Abstract
This chapter includes the anatomy, physiology, radiopharmaceuticals, and techniques to image the respiratory system in nuclear medicine practice. There is extensive discussion of the criteria for interpreting ventilation and perfusion scans, particularly for diagnosis of pulmonary embolism. Nonembolic disease and consideration of imaging during pregnancy are presented.
Keywords
ventilation scan, perfusion scan, pulmonary embolism, interpretative criteria, asthma, COPD, pregnancy, CTPA
Chapter Outline
Lung Scanning During Pregnancy
Radionuclide lung imaging most commonly involves the demonstration of pulmonary perfusion using limited capillary blockade, as well as the assessment of ventilation using inspired inert gas, usually xenon, or technetium-99m ( 99m Tc)-labeled aerosols. Although these studies are essentially qualitative, they have an advantage over most quantitative tests of global lung function in distinguishing between diffuse and regional pulmonary disease. Most significantly, the ability to display both regional airway and vascular integrity forms the basis for the noninvasive diagnosis of pulmonary emboli. Ventilation (V) and perfusion (Q) scans are often referred to as V/Q scans. Evaluation of lung cancer and staging are discussed in Chapter 11 on positron emission tomography (PET) scanning. Some aspects of pulmonary infection and inflammation imaging are presented in Chapter 12 .
The most common indication for use of lung scintigraphy is determination of the likelihood of pulmonary embolism. Less common indications are documentation of pulmonary embolism resolution, regional quantification of lung ventilation and perfusion before lung cancer surgery, evaluation of lung transplant, bronchopleural fistula, pulmonary hypertension, cardiac shunts, and chronic pulmonary parenchymal disorders (e.g., cystic fibrosis). While computed tomography pulmonary angiography (CTPA) has become the de facto standard for pulmonary embolism evaluation (largely due to availability, speed, specificity, and accuracy), lung scintigraphy remains a valuable clinical tool, particularly in pregnant patients with a normal chest x-ray and those with poor renal function, contrast allergy, or failed or unavailable CT angiogram.
Anatomy and Physiology
The trachea divides into the right and left mainstem bronchi, and these, in turn, divide to form lobar bronchi. The lobar divisions on the right are the upper-, middle-, and lower-lobe bronchi; on the left, there are just upper- and lower-lobe bronchi. The lobes are further divided into segments based on bronchopulmonary anatomy and are shown in Fig. 6.1 . Knowledge of the anatomy of the lobes and segments of the lungs is essential for accurate interpretation of radionuclide pulmonary images.
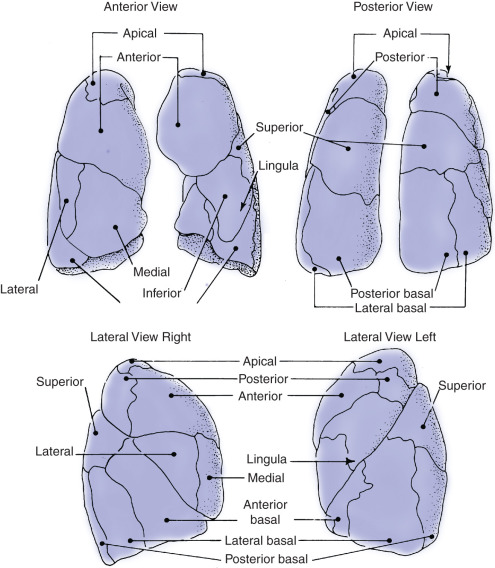
Inspiration produces a negative intrapleural pressure, which is generated by action of the thoracic cage musculature and the diaphragm. Each terminal respiratory unit or alveolus is elastic, and this elasticity provides the major impetus for expiration. Adults have about 250 to 400 million alveoli, with an average diameter of 150 µm per alveolus. It is important to remember that the direct anatomic pathway is not the only means by which air can enter the alveoli. If a bronchiole is blocked, air may get into the distal alveoli through the pores of Kohn, which provide direct communication between neighboring alveoli. In addition, the canals of Lambert connect the respiratory bronchioles and alveolar ducts. Both of these indirect pathways allow collateral ventilation in the peripheral lung and often prevent collapse of an obstructed pulmonary segment or segments.
