Fig. 4.1
The annual number of ICG publications according to PubMed (27.3.2014; “indocyanine green”)
Here ICG fluorescence imaging is reviewed in the areas of plastic and reconstructive surgery, neurosurgery, cardiac, oncological, vascular, and hepatic surgery for the years 2012 and 2013 since our previous review of ICG imaging in surgery [2]. The large area of ICG imaging and angiography in ophthalmological applications has been excluded in this review. In addition to clinical applications, some technical aspects and potential future developments of ICG-based fluorescent imaging are discussed.
Diagnostic Applications
Liver function is assessed with several biochemical and other tests to detect the presence of liver disease, to evaluate the extent of liver damage and to follow the response to treatment. Especially preoperative assessment of liver function can help with the decision of the extent of a hepatic resection. These evaluations are important in preventing postoperative liver failure and mortality after hepatic resection.
Hoekstra et al. reviewed several clinically used liver function tests and concluded that due to the complexity of liver function, one single test does not stand for overall liver function [3]. Nevertheless, studies have shown that the ICG clearance test is a sensitive indicator to gauge liver function [4, 5]. After intravenous injection ICG bound in plasma proteins is taken up by hepatocytes and excreted in bile via an active transport system. Since ICG is not metabolized, its disappearance rate from plasma to bile reflects the excretory function of the liver [6]. ICG retention rate measured at 15 min (ICG-R15) has been reported to act as a significant predictor for postoperative outcome [7, 8]. In healthy liver ICG-R15 is usually less than 10 %, whereas 20 % is generally considered as a safety limit for extended hepatectomy in chronic liver disease [9, 10]. To aid the decision making process in surgical treatment of diseased liver, Makuuchi and Sano established an algorithm based on the following three parameters: the presence or absence of ascites, total bilirubin level, and the ICG-R15 [11]. Operability is assessed by the presence or absence of ascites and total bilirubin level, whereas the maximal resectable liver volume is assessed by ICG-R15. Though ICG-R15 test is very informative and widely accepted method, discrepancies still exist between ICG measurements and conventional liver function tests. Conditions such as hyperbilirubinemia and large port-systemic shunts interfere with the results of ICG tests [12, 13]. Additionally, a few patients are intolerant of ICG [14].
Orthotopic liver transplantations are often followed by early postoperative complications. Inconsistencies exist in graft evaluation results making the initial graft function problematic to determine. ICG plasma disappearance rate has been shown to predict absence of early postoperative complications, better and earlier than clinically used laboratory parameters [15, 16]; whereas, a lack of prognostic value to predict individual patient outcomes has also been shown [17].
ICG dynamics studies by Kang et al. suggest the possibility of ICG imaging for reliable diagnosis of Raynaud phenomenon [18]. A potential use of the ICG perfusion imaging as an effective evaluation tool to validate the vasoactive effect of acupuncture was also shown for normal healthy volunteers [19].
Surgical Applications
The binding and spectral properties of ICG have enabled the development of intraoperational imaging systems for several surgical specialities.
Reconstructive (Plastic) Surgery
There are three main purposes in reconstructive surgery, where indocyanine green imaging (ICGI) has been used and developed: For assessment of skin flap viability in elective surgery or traumatic skin lacerations; for intraoperative patency testing of microvascular anastomosis; and for postoperative flap monitoring.
Initially, ICGI was used for assessing viability of skin flaps formed by traumatic degloving injuries and depth of burn injuries [20, 21]. Although this method showed potential, it never gained wide popularity among clinicians. However, breast surgeons performing skin sparing mastectomies have adopted ICGI for evaluating viability of breast skin envelope before reconstruction [22–25]. Intraoperative use of ICGI might lead to decreased complication rates associated with skin necrosis [25].
Similarly, ICGI can be used to assess the perfusion of large cutaneous flaps, both microvascular and pedicled, used in reconstructive surgery. Using ICGI could reduce complications associated with partial flap necrosis due to insufficient perfusion to distal parts of the flap [26–35]. When combined with operational microscope, ICGA can be used to detect anastomotic failure after microvascular tissue transplantation [36]. The technical success of the anastomosis can easily be evaluated with ICGA through the operational microscope, and furthermore, the intristic transit time can be calculated [37]. This may prevent complications associated with anastomotic failure, which is a common cause of failures in reconstructive microsurgery.
After transplantation, ICGI can be used for flap monitoring. However, ICGI is more invasive than other commonly used methods and cannot be done real time. Instead, ICGI is more feasible in situations, where failure of the microvascular anastomoses is suspected based on clinical findings. When combined with endoscopy, ICGI can also be used to monitor flaps that are inside the oral cavity or in the upper digestive tract, and would otherwise be difficult to monitor [30, 38, 39].
