment. These abilities will contribute to the assessment of residual risk and the identification of patients who need more intensive therapies.
Noninvasive Imaging
Carotid Ultrasound
Noninvasive B-mode ultrasonic imaging of the carotid arteries visualizes the artery wall with resolution to detect very early thickening of the intimal–medial layer that precedes the appearance of macroscopic atherosclerosis. Multiple studies have demonstrated that carotid intima-media thickness (CIMT) predicts future cardiovascular events in diabetic patients, asymptomatic patients, and patients with CAD [82, 83] (Table 1.4). As a result, measurement of CIMT of the carotid artery can serve as a useful method for cardiovascular risk assessment.
Table 1.4
Anatomical plaque imaging modalities
Imaging modalities | Physical basis | Spatial resolution | Luminal stenosis | Plaque area | Plaque composition | Limitation |
---|---|---|---|---|---|---|
A. noninvasive | ||||||
Carotid ultrasound | reflection of high-frequency sound | >400 μm | ○ | ○ | ∆ | Interobserver variability |
Computed tomography | ||||||
1. Coronary artery calcification | X-ray | 400–600 μm | X | X | Calcium | Radiation exposure |
2. Multidetector-computed tomography | X-ray | 400–600 μm | ○ | ○ | ○ | Radiation exposure, contrast use |
Magnetic resonance imaging | Radiowaves | 150–200 μm | ○ | ○ | ○ | Unsuitable for the assessment of small arteries |
B. Invasive | ||||||
Intravascular ultrasound (IVUS) | Reflection of high-frequency sound | 100 μm (40 MHz) | ○ | ○ | ∆ | A suboptimal characterization of plaque composition |
Virtual histology-IVUS | Reflection of high-frequency sound | 200 μm (20 MHz) | ○ | ○ | ○ | Only R wave gated images, no algorithm for thrombus |
Optical coherence tomography | Near-infrared light | 10 μm | ○ | X | ○ | Limited penetration |
Near-infrared spectroscopy | Near-infrared light | N/A | X | X | Lipid | Not structural information |
Computed Tomography
Coronary Artery Calcification
Coronary artery calcification (CAC) is a characteristic of atherosclerosis and readily identified by computed tomography (CT). Multiple studies have demonstrated that CAC is an independent predictor of cardiovascular events including myocardial infarction and sudden death [84, 85]. Recent studies reported that CAC scanning improves risk stratification for coronary heart disease in the elderly patients [86] and in asymptomatic healthy subjects independent of standard risk factors [87]. Additionally, risk assessment through CAC is more accurate than standard risk factors and CRP, and refines Framingham risk stratification. It remains to be determined, however, to what degree its measurement provides an incremental benefit in risk prediction with clinical use of calcium scoring and whether its use ultimately changes the treatment strategy or outcome for individual patients.
Atherosclerotic Plaque in Coronary Arteries and Aorta
Multidetector-computed tomography (MDCT) has recently emerged as a useful imaging modality for the assessment of vessel anatomy and atherosclerotic plaque morphology in arterial beds. Recent studies using MDCT have demonstrated that plaque characteristics within coronary artery such as positive remodeling, spotty calcification, and napkin-ring enhancement are associated with a higher risk of acute coronary syndrome [88, 89]. In addition, the assessment of aortic plaque burden in thoracic aorta has been shown to be associated with increased long-term mortality in patients following cardiac surgery [90]. These data indicate that coronary and thoracic aortic atherosclerosis is a marker of cardiovascular events including all-cause death and myocardial infarction.
Magnetic Resonance Imaging
Due to recent improvements in magnetic resonance imaging (MRI) techniques, this modality can provide information on both plaque volume and composition in multiple arterial territories [91, 92]. The composition of plaque within the carotid artery on MRI has been reported to correlate with likelihood of cerebrovascular events [93]. Also, a number of studies have revealed that MRI could document a marked reduction in atherosclerotic lesion size induced by statin therapy [94, 95]. However, the data of MRI for risk assessment is still limited. Further investigation will be required.