The main pulmonary arteries divide in each lung to follow the divisions of the bronchi and bronchioles to the level of the alveoli. Each alveolus is supplied by a terminal pulmonary arteriole, which, in turn, gives rise to capillaries. The capillaries that surround the alveoli are between 7 and 10 µm in diameter. The lungs also receive blood from the aorta via the bronchial arteries, which follow the bronchial tree as far as the respiratory bronchioles. The bronchial arteries anastomose at the capillary level with the pulmonary circulation, and most of the blood from the bronchial arteries returns to the left atrium via the pulmonary veins. The bronchial circulation supplies about 5% of the blood flow to the lung under normal circumstances.
Gravity and patient position have a significant impact on both ventilation and perfusion. However, the alteration of blood flow throughout the lungs with positional change is much more marked than accompanying changes in ventilation. In the upright position, intrapleural pressure is significantly more negative at the apices than at the lung bases. As a result of this negative pressure difference, the upper lung zone alveoli are held more open in expiration than are the lower lung alveoli, which are relatively collapsed. The increased potential volume in the lung bases provides a greater change in alveolus size during inspiration than at the apices, with the net effect that ventilation (air exchange) is greater in the lower lungs. Normally, ventilation in the lower portion of the lung is about 150% of that in the apex.
Pulmonary perfusion is also unevenly distributed throughout the lungs. Maximal pulmonary blood flow normally occurs in the lung zone bracketing the junction of the lower third and upper two-thirds of the lungs. In the upright position, the apex receives only about one-third of the blood flow per unit volume as compared with the bases. In the supine position, however, perfusion is more uniform, although again, there is relatively increased blood flow in the dependent portions of the lung. In patients who demonstrate more flow to the upper lobes, congestive failure with increased left atrial pressure or α 1 -antitrypsin deficiency should be considered.
Thus in the normal, upright patient, both ventilation and perfusion increase progressively from the lung apex to the bases, although this gradient is more pronounced for perfusion. Because ventilation increases much less rapidly from apex to base, the V/Q ratio changes in the reverse direction, increasing from base to apex. In the supine position, both ventilation and perfusion gradients are less pronounced, resulting in more even ventilation and perfusion throughout the lungs.
Uncommonly, acute changes in perfusion affect ventilation; local ischemia and hypoxia can cause reflex bronchoconstriction, with a resulting shift of ventilation away from the hypoperfused areas. However, this phenomenon appears to be transient and is uncommonly demonstrated in humans. Conversely, abnormalities of ventilation commonly cause redistribution of pulmonary perfusion; hypoventilation leads to regional hypoxia and reflex vasoconstriction with redistribution of perfusion away from the hypoventilated regions.
Radiopharmaceuticals
Perfusion Imaging Agents
Technetium-99m macroaggregated albumin (MAA) is the radiopharmaceutical used for pulmonary perfusion imaging. It localizes by the mechanism of capillary blockade. In general, fewer than 1 in 1000 (< 0.1%) of the capillaries are blocked. In the absence of shunts, 95% of the particles are removed from the circulation on the first pass through the pulmonary capillary bed. About 5% of particles measure less than 5 µm in diameter and pass through the capillary system. For purposes of pulmonary perfusion imaging, it is important to use a sufficient number of particles to allow for good statistical distribution. In general, for adults, injection of a minimum of 100,000 particles, and optimally between 200,000 and 700,000 particles, is required.
The production of 99m Tc-MAA entails aggregation of human serum albumin using heat and a reducing agent to form the particles. Visual inspection of the preparations through the use of a microscope and hemocytometer demonstrate whether the MAA particles are too large or have clumped. The particle size of 99m Tc-MAA generally ranges from 5 to 100 µm, with most in the range of 10 to 30 µm. MAA has a biologic half-life in the lung of 2 to 4 hours, depending on the kit manufacturer and preparation. Some preparations begin to break down as early as 30 minutes after injection. The particle fragments enter the general circulation as smaller particles, which are usually removed from the circulation by the liver and spleen. The normal administered activity in adults is 1.1 to 4.1 mCi (40 to 150 MBq). The lung is the critical organ. The absorbed dose to the lung is variable but is about 0.7 rad (7 mGy) for a 3-mCi (111-MBq) dose.
