Infectious agents
Virus
Herpes, Epstein-Barr, retrovirus, Coxsackie B virus, cytomegalovirus
Bacteria
Borrelia burgdorferi, Propionibacterium acnes, Mycoplasma
Mycobacteria
Mycobacterium tuberculosis
Organic compounds
Clay, pine tree pollen, combustion products
Inorganic compounds
Aluminum, talc, titanium, zirconium
4.2.2 Clinical Presentation and Treatment
Presenting symptoms of sarcoidosis typically include dyspnea and cough due to lung involvement as well as systemic symptoms including fatigue, weight loss, and fever. However, sarcoidosis can affect virtually any organ of the human body and can present with a wide variety of symptoms depending on organ involvement (Table 4.2).
Table 4.2
Symptoms and signs of sarcoidosis depending on organ involvement
Clinical presentation | |
---|---|
Constitutional | Fever fatigue Weight loss Low-grade fever |
Lungs and upper respiratory tract | Dyspnea Cough Dysphagia Chest pain |
Lymphatic system | Enlarged lymph nodes |
Heart | Palpitation Syncope Dizziness Chest pain |
Liver | Abnormal liver function tests Hepatomegaly |
Spleen | Splenomegaly |
Skin | Papular sarcoidosis Erythema nodosum Lupus pernio |
Eyes | Uveitis Retinal vasculitis |
Central nervous system | Cranial mononeuropathies Neuroendocrine dysfunction |
Musculoskeletal | Acute polyarthritis Chronic arthritis Diffuse myositis |
Salivary glands | Painless swelling |
Most patients with sarcoidosis present with mild symptoms and will recover spontaneously. Decision to initiate treatment should be based on risk-benefit analysis [14]. The mainstay of sarcoidosis treatment is corticosteroids. Current guidelines recommend the use of 20–40 mg of oral prednisone per day [2], and response to therapy should be assessed within 1–3 months. In case of improvement, prednisone treatment can be tapered down to dosage of 5–15 mg per day for an additional 9–12 months, whereas lack of response suggests irreversible fibrotic disease [14]. Given the nonspecific nature of sarcoidosis symptoms, it can be difficult to accurately monitor response to therapy. The use of cytotoxic medication such as methotrexate and immunomodulatory agents such as tumor necrosis factor alpha has been shown to be a viable therapeutic option in patient not responding to corticosteroids or unable to pursue corticosteroid treatment due to adverse effects [15]. Other therapeutic options include hydroxychloroquine to treat patients with skin involvement and hypercalcemia [14].
4.2.3 Diagnosis of Sarcoidosis
The diagnosis of systemic sarcoidosis is established when there is radiographic evidence of sarcoidosis with compatible clinical features and noncaseating granulomas on biopsy with other causes of granulomas ruled out. Response to corticosteroids is not enough to establish diagnosis of sarcoidosis [14]. There are no specific serum markers for sarcoidosis. C reactive protein (CRP) and erythrocyte sedimentation rate (ESR) are sensitive inflammation markers but are not specific for sarcoidosis. Serum angiotensin-converting enzyme (ACE) levels are elevated in approximately 60 % of patients with sarcoidosis due to production of ACE by the granulomas [14]. However, serum ACE is neither sensitive nor specific enough to screen patients with suspected sarcoidosis with its positive predictive value of 84 % and negative predictive value of 74 % [16]. Furthermore, serum ACE should not be used to diagnose sarcoidosis or guide therapy [14].
Due to lack of useful serum markers, diagnosis of sarcoidosis relies greatly on imaging. Classical features of sarcoidosis on chest radiographs include mediastinal and hilar lymphadenopathy, parenchymal opacities, and parenchymal fibrosis in more advanced cases [17]. A staging based on chest radiography has been described more than 40 years ago to monitor chest disease in patient with sarcoidosis (Fig. 4.1) [18]. Although this scale shows poor interobserver agreement, especially for advanced disease, it remains widely used due to its prognostic value [19].