Sentinel Lymph Node Biopsy
Sentinel lymph node (SLN) biopsy is a standard method for lymph node staging in breast cancer surgery. This avoids axillary lymphadenectomy in many patients, reducing associated morbidity. Technetium 99 m (99mTc) radiotracer is the most commonly used substance for locating the SLN(s) [40]. The isotope is injected next to the primary tumor, and drains through lymphatic vessels to the regional lymph nodes. The radiotracer is found using a gamma detector probe intraoperatively. Recently, ICGI has been used to detect SLNs in breast cancer patients. According to the first studies, ICGI is able to find the same lymph nodes as conventional radiotracer [40–51]. Also, ICG has been combined with 99mTc as a hybrid radiotracer, where it can be used to ease the detection of SLN, and to replace blue dye [52]. This is a promising aspect, since 99mTc is still believed to be a more reliable tracer, especially for deep sitting SLNs and in obese patients [47].
Skin Cancer Surgery
As well as in breast cancer surgery, SLN biopsy is used extensively in surgical treatment of skin malignancies. Melanoma and other forms of skin cancer, such as squamous cell carcinoma, some forms of basal cell carcinomas, and several other carcinomas can metastasize to regional lymph nodes. As well as in breast cancer, 99mTc has been the standard tracer. However, using ICGI for locating SLNs in skin cancer has some benefits. The fluorescence of ICG travelling from the injection site to the SLN can be traced real time, possibly enabling identification of SLNs in less common areas such as the cervical, popliteal, or parascapular areas [53]. ICGI is performed intraoperatively, and saves the patient and the staff from radiation associated with a radiotracer. The detection rate of SLNs using ICGI seems to be similar to using conventional tracers such as 99mTc, and the cost of ICG is also lower [54–58].
There are publications reporting the use of ICGI for SLN detection in vulvar carcinoma, cervical carcinoma, endometria carcinoma, and especially in head and neck carcinomas [59–65]. Yokoyama et al. also studied the optimal time between ICG injection and imaging, which seems to be in the interval from 30 min to 2 h [65]. In addition, they showed that ICG imaging is suitable for safe navigation in narrow spaces like the parapharyngeal space [66].
Lymphatics
Lymphastasis is a restriction of lymphatic flow out of an extremity, which may lead to chronic swelling of the affected extremity, lymphoedema. Although sometimes idiopathic, lymphastasis is most often secondary to surgical removal of lymphnodes in cancer surgery. Until recently, treatment of lymphoedema has mostly been conservative, but recent advancements in microsurgical techniques have made surgical treatment of lymphoedema possible.
Recently, ICGI has been used as a diagnostic tool to detect lymphastasis, both primary and secondary, where it seems to give equivalent results than lymphoscintigraphy, but with less invasiveness [67–71]. The findings of ICGI in lymphoedema patients also correlate with anatomical findings during surgery [72]. Early diagnosis of lymphastasis is valuable, because conservative treatment can be initiated already before onset of lymphoedema [73, 74].
An increasingly popular operation to treat upper and lower limb lymphoedema is connecting lymphatic vessels to subdermal veins (lymphaticovenous anastomosis). ICGI has become an integral part of planning and executing these procedures; ICGI is used to detect suitable lymphatics in the affected area before and during the operation [75–77]. The success of anastomosis is easy to assess by intraoperative ICGI through the operational microscope [78].
Neurosurgery
Over the last 10 years, intra-operative ICG angiography has become a standard tool for neurosurgeons operating on various vascular lesions of the brain. Microneurosurgical operations of the brain and spinal cord are an ideal area for the use of ICG in general due to three boundary conditions which are met: (1) All the surgeries are performed under the magnification of an operating microscope which can be fitted with all the necessary camera and filter systems; (2) The arteries and veins on the surface of the brain are exposed during the procedure and thus they can be seen directly by visual means; and (3) The ischemic time for brain tissue is very short so any method for providing information about the blood flow inside the cerebral vessels needs to be fast and easy to use.
Already in 1994 Wrobel et al. started to use the fluorescein for the intraoperative assessment of arterial branches and their patency during intracranial aneurysm surgery [79]. They worked with a complex and inconvenient camera and filter system which prevented the technique to become widespread at that time. In 2003 Raabe et al. published their first experience with the use of ICG video angiography in patients with various vascular lesions of the central nervous system [80], and later in 2005 the same group reported the use of ICG angiography integrated into a surgical microscope (Carl Zeiss Co., Oberkochen, Germany) [81]. A microscope-integrated light source containing infrared excitation light illuminated the operating field. The ICG dye was injected intravenously into the patient, and intravascular fluorescence from within the blood vessels was imaged using a video camera attached to the microscope. In 2005–2007 the ICG video angiography became commercially available and various groups around the world started to use it in clinical practice.
Before ICG angiography, the only rapid method to evaluate flow inside intracranial arteries was using a micro-Doppler flow probe which, unfortunately, is a rather unreliable method producing false-positive and false-negative results frequently and cannot be used for evaluating very small perforating vessels. On the other hand, intra-arterial digital subtraction angiography (DSA), the gold standard of brain vessel imaging, is a much more time-consuming method requiring significant infrastructure as well as experience to be readily available during neurosurgical operation. The introduction of ICG video angiography changed the game completely. Suddenly, there was a technique which was fast and easy to use with reliability close to that of DSA [82]. Nowadays, ICG video angiography is considered a must in centers performing high-quality microneurosurgery.