Positron Emission Tomography
Nuclear imaging techniques such as positron emission tomography (PET) can target distinct mediators and regulators involved in the cascade of atherosclerosis. PET imaging with F18-fluorodeoxyglucose (FDG) is currently considered to be one of the most promising imaging modalities for the identification of inflammation. Preliminary studies have demonstrated greater uptake in plaques containing a large proportion of macrophages, suggesting that this approach may be used to evaluate the inflammatory composition of atherosclerotic plaque [96, 97]. There is some evidence that arterial FDG uptake is related to subsequent risk of both plaque rupture and clinical events. In a study of patients with cancer, patients with the highest FDG uptake were more likely to have a vascular event during the 6 months after PET imaging [98]. In another study focusing on patients with symptomatic carotid artery disease, those in the high FDG group had poorer outcomes over the next 6 months than subjects with initially lower FDG levels [99].
Invasive Imaging
Intravascular Ultrasound
Intravascular ultrasound (IVUS) allows robust quantitative measurements, including lumen, vessel, and plaque area, and provides information on vessel remodeling and, to a lesser extent, on its composition [100] (Table 1.4). Recently, IVUS has been increasingly used as the gold standard in trials evaluating progression or regression of plaque in the coronary arteries [101–108]. Post hoc analysis from pooled data of seven clinical IVUS trials has demonstrated the importance of control for residual risks for the prevention of ongoing disease progression [109]. In addition, IVUS-measured progression of coronary plaque has been shown to serve as a marker for future cardiovascular events [110]. Thus, monitoring atherosclerosis by IVUS enables us to reveal residual risks related to plaque progression and predict cardiovascular events.
Virtual Histology-IVUS
Virtual histology-IVUS (VH-IVUS) technique displays a reconstructed color-coded tissue map of plaque composition (fibrous, fibrofatty, necrotic core, and dense calcium) superimposed on cross-sectional images of the coronary artery obtained by grayscale IVUS [111]. The VH-IVUS map successfully predicted the histology of these with a high accuracy in detecting fibrous, fibrofatty, necrotic cores, and calcium [112]. Recently, the Providing Regional Observations to Study Predictors of Events in the Coronary Tree (PROSPECT) trial has reported that plaque composition assessed by VH-IVUS is associated with future cardiovascular events [113]. The detection of high-risk plaques may contribute to the improvement of patients care and prognosis.
Optical Coherence Tomography
Optical coherence tomography (OCT) is a recently developed intravascular imaging modality using near-infrared light (1,300 nm) to create images. Compared with various other coronary imaging modalities, the greatest advantages of OCT are its high resolution of up to 10 μm in an axial resolution and to 20 μm in a lateral resolution, which is approximately ten times higher than that of IVUS and its accuracy of tissue characterization [114–116]. Given that the increase in imaging resolution is accompanied by poor tissue penetration, coronary OCT imaging produces high-quality imaging for fibrous cap, microchannel, accumulation of lipid and macrophages below the endothelial surface [117, 118]. As a result, OCT has been proposed to have a potential role in the assessment of factors that increase the vulnerability of atherosclerotic plaque. However, it remains unknown whether OCT findings could correlate with future cardiovascular events. Limitation of OCT is poor tissue penetration. Due to this limited penetration, it is difficult to image atherosclerotic plaques in large arteries.
Near-Infrared Spectroscopy
Near-infrared spectroscopy (NIRS) is based on the absorbance of light by organic molecules. Because different molecules absorb and scatter near-infrared light differently, NIRS allows for the chemical characterization of biological tissues and can be used to assess lipid and protein content in atherosclerotic plaques [119, 120]. The probability of high lipid content at the interrogation site is displayed on a color scale and is termed a chemogram. The ability to visualize lipid cores has been validated in autopsy specimens and in vivo [121]. In these validation studies, NIRS identifies the histological hallmarks of plaque vulnerability, such as a lipid pool, a thin cap, and inflammatory cells. Further clinical studies to reveal the usefulness of NIRS for the risk assessment will be required. The major limitation of NIRS is that it only detects one characteristic of vulnerable plaque and is unable to determine depth, superficial versus deep, of the lipid core. Further advances may permit detection of other plaque features implicated in future cardiovascular events.