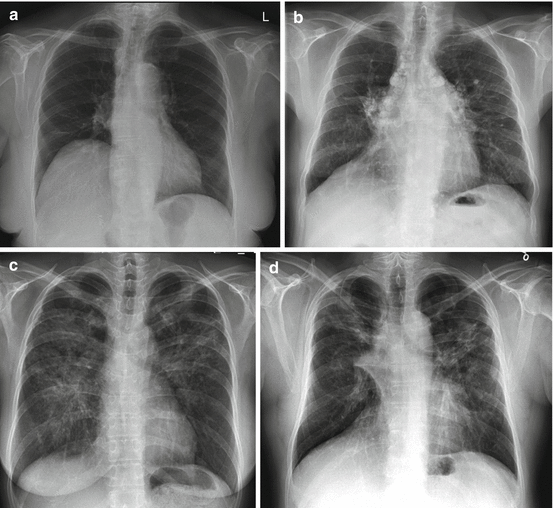
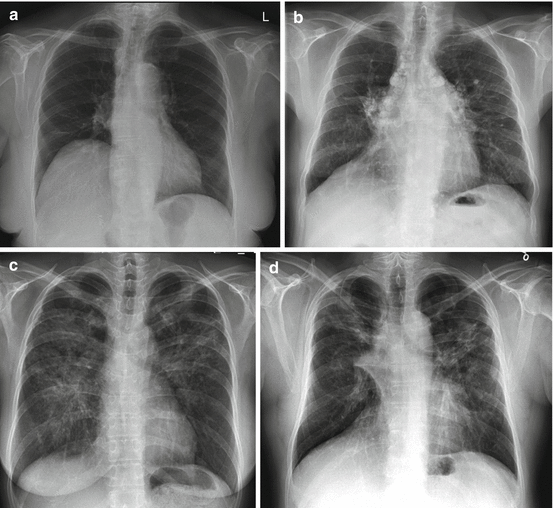
Fig. 4.1
Four stages of sarcoidosis on chest radiographs. (a) Stage I: hilar or mediastinal lymphadenopathy. (b) Stage II: lymphadenopathy and parenchymal disease. (c) Stage III: parenchymal disease alone. (d) Stage IV: pulmonary fibrosis
Computed tomography (CT) imaging is only indicated when chest radiograph is atypical for sarcoidosis or when patients present with hemoptysis. Lymphadenopathy and parenchymal disease are more readily seen on CT. Classically, parenchymal lesions present as small pulmonary nodules in a peribronchovascular distribution or following the fissures [17]. Lymphadenopathy with coarse calcification or “icing sugar” lymphadenopathies are frequently described in sarcoidosis. In more advanced disease, lung fibrosis is seen.
Gallium-67 citrate has been used for the diagnosis, staging, and treatment follow-up of patients with sarcoidosis. Classical findings include the so-called lambda sign, arising from uptake in the mediastinal and hilar lymphadenopathy, and the panda sign, from abnormal parotid and lacrimal gland uptake (Fig. 4.2). However, accuracy of gallium scintigraphy varies greatly from one study to another with reported sensitivity ranging from 60 to 90 % and suboptimal interobserver variability [20–25].