Technetium-99m MAA should be injected during quiet respiration, with the patient supine to minimize the normal perfusion gradient between the apex and lung base. Because the MAA particles tend to settle out in solution, the syringe should be gently agitated before injection to ensure even mixing. A peripheral vein is preferred, and administration through a Swan-Ganz catheter or any indwelling catheter port containing a filter generally should be avoided. To assist in homogeneous pulmonary distribution of the particles, injection should be made slowly, usually over three to five or more respiratory cycles, and the injected volume should be at least 1 to 2 mL. If blood is drawn into the syringe to confirm an intravascular needle location, it is important not to let the blood sit long in the syringe because this may allow the formation of small, clinically insignificant, labeled blood clots, which, when injected, result in focal hot spots on the perfusion scan ( Fig. 6.2 ).
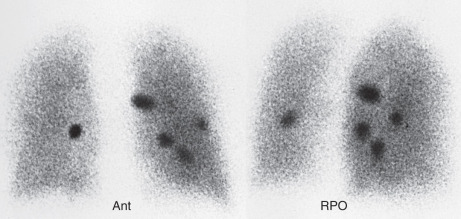
A relative contraindication to performing particulate perfusion lung scans is severe pulmonary hypertension because the blockade of additional lung capillaries may acutely exacerbate the condition and its cardiac complications. Care should also be taken in patients with known right-to-left shunts, although adverse effects on the coronary or cerebral circulations have rarely been observed. In these patients, however, it is probably prudent to reduce the number of injected particles to 100,000. The presence of a right-to-left shunt can be easily recognized on posterior images by the immediate presence of renal activity, usually best seen on the posterior or lateral views, and can be confirmed by the demonstration of activity in the brain ( Fig. 6.3 ). Sample imaging protocols, number of particles for children, and dosimetry are presented in Appendix E .
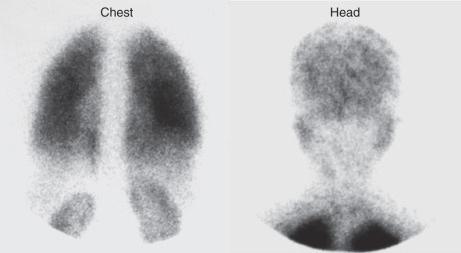
Ventilation Imaging Agents
Radiolabeled Aerosols
Technetium-labeled radioactive aerosols are commonly used to image ventilation. Unlike ventilation studies using radioactive gases, aerosol studies do not allow for dynamic single-breath or washout phase imaging but rather map the distribution of aerated lung volume. Once deposited in the lungs, the aerosol particles remain in place for sufficient time to permit imaging in multiple projections. Preparation of 99m Tc–diethylenetriamine pentaacetic acid (DTPA) aerosol begins with the injection of about 25 to 35 mCi (0.9 to 1.3 GBq) of 99m Tc-DTPA in a volume of 2 mL into the nebulizer of an aerosol delivery system. Oxygen tubing is then connected to the side port, and oxygen is supplied through a flow meter. Flow rates are in the range of 7 to 10 L/min. A mouthpiece with a nose clip is then used to administer the aerosol. If necessary, this can be connected to an endotracheal tube. The patient is usually in a supine position (which allows for an even distribution of aerosol) and breathes at tidal volume for 3 to 5 minutes.
The aerosols usually have a mean aerodynamic diameter of about 0.5 µm. The half-time clearance time from the lungs is 45 to 60 minutes in nonsmokers and 20 minutes in smokers. The larger the particle size, the more central its deposition in the bronchial tree. Central deposition is also common in patients with chronic obstructive pulmonary disease (COPD), probably owing to turbulent flow in the central airways compared with that of healthy people. The 99m Tc-DTPA aerosol for ventilation imaging has several advantages, the most notable of which are the ready availability of 99m Tc and its ideal imaging energy. Little patient cooperation is required. The aerosol can be delivered in a room separate from the camera room and can easily be delivered through mechanical ventilators or during normal tidal breathing. There is no need for special exhaust systems or traps, and thus it can be used on a portable basis in intensive care units.
In most patients, DTPA aerosol particles cross the alveolar-capillary membrane with a half-time of about 1 hour, enter the pulmonary circulation, and from there are rapidly cleared by the kidneys. In smokers, clearance is significantly accelerated because of increased alveolar membrane permeability.