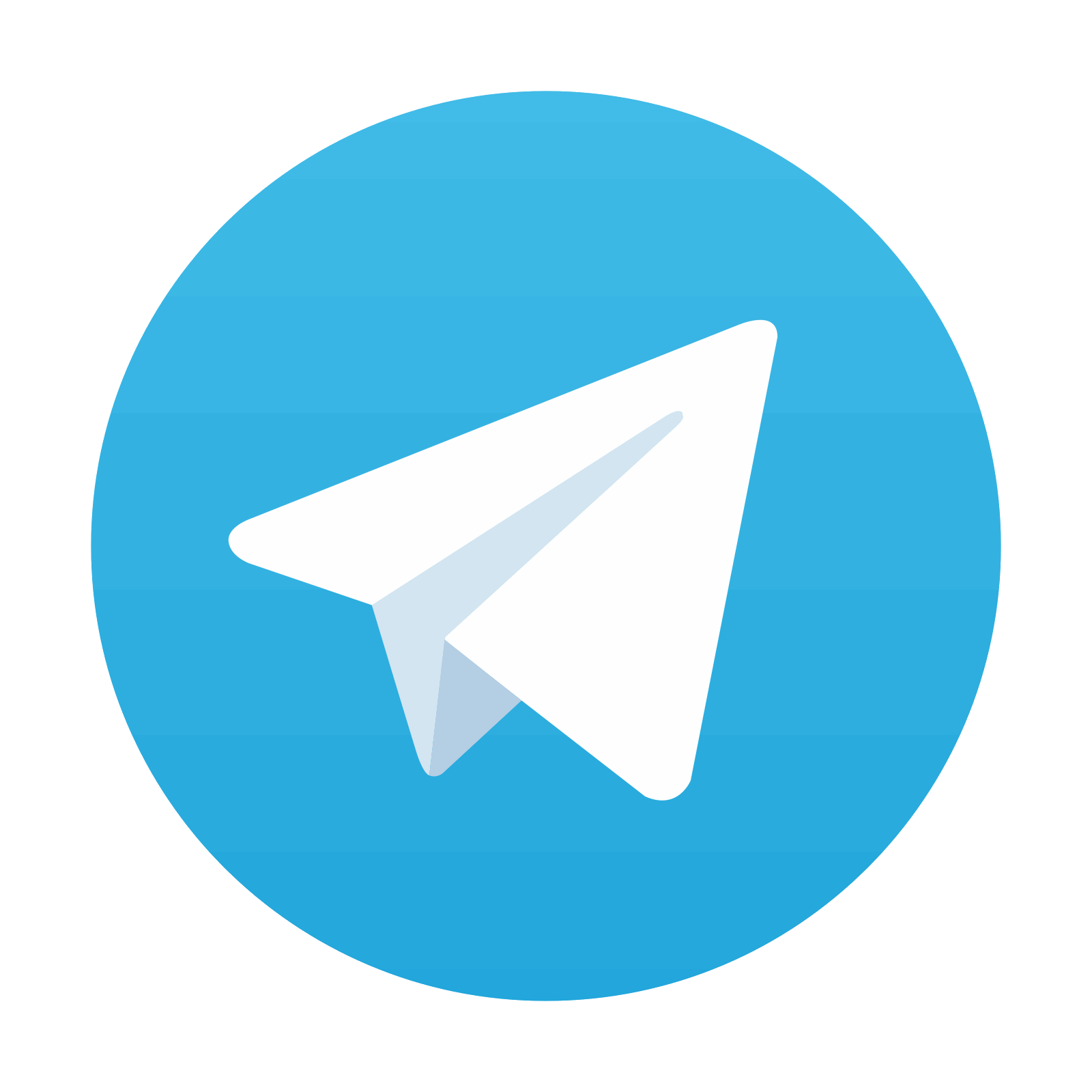
Stay updated, free articles. Join our Telegram channel

Full access? Get Clinical Tree
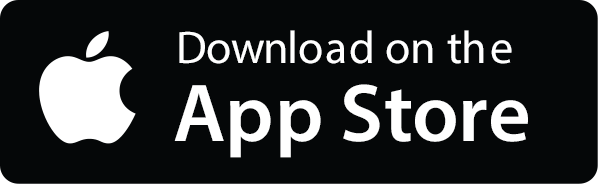
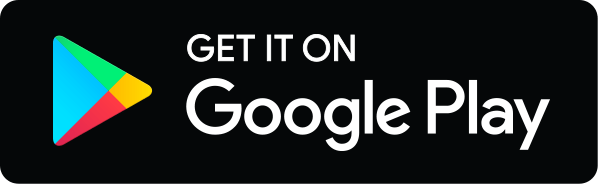