The most important clinical use for ICG video angiography in neurosurgery is in microsurgical treatment of different arterial and venous malformations, some congenital other acquired. ICG angiography has been mainly useful in: (a) evaluating intracranial aneurysm occlusion as well as patency of the surrounding branches [81, 82]; (b) treatment of brain arteriovenous malformation (AVMs) where ICG can be used to distinguish between arterial feeders and arterialized veins as well as for indirect evaluation of whether the whole AVM nidus has been removed [83]; (c) treatment of both cranial and spinal dural arteriovenous fistulas (dAVFs) for intraoperative identification of the fistulous region [84]; and (d) cerebral bypass surgery both for evaluation of anastomosis patency as well as for identification of recipient arteries [85]. In addition, the ICG video angiography has been also used for some less frequent purposes such as planning of approach to cortical tumors, identification and manipulation of important veins in their vicinity, and extracranial carotid and vertebral artery surgery.
Lately, a new fluorescent module has been introduced in neurosurgery, the Yellow 560 (Carl Zeiss, Germany). It visualizes fluorescent dyes in the wavelength range from 540 to 690 nm. It allows for highlighting the fluorescence-stained structures (e.g. with sodium fluoresceine) while viewing non-stained tissue in lifelike color. There is only limited experience with this application but main focus has been on intraoperative delineation of highly vascularized tissue of malignant brain tumors to allow for better distinction from the unaffected surrounding brain [86]. In general, ICG video angiography is an integral tool in modern vascular neurosurgery, but it has to be coupled with other imaging techniques for optimal visualization.
Adult Cardiac Surgery
At the moment, fluorescence imaging in cardiac surgery is an emerging technique for quality assessment namely in coronary artery bypass grafting (CABG). It possesses an opportunity to systemically improve the quality of the operational outcome. However, the outcome of CABG is already good, the 30-day survival rate being more than 99 % in relatively low-risk patients [87]. Thus the impact of fluorescence imaging in cardiac surgery is limited, but there clearly is a technical niche for its use. This suggestion is based on the fact that immediate graft failure occurs in up to 4 % of grafts, which relates to 8 % of the operated patients. At the time of the discharge after the operation, the graft occlusion rate is reported to be as high as 5–20 %. At 1 year after the operation, up to 30 % of the grafts are occluded. Some of these occlusions have no impact to heart function or long-term survival, but some probably will. There are several possible technical problems emerging in CABG. The most commonly used intraoperative method for graft functioning is transit-time flowmetry (TTFM). TTFM measures the mean flow of the bypass graft and calculates an index of the amount of diastolic flow relatively to systolic flow. The reliability of the TTFM is good but there are no standard values and in several patients it might lead to unnecessary graft revision [88].
The direct imaging of coronary arteries includes the location of the coronary arteries and anastomotic evaluation. Intraoperative ICG imaging is especially useful in the location of coronary vessels in coronary artery re-operations, but it would be helpful in any case, when difficulties are encountered in locating a desired vessel. It might also be useful to determine the location of the correct anastomosis site. Fluorescence imaging has inherent limitations of its use, which in cardiac surgery is mainly the low penetration of the light in tissues. This limits the use of the imaging method on the topical parts of the heart. The location of the conduit parts of coronary vessels is situated on top of the heart muscle. This is also the area, where the coronary heart disease is developed and eventually the area, where the anastomoses of the grafts are sutured in CABG operations. This location is optimal for fluorescent imaging. The evaluation of the anastomotic anatomy and function preoperatively is useful. However, direct evaluation of anastomoses requires a high-quality imaging device and maybe even from simultaneous angles to evaluate the technical quality of the anastomoses. This kind of device is not available in the market at the time being. The anastomoses evaluation with the devices in the market is more indirect and is estimated by the filling of the distal vessel.
Clinical studies of intraoperative direct imaging of the grafts by ICG angiography are increasing slowly but steadily. Twenty patients were assessed intraoperatively by ICG angiography by Rubens et al. in one of the earliest clinical studies and one patient was reported to eventually have a graft revision according to the imaging, which resembles 5 % [89]. Taggart et al. reported a study of 213 grafts with a revision rate of 4 grafts (1.9 %) due to findings in ICG angiography [90]. Reuthebuch and colleagues studied use of fluorescence imaging in 107 patients and reported a graft revision rate of 3.7 % [91]. In a larger study, Balacumaraswami et al. assessed 533 conduits in 200 patients. ICG imaging confirmed technical failure in 8 (1.5 %) conduits in 8 (4 %) patients, which eventually lead to graft revision [92]. Takahashi et al. studied 290 grafts, in which four grafts (1.9 %) were revised due to ICG angiographic findings [93]. Desai et al. reported that 348 grafts were studied by intraoperative ICG angiograms [94]. Patients who had their graft revised were 4.2 %. The evidence in these studies is rather constant. ICG imaging leading to graft revisions that would have otherwise gone unrecognized is roughly between 2 and 4 %. These numbers refer practically to the technical failures met in the operations. These graft revisions might have a long-term effect to the patients involved.