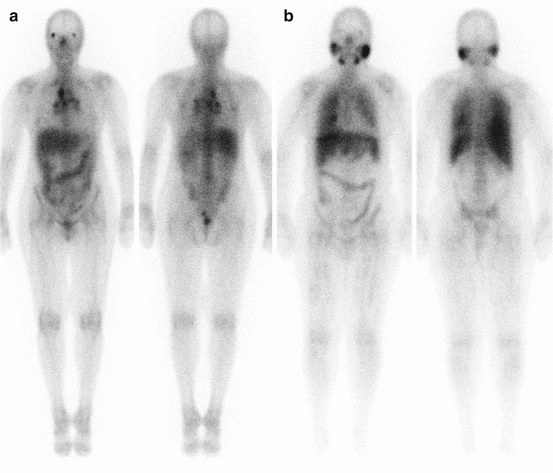
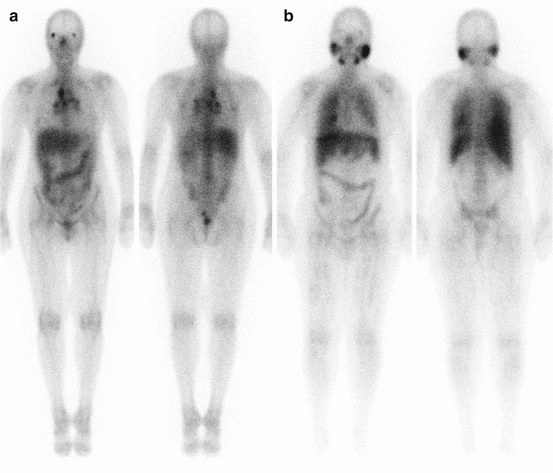
Fig. 4.2
Gallium-67 scintigraphy of two different patients with sarcoidosis. (a) Increased activity in the mediastinal and hilar lymph nodes gives the characteristic lambda sign. (b) Uptake in the parotid and submandibular salivary glands as well as diffuse lung uptake due to sarcoidosis
4.2.4 Role of PET/CT in Sarcoidosis
18F-fluorodeoxyglucose (FDG) is a diagnostic PET radiopharmaceutical with half-life of 109.7 min. FDG is a glucose analog that is transported from the blood into the cells by glucose transporters GLUT 1 and GLUT 4. Once intracellular, FDG is then phosphorylated by hexokinase to form FDG-6-phosphate [26]. Unlike glucose, FDG-6-phosphate is not further metabolized, which leads to trapping of the radiopharmaceutical into the cells (Fig. 4.3). Uptake of FDG is proportional to glucose metabolism, and therefore, increased FDG uptake is seen in several conditions including infection, inflammation, and malignancies.
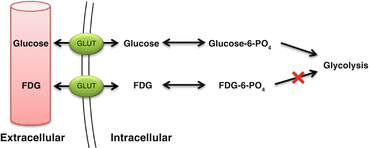
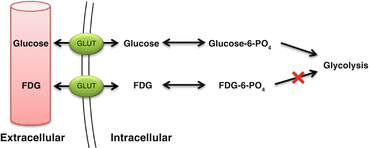
Fig. 4.3
Mechanism of uptake and trapping of FDG
FDG-PET has several practical and technical advantages over 67Ga, including favorable tracer kinetics, lower radiation exposure, and better quality images [27]. FDG-PET is more accurate and allows better evaluation of extrapulmonary involvement compared to 67Ga [25, 28].
4.2.4.1 Evaluation of Disease Extent
FDG-PET imaging permits whole-body assessment in a single-step examination. A large proportion of patients with suspected pulmonary sarcoidosis have extrapulmonary involvement visualized on FDG-PET [29]. Frequent extrapulmonary sites include the bone, spleen, liver, and abdominal lymph nodes [30]. Accurate determination of the spread of active disease with FDG-PET provides an explanation for persistent disabling symptoms and has been shown to influence patient management [29, 31]. Moreover, identification of sites of extrapulmonary sarcoidosis can identify potential biopsy sites (Fig. 4.4) [29].