One of the major disadvantages in the use of 99m Tc-DTPA aerosols is the small amount of activity actually delivered to the patient (2% to 10%) compared with that available in the aerosol generator. Usually about 0.5 to 1.0 mCi (20 to 40 MBq) is actually being delivered to the patient. Another limitation is rapid lung clearance in patients with inflammatory lung disease. Because both MAA and DTPA aerosols are labeled with 99m Tc, sequential imaging of ventilation and perfusion requires the relative doses of each to be adjusted to prevent interference of one 99m Tc-labeled agent with the other when imaging is performed.
Radioactive Gases
The use of radioactive inert gases to evaluate ventilation permits sequential imaging of both lung ventilation and perfusion in conjunction with 99m Tc-MAA because of the rapid clearance of the gases from the lungs and the relative energy differences of the photon emissions.
Xenon-133 ( 133 Xe) is the primary isotope used for assessment of ventilation. It is relatively inexpensive and has a half-life of 5.3 days and a principal gamma ray energy of 81 keV. The low energy of these photons causes about half of them to be attenuated by 10 cm of inflated lung tissue. Thus overlying soft tissues, such as breasts, can produce substantial artifacts; these are usually avoided by performing xenon ventilation scans in the posterior position. The critical organ for 133 Xe is the trachea. Xenon-133 allows for the assessment of all phases of regional ventilation: initial single breath, wash-in, equilibrium, and washout. Single-breath images represent instant ventilation, wash-in and equilibrium images are proportional to aerated lung volume, and washout phases show regional clearance of activity from the lungs and delineate areas of air trapping. This complete characterization of ventilation renders 133 Xe imaging the most sensitive ventilation study for detection and assessment of airway disease.
Ventilation examinations are generally performed either to assess regional ventilation or to improve the specificity of a perfusion scan. Ventilation imaging using 133 Xe is limited in that images are usually obtained in only one projection and are performed before the perfusion study. The use of a single-projection image ensures that some regional ventilation abnormalities will be missed because the lungs are not entirely imaged.
The ventilation study is usually performed before the perfusion scan, using upright posterior views. The posterior view is selected because it is technically convenient and allows a ventilation survey of the greatest number of pulmonary segments with the least amount of overlying soft tissue. Although there are several common methods of performing ventilation imaging, the most complete involves three phases: (1) single wash-in or initial breath, (2) equilibrium, and (3) washout. Ventilation imaging with 133 Xe requires a considerable amount of patient cooperation because the patient must be able to tolerate breathing on a closed spirometer system for several minutes to reach equilibrium.
The single-breath phase involves having the patient exhale as deeply as possible and then inhale 10 to 20 mCi (370 to 740 MBq) of 133 Xe, holding his or her breath for about 15 seconds while a static image is taken. The equilibrium phase constitutes the rebreathing of the expired xenon diluted by about 2 L of oxygen contained in a closed system. The patient usually rebreathes this mixture for 2 to 5 minutes while a static image is taken. Thus, the 133 Xe image obtained at equilibrium essentially represents the distribution of aerated lung volume. After equilibrium is reached, fresh air is then breathed during the washout phase while serial 15-second images are obtained for 2 to 3 minutes as the xenon clears from the lungs. In patients with COPD, the washout phase may be prolonged to 3 to 5 minutes, if necessary, to assess areas of regional airway trapping.
Xenon-133 is usually administered by using one of a number of commercially available delivery and rebreathing units. These generally allow the disposal of expired xenon by one of two methods. The simplest way is to exhaust the xenon to the atmosphere using a dedicated exhaust system. A more common method is to use an activated charcoal trap to accumulate the exhaled xenon gas until it has decayed to background. Although not required by specific regulation, 133 Xe imaging is optimally performed in a room with negative pressure in case of accidental leakage from the closed system, especially during administration to the patient. The exhaust vent should be placed near the floor because xenon is heavier than air.
There are three agents used in Europe that are not currently available in the United States. Krypton-81m ( 81m Kr) has been advocated for use in ventilation imaging. Krypton generators using the parent isotope rubidium-81 ( 81 Rb) are available, but they have a short shelf-life (half-life, 4.6 hours) and are inconvenient and expensive. 99m Tc-Technegas (Cyclomedica) consists of ultrafine carbon particles that behave physiologically like a gas at wash-in but lodge in alveoli. The positron emitter 68 Ga has been labeled to carbon nanoparticles and to MAA for use as PET V/Q agents.
Technique
Sample technique protocols and dosimetry are presented in Appendix E .