ICG imaging offers also a method for indirect measuring of coronary perfusion imaging. It can be assessed during the operation either before the coronary artery grafting or after the coronary artery grafting. Myocardial tissue perfusion is an indirect method to evaluate the coronary flow. Region of interest is the perfusion area of the measured coronary artery [95, 96]. In a study by Ferguson et al. in 2013, 167 patients underwent CABG with traditional angiography-based revascularization [97]. Coronary perfusion imaging was performed both before and after the bypass grafting and 359 grafts (53 % arterial) were assessed. After grafting, 24 % of the arterial and 22 % of the saphenous vein grafts showed no regional myocardial perfusion change in response to bypass grafting. In 165 in situ internal mammary artery grafts, 40 had no change in regional myocardial perfusion, and 32 out of the 40 were estimated to have competitive flow. This study showed that the anatomic evaluation of stenoses might not be enough and these physiological responses are possible to image with fluorescence perfusion method.
Pediatric Cardiac Surgery
In congenital heart surgery, the use of fluorescence imaging is rare. However, there is a slowly increasing amount of papers around the subject. Most of the heart operations in children are corrections within the heart itself, where the low penetration of the near-infrared light naturally inhibits use of fluorescence imaging. However, when there is an increasing tendency to correct the malformations in an early infancy and the structures are small, there certainly is a place for its use especially in certain defects. On the other hand, imaging of vascular structures including coronary arteries is feasible in children.
In a report of Kogon et al., 30 patients were undergoing the following repairs: (1) coronary artery reimplantation, (2) coarctation, (3) palliative shunts, and (4) pulmonary artery reconstruction [98]. After the repairs, imaging of the hearts was performed in the operating room. In these patients, adequate image quality was reached in 18 of 30 (60 %) patients. Image adequacy was highest for Blalock-Taussig shunts (100 %), coarctation repairs (86 %), and coronary reimplantations (66 %). The lowest quality was reached for the hemi-Fontan (0 %), Fontan (40 %), and pulmonary artery reconstructions (33 %). The authors report several reasons for the unsuccessful image quality, including small incision with difficult exposure, the overlying structures on top of the region of interest, indocyanine dosing and extravasation and the low penetration of the prosthetic material (PTFE) used in the corrections. In this study, the imaging leads to no corrections or changes to the planned surgical procedure. There were no adverse events related to the imaging. The authors concluded that indocyanine green fluorescence imaging may provide an important intraoperative imaging modality for congenital heart surgery. The method shows successful intraoperative images of the target area, which correlate to echocardiograms and cardiac cineangiograms.
Peripheral Arteriovenous Surgery
Even though the amount of scientific studies is limited in the area, ICGI has been used in peripheral arteriovenous surgery. Igari et al. propose a method for the quantitative measurement for assessing peripheral perfusion. According to their study comprising of 21 patients with peripheral arterial disease (PAD) the time to half maximum intensity (T 1/2) was the strongest parameter in their parameter set. They conclude that the outcomes of revascularization procedures can be assessed by the value T 1/2 at the distal region of the first metatarsal bone [99, 100]. Also Terasaki et al. used T 1/2 for assessing the severity of ischemia [101]. In addition to intraoperational use in peripheral arteriovenous surgery, ICG has also been used in the laser therapy of telangiectatic leg veins [102].
Liver Surgery
In oncologic liver surgery, ICG has been used in preoperative assessment as described in Chap. 2. Recently, Asian researchers have developed imaging techniques for ICG fluorescence, enabling visualization of bile ducts [103–105] and hepatic cancer during liver surgery [106, 107]. Lim et al. have very recently presented an excellent review of ICG imaging in surgical procedures of liver cancers [108].
The use of ICG fluorescence imaging has been studied in resection of colorectal liver metastases. Van der Vorst et al. concluded that the optimal minimal dose is 10 mg of ICG administered 24 h prior to the resection [49]. Compared to preoperative CT scan, intraoperative ultrasound, and palpation, ICG-based method is able to better found and discern small superficially located metastases [49]. This ability is based on the architecture of ICG fluorescing tissue, where ICG is actually surrounding the metastases and looks like a ring in the side view of the lesion. The reason for this ICG accumulation is unclear. It is assumed that the immature hepatocytes of the tumor have impaired transport of ICG. In any case, the spatial shape of the fluorescence is a clear indication of metastases and could be used for further image processing to increase the sensitivity of this approach.
Tanaka et al. compared the ability of ICG fluorescence navigation in detection of liver malignancies between cirrhotic and non-cirrhotic livers [109]. According to their study, ICG imaging was significantly better in detection of malignant liver tumors in non-cirrhotic livers. In any case, the positive prediction value of ICG fluorescence was small for pathologically diagnosed tumors. Obviously ICG is quite good in detection of near surface located and large enough tumors, but it seems that more advanced imaging is needed for further tumor classification.