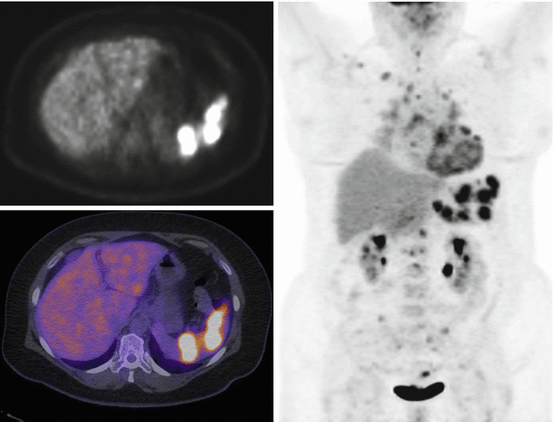
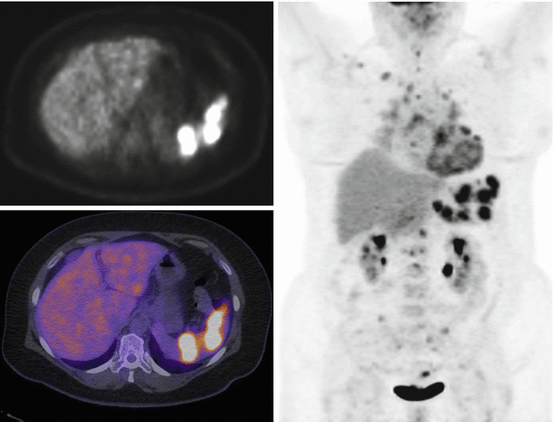
Fig. 4.4
Whole-body FDG-PET scan with selected axial slice in a patient with known thoracic sarcoidosis demonstrating abnormal uptake corresponding to sarcoidosis involvement of the spleen, thoracolumbar spine, and heart as well as in pelvic lymph nodes
4.2.4.2 FDG-PET as a Marker of Disease Activity and in Assessment of Therapy Response
The goal of treatment in sarcoidosis is to treat reversible granulomatous inflammation. Still, there is currently no gold standard method for monitoring inflammatory activity in patients with sarcoidosis. Because of the serious side effects associated with sarcoidosis therapy, it is important to differentiate active and treatable inflammation from quiescent fibrosis. Sarcoidosis often presents with nonspecific systemic symptoms such as fatigue. Monitoring of these nonspecific symptoms does not allow to assess disease progression or response to therapy [32]. Besides, some patients are completely asymptomatic. Serum ACE is also used to monitor response to treatment but does not accurately reflect inflammatory activity [33]. Changes on conventional anatomical imaging, including x-ray and high-resolution CT, are not reliable markers of active inflammation, as they can remain positive even without active disease [34]. On the contrary, PET was shown to provide additional value to assess inflammatory activity in patients with pulmonary fibrosis [35]. Bronchoalveolar lavage (BAL) is an important tool in diagnosis of pulmonary sarcoidosis, but is not recommended for assessment of treatment response [36]. The prognostic value of BAL depends on the number of T lymphocytes, neutrophils, and mast cells [37–39]. Although gallium-67 has been used to detect active granulomas, it lacks correlation with BAL lymphocyte counts [23, 40]. Because FDG accumulates in inflammatory cells and allows imaging of active inflammation, FDG-PET could be used to monitor response to therapy (Figs. 4.4 and 4.5) [41]. FDG-PET has been shown to be a useful adjunct to other diagnostic methods for detecting active inflammation, especially in patients with persistent symptoms and normal ACE levels [31]. FDG-PET is also used to monitor response to therapy. So far, only few studies, most retrospective and with a small sample size, have looked at FDG-PET before and after corticosteroid therapy [20, 42–45] and TNF-α inhibitor therapy [46, 47]. FDG-PET post therapy was shown to correlate with symptoms [44, 46] as well as corticosteroid dosage [42]. It also correlates with endoscopic findings in patients with sinonasal sarcoidosis [45]. On the other hand, FDG-PET does not correlate with serum ACE, serum IL-2R, or pulmonary function tests (PFT) [20, 44, 46, 47]. These findings suggest that FDG-PET could provide useful information to assess response to treatment and adjust steroid dosage.