Because most clinical situations dictate the performance of both ventilation and perfusion studies, the question may arise as to which study to perform first. When 99m Tc-DTPA aerosol is used, the relative doses of 99m Tc-MAA and the 99m Tc-DTPA aerosol must be adjusted, with a reduction in the dose of the initial examination, depending on which is performed first. When 133 Xe gas is used, it is customary to begin with the ventilation because of the lower photon energy of xenon and its rapid clearance from the lungs. The disadvantage of this order is that the ventilation study may not have been performed in the projection that best evaluates the region of a subsequently demonstrated perfusion defect. If the perfusion study is performed first and followed by the ventilation examination, it is helpful to decrease the dose of 99m Tc-MAA and increase the dose of 133 Xe. This minimizes the effect of any Compton scatter from the 99m Tc that may occur in the xenon window of the pulse height analyzer and degrade the ventilation images.
A number of authors have shown that the accuracy of lung scans can be improved somewhat by performing single-photon emission computed tomography (SPECT) lung imaging (so called SPECT V/Q or V/P SPECT ). Xenon cannot be used for SPECT ventilation studies but 99m Tc-DTPA aerosol can be used. Other described techniques include SPECT V/Q combined with low-dose lung computed tomography and SPECT 99m Tc-MAA perfusion images fused with CT. At the present time, there are a limited number of clinical comparison studies, and these more complicated techniques are not commonly used or available at most community and some major hospitals.
Normal Lung Scan
Perfusion Scan
A normal perfusion scan is shown in Fig. 6.4 . In the posterior projection, there is some tapering of activity toward the bases as a result of the thinning of the lungs in the region of the posterior sulci. In the anterior view, the cardiac silhouette and the aortic knob are commonly identified. The left lateral view may show a substantial anterior defect because of the heart. A cardiac silhouette considerably larger than expected from the chest radiograph may occasionally be produced by hypermobility of the heart when lateral images are obtained, especially in the decubitus positions. In the lateral gamma camera views, about one-third of the image statistics (or counts) come from the contralateral lung. This “shine through” frequently allows enough photons to be collected from the opposite lung to render a normal lateral image, even in the presence of a prominent defect seen in one lung on the anterior or posterior view. Oblique projections are often helpful but may be confusing to the uninitiated observer and frequently demonstrate prominent hilar defects. In general, defects suspected on the oblique projections should be confirmed on one of the four standard views.
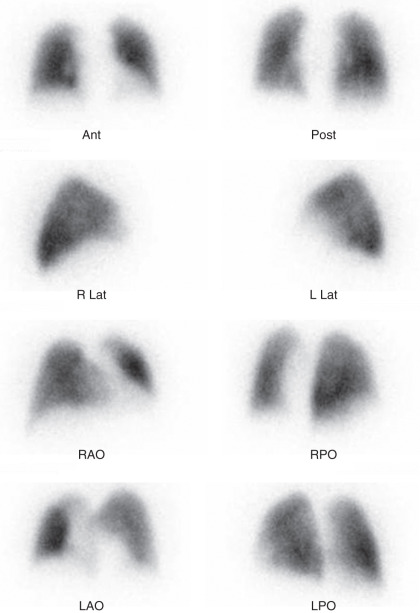
Pleural disease may produce distinctive changes on an otherwise normal perfusion scan. Small pleural effusions may best be seen on the lateral or oblique views as posterior sulcus blunting or as a fissure sign, a linear defect caused by fluid in an interlobar fissure ( Fig. 6.5 ). The fissure sign may also be produced by pleural scarring or thickening (even when not apparent on chest radiographs), by COPD, or, rarely, by multiple pulmonary microemboli. Moderate-sized pleural effusions may occasionally simulate segmental defects; if scanning is performed in the supine position in a patient with a pleural effusion, the fluid may collect in the superior part of the major fissure and mimic a superior-segment, lower-lobe defect. This defect, however, may disappear in the upright position. If an effusion is large, it may compress an entire lung and decrease the blood flow to that side generally or may surround the lung, producing the appearance of a small lung.