Yokoyama et al. have used ICG imaging for detection of hepatic micrometastases from pancreatic cancer using the method developed by Ishizawa et al. [106] and Aoki et al. [110] for identifying small hepatic tumors during surgery [111]. In this method, the intravenous ICG injection is done on the day before surgery. Some of the ICG leaks from metastases thus revealing them while the ICG in blood circulation has already been excreted out of the body. Similarly, Ishizuka et al. have successfully detected small liver metastasis from colorectal cancer [112].
Imaging Engineering
The basic ICG fluorescence imaging system is small and easy to construct [113]. Replacing a standard computer monitor by goggles may provide a really useful aid for clinical work [114, 115]. Another display mode is to project the fluorescence image on the patient to aid navigation [116]. Optical devices already used in surgery such as endoscopes provide a tempting framework for further ICG imaging enhancements [117]. ICG can also be used as fiducial marker for aiding navigation in endoscopic or laparoscopic operations [118].
Towle et al. have compared ICG and laser speckle contrast imaging (LSCI) for the assessment of vasculature perfusion using a rodent model. Their conclusion is that the methods complement each other and LSCI provides accurate and continuous measurement of blood flow without the need of an external contrast agent. Both methods used same laser diode at 785 nm [119]. In some cases ICG imaging can compete or even supercede traditional imaging like CT as Ohba et al. have shown for intra-arterial chemotherapy for oral cancer, where ICGA was used to find the tumor supplying arteries [120, 121].
Resolution is not considerably reduced with the longer than visible light wavelength used in ICG fluorescence imaging. Therefore, microscopy or microendoscopy is possible with ICG up to resolutions that are in theory about half of that of traditional visual microscopy [122, 123]. Actually even super-resolution microscopy is possible with ICG as with other fluorescence dyes [124]. On the other extreme case, even whole-body ICG fluorescence imaging has been demonstrated in humans [125].
Lasers having wavelength around 800 nm can be used as monochromatic light sources in ICG fluorescence imaging. Another use of lasers with ICG is more active: radiation absorption can be used, e.g. in vascular repair. Using a rabbit model, Esposito et al. have shown how to repair blood veins by ICG-infused chitosan patches using laser tissue soldering. The laser was a AlGaAs diode laser having 810 nm wavelength [126]. Similar diode lasers have been used for ICG-assisted photothermal/photodynamic fat cell destruction [127–130]. The data obtained are useful for safe layer-by-layer dosimetry of laser illumination of ICG-stained adipose tissue for treatment of obesity and cellulite and support the hypothesis that photodynamic and photothermal effects induce fat cell lipolysis during tenths of minutes after treatment. Klein et al. have tested ICG in the laser therapy of cutaneous capillary malformations. The rapid clearance of ICG and other difficulties such as having a proper pulse length in controlling the laser-induced coagulation process of blood veins still remains to be solved in order to make this method more competitive with the alternative therapies. The diode laser had 808 nm wavelength [131].
A multichannel time-resolved optical monitoring system for measurements of diffuse reflectance allows for assessment of inflow and washout of the ICG in human brain tissue up to very large depths corresponding to source-detector separation up to 9 cm at intravenous dye injection [132]. Photoacoustic (PA) in vivo imaging of dynamic biological processes at the molecular level resulting from selective absorption of light at multiple wavelengths can now “hear color” including ICG dye kinetics in three dimensions from deep living tissues at high spatial resolution and in real time [133, 134].
Robotic-Assisted Surgery
The lowest threshold to introduce ICG based imaging is in areas of surgery that already routinely use optical devices such as endoscopes, laparoscopes, and microscopes. Integration of these devices with optics needed for ICG imaging is rather easy both technically and economically. Therefore, one of the most natural pioneering areas of ICG fluorescence imaging is robotic-assisted surgery. In addition, this area provides the unique potential opportunity for the development of applications of image data, i.e. machine vision for the automation of routine procedures.
A review of early works (2011–2012) on ICG imaging in robotic-assisted surgery is given by Marano et al. They cite six works using ICG imaging with robotic-assisted surgery. Since then over 40 papers have been published in about 1 year. Thus we can safely say that the interest of using ICG imaging in medical robotic is increasing quickly [135]. Here, we will briefly review some of the most recent and interesting contribution in this field.
Lee et al. have successfully used ICG for the localization of ureteral stenosis for seven patients during robot-assisted ureteroureterostomy [136]. They conclude that ICG administration aids in discerning healthy ureter from diseased tissue.
Manny et al. used ICG fluorescent imaging for robotic partial adrelectomy with good results [137]. They also used ICG to mark prostate tissue and sentinel lymphatic drainage by percutaneous, robot-guided injection. Their conclusion based on a population of 50 patients for radical prostatectomy is that ICG is safe, feasible, and reliable dye for prostate tissue and sentinel lymphatic drainage marking in majority of patients [138].