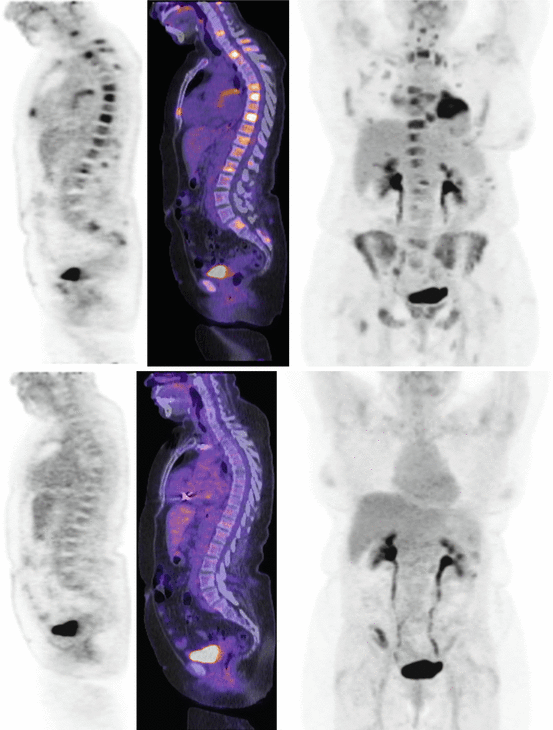
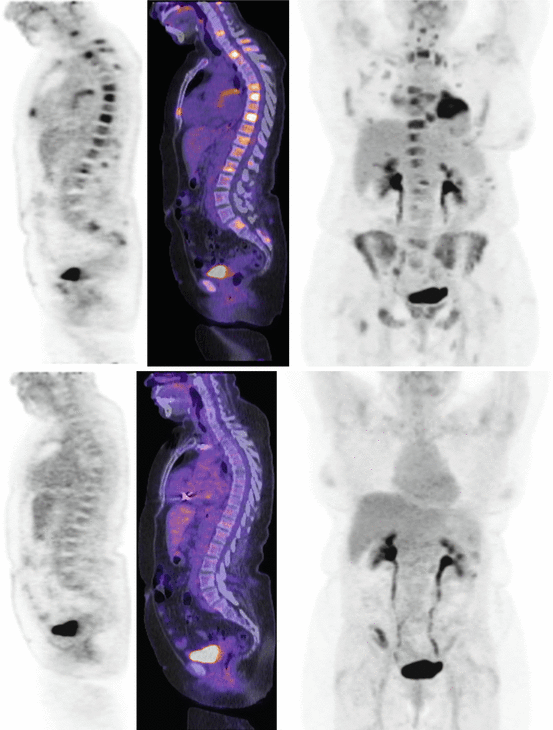
Fig. 4.5
Whole-body FDG-PET scan with selected sagittal slice and maximum intensity projection (MIP) of a patient with extensive sarcoidosis in the heart and bone. Follow-up scan after corticosteroid therapy demonstrates normal biodistribution of FDG, consistent with response to treatment
4.3 Cardiac Sarcoidosis
4.3.1 Epidemiology
In the United States, myocardial involvement is recognized in approximately 25 % of patients with sarcoidosis [48]. In the Japanese population, the prevalence of cardiac involvement in patients with sarcoidosis is significantly greater, reaching 58 % [49–51]. Cardiac sarcoidosis is characterized by noncaseating granulomas in the myocardium surrounded by lymphocytes [52]. The left ventricular free wall and septum are the most frequently affected myocardial regions [49, 52].
4.3.2 Clinical Presentation and Treatment
Patients with cardiac sarcoidosis may have different presentations depending on the extension and location of the disease [14]. They can present with conduction abnormalities due to direct infiltration of inflammatory cell and/or scar formation. These can also manifest as atrioventricular block or bundle branch block [49]. Atrioventricular block affects approximately 25 % of patients with cardiac sarcoidosis and is caused by inflammation or scar in the basal septum [53, 54]. Diagnosis of cardiac sarcoidosis should be considered in young patients with atrioventricular heart block of unknown etiology [54, 55]. Patients with cardiac sarcoidosis can also present with congestive heart failure due to extensive infiltration of the myocardium by granulomas [49, 56].