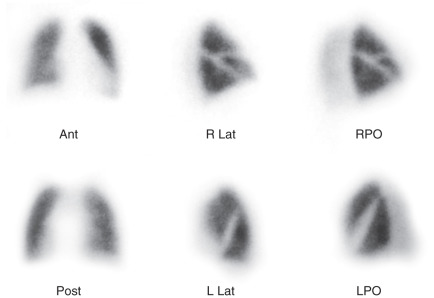
Perfusion defects occur incidentally in asymptomatic people with normal chest radiographs and without a clinical history of pulmonary emboli. It appears that small pulmonary emboli are a normal physiologic occurrence. Incidental detection of small PE occurs in about 15% to 20% of mechanically ventilated or trauma patients or those over age 80.
Ventilation Scan
A normal 133 Xe ventilation study performed in the posterior projection is shown in Fig. 6.6 . After the initial breath, a relatively homogeneous distribution of activity should be seen throughout both lungs; the initial breath image reflects regional ventilatory rate if there is maximum inspiratory effort. The equilibrium-phase image indicates the aerated volume of lung and may be thought of as the “scintigraphic chest radiograph.” Even in patients with abnormal single-breath and washout images, the equilibrium phase is frequently normal, particularly if rebreathing is performed for several minutes so that adequate collateral ventilation can occur. During the washout phase, activity clears from the lower portions of the lung at a faster rate than from the apices because the air exchange is greater. However, activity is frequently seen longer at the bases, owing to the relatively larger volume of lung present in that region. In most normal studies, the lungs are almost completely clear of xenon within 2 or 3 minutes of beginning the washout phase because the normal half-time for xenon washout is about 30 to 45 seconds. Because washout is the most sensitive phase for the detection of trapping caused by airway obstruction, if xenon gas does not enter an area during equilibrium, washout cannot be evaluated. Thus, to a large extent, the sensitivity of the washout phase depends on performing sufficient rebreathing to obtain adequate equilibrium in as much of the lung volume as possible as well as on the length of the washout phase.
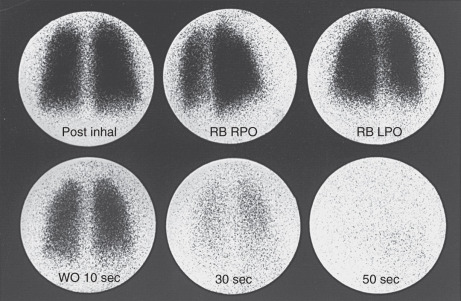
Because xenon is soluble in fat and partially soluble in blood, it may be deposited in the liver, resulting in increased activity in the right upper quadrant. This becomes apparent near the end of the xenon washout study and should not be mistaken for trapping of xenon in the right lower lung. The finding is particularly prominent in patients with disorders producing a fatty liver ( Fig. 6.7 ). In children, activity may be seen in the left upper quadrant of the abdomen because of swallowing of the xenon gas during the study.
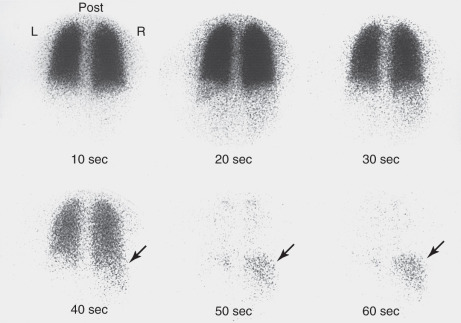
Normal DTPA aerosol ventilation images demonstrate homogeneous, symmetric aerosol deposition from apex to base ( Fig. 6.8 ). Areas in which there is no activity represent nonventilated regions. Normal aerosol scans resemble perfusion scans, except that the trachea and the bronchi are commonly visualized. In addition, swallowed activity can sometimes be seen in the esophagus and stomach.