Harke et al., Borofsky et al., and Bjurlin et al. have used ICGA for aiding the robot-assisted partial nephrectomy, where ICGA helps to clamp only the tumor feeding vascular branches, while preserving the vasculature feeding the healthy kidney tissue [139–141].
Jafari et al. and Hellan et al. have used ICGA to assess optimal spatial perfusion for robot-assisted rectal surgery to reduce the risk of postoperative anastomotic leak. They conclude that ICGA may play a role in anastomotic tissue perfusion assessment and affect the anastomotic leak rate. However, larger prospective studies are needed to further validate this ICGA use [142, 143].
Angell et al. proposed an ICG fluorescence calibration method based on two doses. The first dose is small and used to estimate the optimal dose for tumor location in robot-assisted partial nephrectomy. This type of calibration is worth considering also in many other as well roboticed as manual operations [144].
Wagner et al. have used ICG to identify the contralateral pericardiophrenic neurovascular bundle and further the contralateral phrenic nerve in unilateral robotic thymectomy in order to achieve maximal thymic tissue resection. No injuries to the phrenic nerve occurred in the population of ten patients. They conclude that ICG has the potential to maximize thymic tissue resection, while reducing operative time and nerve injury [145].
Spinoglio et al. have used ICG for cholangiography in robotic cholecystectomy to prevent accidental intraoperational bile duct injury [146].
Rossi et al. have studied the optimal injection site (cervical or hysteroscopic) for detection of sentinel nodes for endometrical cancer. They found cervical injection to be better [64].
Imaging Development
Projective Display
The use of monitors for viewing ICG images and videos in intraoperative work is problematic in practice, because doctors need to mentally compare and fuse the patient and the displayed image, in addition to the obvious ergonomical problems. These can be solved by projector-based system that overlay the ICG image onto a patient. By using this projecting system, medical doctors can observe the ICG fluorescent area directly on the target tissue.
The existing special ICG cameras tend to be rather expensive. In order to make the system’s cost lower, the signal is captured by using a normal camera, which is sensitive to infrared light while the signal is enhanced by controlling the excitation light and some image processing.
The structure of the ICG image projecting system is shown in Fig. 4.2. The target surface is illuminated by excitation light using infrared LEDs, and then the fluorescent image is captured by a conventional digital camera which is sensitive to infrared. The system has a demountable filter in front of the camera lens, which cuts the visible light. The excitation light can be switched from a PC through USB interface and the successive images captured by the camera are transferred to the PC. In order to display the captured fluorescent image overlaying onto the target surface, a small size projector is employed. After the camera and projector are geometrically calibrated, the captured image can be modified to registered projection onto the target surface (Fig. 4.2).
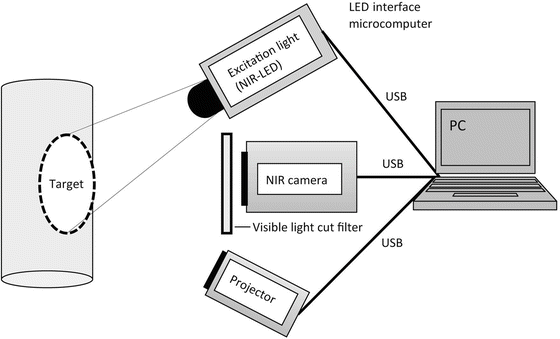
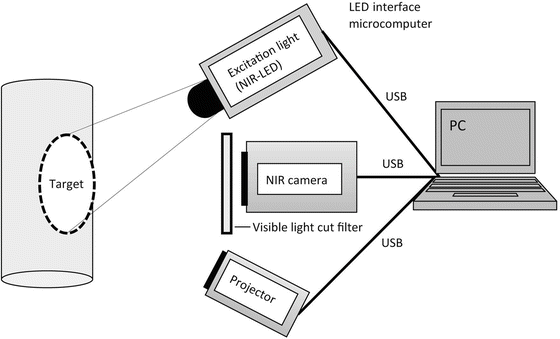
Fig. 4.2
The structure of the ICG image projecting system. The target surface is illuminated by infrared LEDs which can be switched on from the PC. The fluorescent image is captured by a personal-use camera sensitive to infrared. A near-infrared (NIR) filter is set in front of the camera lens
Generally, when the ICG fluorescent area is captured by a normal sensitivity camera, the signal is too weak to be detected clearly. Therefore, a method based on light switching and image processing is used. The data flow of the ICG image projecting system is shown in Fig. 4.3. First, a set of the excited and unexcited images is taken serially. The infrared LED as the excitation light is switched on by the PC, and the camera takes two images, P t with infrared excitation illumination on and
after turning it off. The switching of LED and the shutter of the camera are synchronized. The frame rate of the camera is 15 frames per second. Here t denotes the time index corresponding to shutter timing. Next, by subtracting the unexcited image
from the excited image P t , a differential image D t is calculated. Then by thresholding the differential image D t , a binary differential image B t is derived. Since B t is got by subtracting two temporally successive images, some artifacts may also appear. In order to remove these artifacts, five temporally successive images from
to B t are used. By taking the product of these five images, an enhanced image E t is obtained. By using this method, most of the random noise can also be removed. To further reduce noise, a 3 × 3 median filter is applied to the enhanced image E t and then the final image R t is obtained.