As with pulmonary sarcoidosis, cardiac inflammation from sarcoidosis is typically treated with corticosteroids. Several uncontrolled series and small sample retrospective studies suggest that steroids may be valuable to treat cardiac sarcoidosis [53, 54, 57–59]. Thus far, there are no randomized controlled trials available to confirm these results and treatment of cardiac sarcoidosis with corticosteroids remains controversial [60]. Initiation of corticosteroid therapy, even with antiarrhythmic drugs, does not prevent arrhythmic event [61]. Pacemakers and ICD are often required when there is extensive involvement of the conduction system or when there is significant heart failure [60]. A systematic review of corticosteroid therapy in cardiac sarcoidosis revealed a wide range of steroid regimens, with prednisone dosage of 20–60 mg daily or 50–60 mg on alternate day [62]. In small single-center retrospective studies, corticosteroid therapy was associated with maintenance of LV function in patients with normal function at diagnosis and improvement of ejection fraction in patients with mild to moderate LV dysfunction [58, 59, 63]. However, no improvement was observed in patients with severe LV dysfunction. As regards to ventricular arrhythmias, some studies showed benefits of immunosuppression therapy [59, 64, 65], whereas others showed no benefit or worsening [61, 66]. As for LV dysfunction, immunosuppressive therapy appears more beneficial in early phase of disease [58, 59]. There is, however, currently not enough data to establish mortality benefit from steroid therapy [62].
4.3.3 PET Imaging
4.3.3.1 Patient Preparation
The myocardium can metabolize different substrates, including free fatty acids, glucose, pyruvate, and ketone bodies. Under physiological conditions, the heart metabolizes mainly free fatty acids and glucose, with free fatty acids accounting for 60–90 % of the energy substrates [67–69]. The source of energy used by the myocardium depends mainly on free fatty acids and glucose plasma concentration and insulin levels [70]. In order to obtain diagnostic FDG-PET images for assessing active cardiac sarcoidosis, pretest preparation is required to suppress physiological uptake in the normal myocardium. Adequate suppression of physiological myocardial FDG uptake is crucial to maximize diagnostic accuracy (Fig. 4.6).
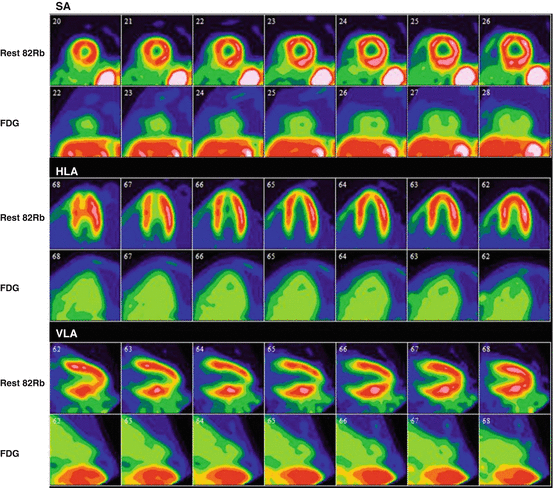
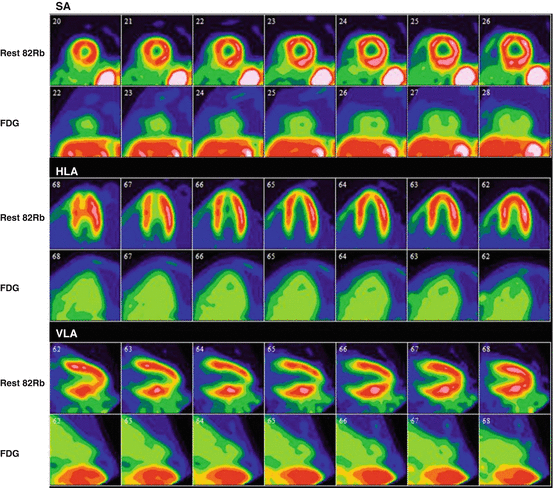
Fig. 4.6
Rest rubidium-82 and FDG-PET scan of a patient with suspected cardiac sarcoidosis. After adequate suppression of physiological myocardial uptake, there is no significant FDG uptake in the myocardium with only blood pool activity, representing a negative study for cardiac sarcoidosis
Fasting
When fasting, the myocardial metabolism is shifted and 90 % of its energy comes from metabolism of free fatty acids [71]. For this reason, prolonged fasting is routinely used to suppress physiological myocardial glucose uptake. Several protocols have been described using different fasting time to suppress physiological myocardial FDG uptake, typically between 5 and 18 h [72–75]. To maximize specificity of myocardial FDG uptake, current guidelines recommend fasting for at least 12 h [76].