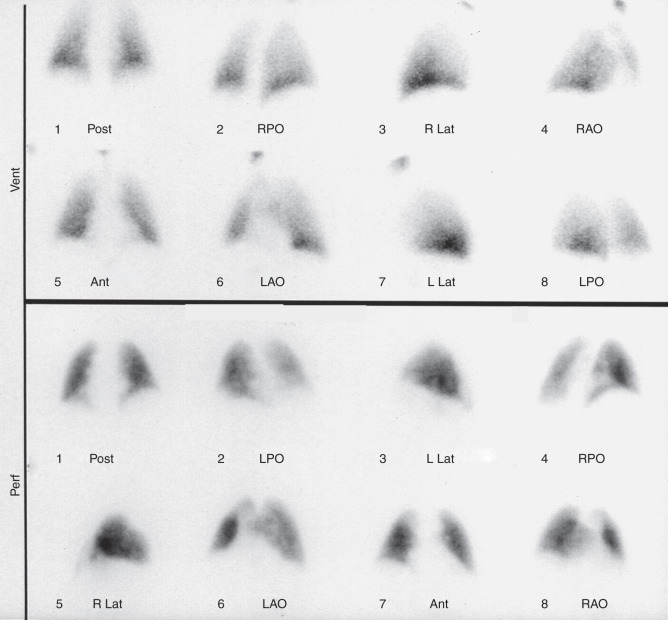
Clinical Applications
By far, the most important and frequent indication for a V/Q lung scan is in the setting of suspected pulmonary emboli. Regardless of the reason for performing V/Q pulmonary imaging, however, it cannot be overemphasized that a high-quality recent chest radiograph and pertinent clinical and laboratory findings should be fully used when interpreting lung scans. Use of poor-quality portable supine films can lead to mistakes in interpretation, although sometimes these are all that are available. Ideally, full-inspiration upright posteroanterior and lateral chest radiographs should be obtained as near as possible to the time the lung images are performed, certainly within 12 to 24 hours before performance of the scan or shortly thereafter.
Pulmonary Emboli
Pulmonary thromboembolism is a potentially fatal complication of deep vein thrombosis. Although anticoagulation and thrombolytic therapies are effective, they are not without potential morbidity. Thus, before the institution of treatment, determination of the reasonable likelihood of the presence or absence of pulmonary emboli is needed.
The clinical diagnosis of pulmonary embolism is often difficult. Less than one-fourth of patients with pulmonary emboli show the classic signs or symptoms of the disease; hemoptysis is seldom observed, and blood enzyme and D-dimer determinations may be equivocal. Chest radiographic findings alone are nonspecific for the diagnosis of pulmonary embolism. However, an adequate chest radiograph is essential to diagnose conditions that can clinically mimic pulmonary emboli and is an important component of the interpretation of V/Q lung scans. Clinical presentations are frequently vague and may be mimicked by a variety of thoracic and abdominal disorders, although the utilization of clinical pretest probability schemes, such as the modified Wells and simplified, revised Geneva scores, have proved useful. A summary of appropriate use criteria for V/Q imaging for pulmonary embolism in various clinical scenarios is presented in Box 6.1 .
Appropriate
PE likely; male or non-pregnant female, with normal or mildly abnormal chest x-ray
PE likely; patient with abnormal renal function or at risk for contrast complication
PE likely; CTA chest inconclusive or discordant with clinical probability
PE likely; hemodynamically unstable patient with portable V/Q equipment available
PE likely; lower extremity with clot
PE likely; pregnant patient with normal or mildly abnormal chest x-ray (low-dose perfusion only)
PE likely; negative D-dimer
PE unlikely; positive D-dimer
Recent/prior documentation of PE, suspected new PE, previous V/Q
Recent documented PE now on anticoagulation. Imaging to document disease status when clinically indicated, previous V/Q
May be Appropriate
PE suspected, male or non-pregnant female, significantly abnormal chest x-ray
PE likely; patient cannot cooperate with ventilation imaging, perfusion only
PE likely; patient ventilator dependent
Rarely Appropriate
PE unlikely; D-dimer negative
PE likely; patient hemodynamically unstable, portable V/Q equipment unavailable
PE likely; pregnant patient with severely abnormal chest x-ray, perfusion only
Recent/prior PE; suspected new PE, previous CTPA
Recent documented PE now on anticoagulation. Imaging to document disease status when clinically indicated, previous CT
CT , Computed tomography; CTPA , CT pulmonary angiography; PE , pulmonary embolism; V/Q , ventilation/perfusion.
Although multislice spiral CTPA may be the initial test of choice to diagnose pulmonary embolism in most institutions, radionuclide V/Q or perfusion only imaging, when properly performed and interpreted with a current chest radiograph, is an effective lower radiation dose procedure for the detection of pulmonary embolus. A normal perfusion scan essentially excludes the diagnosis.