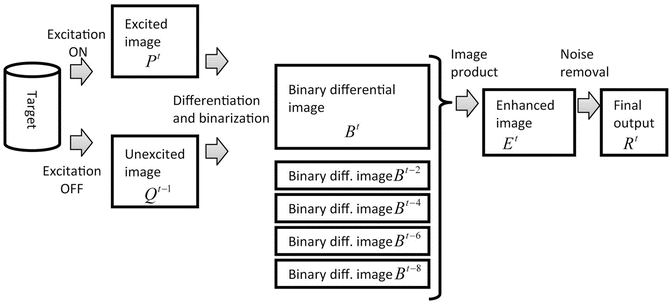



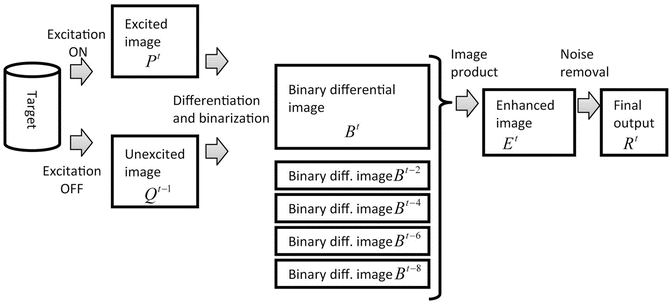
Fig. 4.3
Image data flow of the ICG image projecting system. First, a set of the excited and unexcited images is taken serially, then a binary differential image is calculated. An enhanced image is obtained by taking an image product, then the final output is got by applying noise removal
By conducting a porcine experiment, the feasibility of the ICG image projecting system was confirmed (Fig. 4.4). Since this system assumes that the geometry between the system and the target is static, an automatic update of the calibration as a background task need to be developed in order to make this system suitable for practical clinical work.
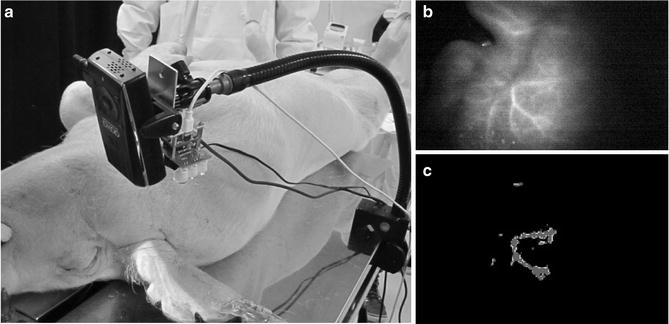
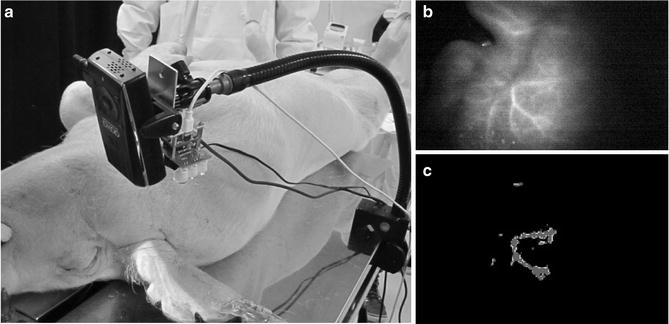
Fig. 4.4
(a) Geometrical setup of animal experiment. The feasibility of the ICG image projecting system was validated by conducting porcine experiments. The detection capacity and the feasibility of the system were evaluated in a practical clinical environment. ICG solution was injected into the blood vessel of the porcine ear, then images were captured by the ICG image projecting system. The primary vessels running around porcine ear can be seen clearly. (b) Original image taken by the near-infrared camera. (c) Resultant image of the ICG fluorescent area detection
Indocyanine Green with Nanostructures
There have been several attempts to chemically modify ICG molecules to further improve the imaging quality and specificity. Unfortunately, that approach has not yet offered clinically potential dyes. An alternative way to modify ICG as a dye is to attach it non-covalently to other molecules and nano structures. For example, Kraft and Ho have very recently combined ICG with liposomes with good results with respect to both quantum efficiency and stability [147]. In addition, liposomes offer a further option for better targeting [148]. Toyota et al. have used liposomally formulated ICG derivatives for lymph node imaging in a mice model [149].
ICG can be combined with phospholipid-polyethylene glycol (PL-PEG) (ICG-PL-PEG) by utilizing non-covalent self-assembly chemistry [150, 151]. The dual functionality of ICG-PL-PEG for targeted optical imaging and photothermal therapy of cancer cells was successfully demonstrated. At conjugation with other therapeutic and imaging agents, this technology is prospective for multifunctional probing at cancer diagnosis and treatment. These nanoparticles were also shown to be good contrast agents for photoacoustic (PA) imaging [152] and as agents providing high selectivity of the PA destruction of cancer cells [153].
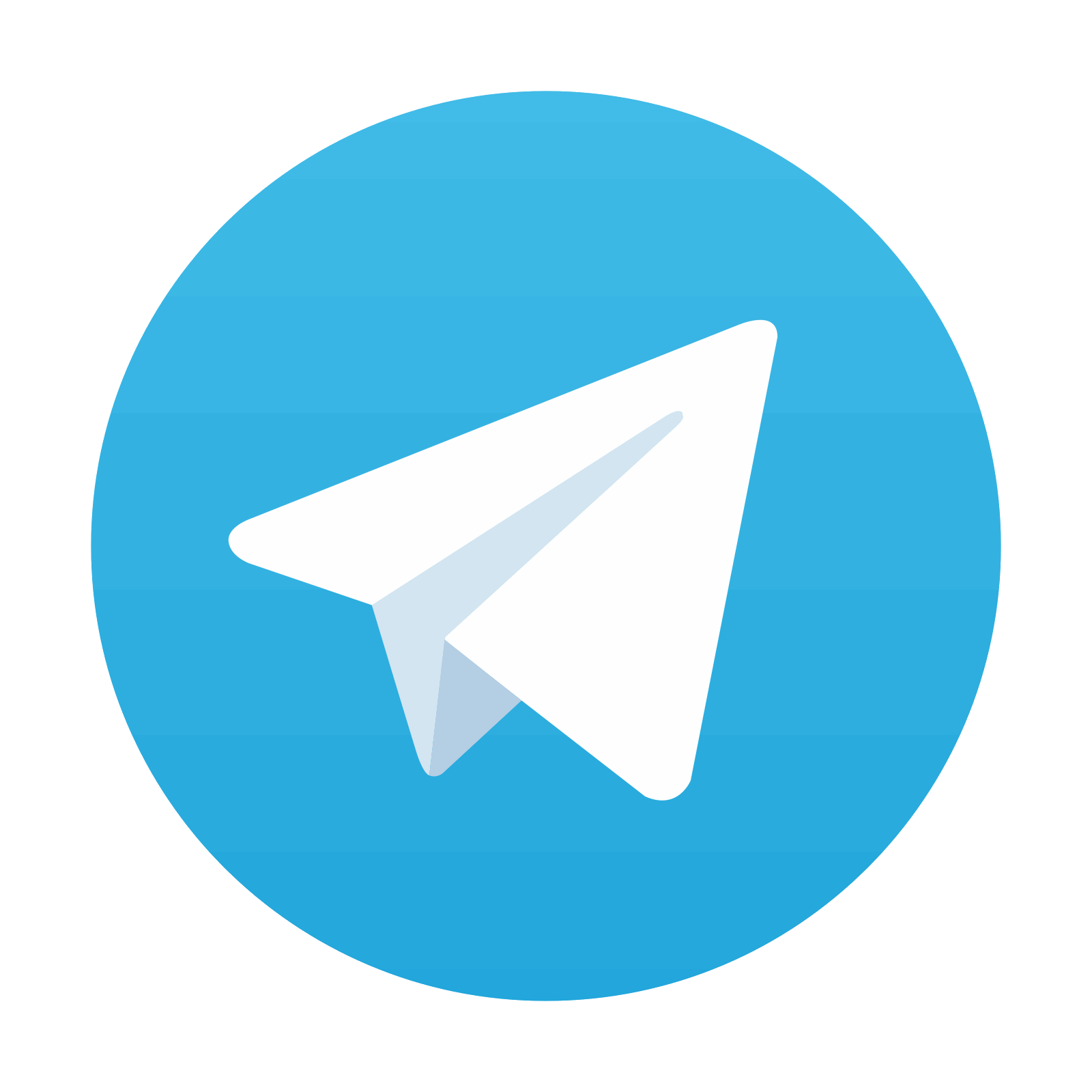
Stay updated, free articles. Join our Telegram channel

Full access? Get Clinical Tree
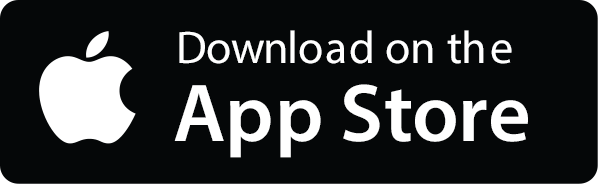
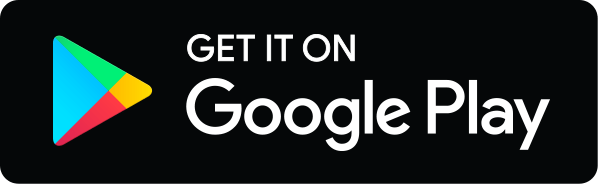