Diagnostic Principle
The diagnosis of pulmonary thromboembolism by V/Q imaging is based on the disassociation between ventilation and perfusion as a result of the obstruction of pulmonary segmental arterial blood flow by the embolus. With 99m Tc-MAA imaging, the MAA particles are unable to enter the capillary bed distal to the arterial occlusion, so that the portion of lung supplied by the involved artery appears as a perfusion defect outlined by the normally perfused adjacent lung parenchyma. Because ventilation is generally unaffected, the xenon or aerosol images remain normal in the same distribution. Thus the most typical manifestation of the pulmonary emboli is as a wedge-shaped perfusion defect with preserved ventilation: the segmental V/Q mismatch ( Fig. 6.9 ).
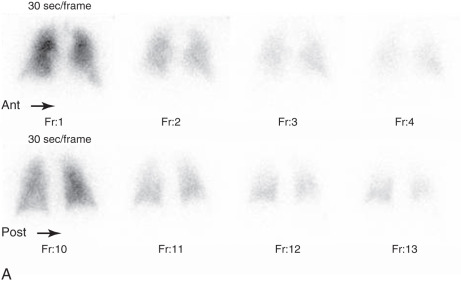
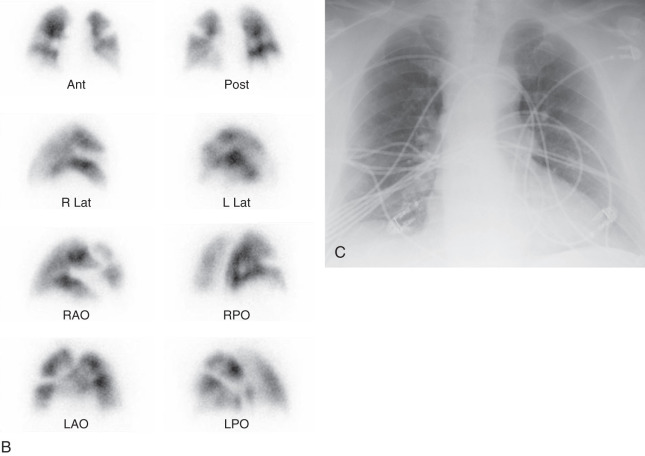
Although this principle is simple, its practical application in various clinical settings can present one of most difficult challenges confronting the nuclear imaging physician. For this reason, much effort has been directed toward defining the language used to describe the findings on V/Q imaging, as well as the criteria used to translate them into diagnostic conclusions. In this regard, a thorough understanding of basic definitions and concepts is imperative.
Basic Concepts
A normal pulmonary perfusion scan has no perfusion defects or perfusion exactly outlines the shape of the lungs seen on the chest radiograph. It should be noted that a normal perfusion scan may demonstrate hilar and aortic impressions and the chest radiograph and/or ventilation study may be abnormal. A normal perfusion scan essentially excludes the presence of clinically significant pulmonary emboli, regardless of the ventilation scan findings.
A perfusion defect is a focus of absent or diminished pulmonary activity on perfusion images. Perfusion defects are nonspecific, and a list of differential diagnoses is given in Box 6.2 . With a pulmonary embolism, perfusion does not have to be completely absent in the region of the defect because partially occluding pulmonary emboli are possible. Perfusion defects are classified as segmental or nonsegmental.
Pulmonary embolism (thrombotic, septic, marrow, or air) a
a Entity may cause a ventilation/perfusion mismatch.
Bulla or cyst
Localized hypoxia caused by asthma, bronchitis, emphysema
Surgery (e.g., pneumonectomy)
Pleural effusion a
Tumor (including hilar or mediastinal) a
Metastases (hematogenous or lymphangitic)
Hilar adenopathy (lymphoma, sarcoidosis)
Pulmonary artery atresia or hypoplasia a
Fibrosing mediastinitis a
Radiation therapy a
Pneumonia
Pulmonary edema
Atelectasis
Fibrosis (postinflammatory, postradiation, pleural thickening)
Vasculitis a
Segmental perfusion defects may involve all or part of a bronchopulmonary anatomic segment. Classically, these defects are pleural based and wedge shaped. They are best described by the particular bronchopulmonary segment that they occupy. Because segmental defects are the hallmark of pulmonary emboli and nonsegmental defects are not, the ability to distinguish between them is crucial to lung scan interpretation. This requires both careful characterization of defect configuration and an appreciation of the pulmonary segmental anatomy as it appears on perfusion lung images. Fig. 6.10 shows the individual pulmonary segments in the projection that best visualizes each segment.