Diet
Twelve-hour fasting alone can lead to variable myocardial FDG uptake and additional measures are important to consider to minimize physiological uptake [76]. Changing diet prior to imaging has been shown to be at least as effective as fasting to suppress physiological myocardial uptake [72]. Indeed, the fatty acid-glucose cycle, known as the Randle cycle, indicates that glucose loading suppresses fatty acid utilization and that fatty acid loading suppresses glucose utilization by the myocardium [77]. Different low-carbohydrate diets and low-carbohydrate high-fat (LCHF) diets have been used to minimize myocardial FDG uptake (Table 4.3). In a small randomized study, Cheng et al. showed that a simple low-carbohydrate diet in combination with prolonged fasting of 6 h provided adequate myocardial suppression of FDG uptake, while addition of fatty acid loading did not, suggesting that low carbohydrate might be preferable [78]. Others suggested that high-fat meal 3–6 h or 1 h prior to imaging provided adequate myocardial suppression [79]. Harisankar et al. demonstrated that LCHF diet is superior to prolonged fasting of 12 h to suppress myocardial FDG uptake [80]. More recently, Soussan et al. demonstrated that a LCHF diet for the dinner and breakfast preceding imaging followed by 4 h fasting provided sufficient myocardial suppression of uptake for the diagnosis of cardiac sarcoidosis [81]. There is currently no evidence that clearly established usefulness of LCHF diet over low carbohydrate alone. Furthermore, there is no data on improvement of diagnosis of cardiac sarcoidosis using such diets. Nevertheless, because diet modification is an easily applicable measure, it is routine to follow a diet with less than 5 g of carbohydrate, with or without high-fat meal prior to imaging. Table 4.4 shows an example of the LCHF recommendations provided to the patients prior to FDG imaging.
Table 4.3
Review of different patient preparation to minimize physiological myocardial uptake of FDG
Authors | Year | N | Patients | Fasting | Diet | Fat | Carbohydrates |
---|---|---|---|---|---|---|---|
Lum et al. [82] | 2002 | 69 | Cancer staging | Overnight | Low carbohydrate | N/A | N/A |
Williams and Kolodny [79] | 2008 | 161 | Cancer staging | 3–6 h | Very high fat, low carbohydrate, protein permitted | N/A | N/A |
Cheng et al. [78] | 2010 | 63 | Cancer staging | 6 h | Low carbohydrate | N/A | <5 g |
Wykrzykowska et al. [83] | 2009 | 32 | Cancer staging with suspected CAD | Overnight | Very high fat, low carbohydrate, protein permitted | N/A | <5 g |
Harisankar et al. [80] | 2011 | 120 | Coronary artery disease | 12 h | Low carbohydrate, high fat, protein permitted | 20.8 g | 1.2 g |
Coulden et al. [84] | 2012 | 120 | Cancer staging | Overnight | Low carbohydrate | N/A | <3 g |
Kobayashi et al. [85] | 2013 | 14 | Healthy volunteers | 6 h | 24 h low-carbohydrate diet with high-fat meal | 9 g | 1 g |
Soussan et al. [81] | 2013 | 58 | Cardiac sarcoidosis | 4 h | Low-carbohydrate high-fat meal 4 h | N/A | N/A |
Table 4.4
Example of the LCHF recommendations provided to the patients prior to FDG imaging at the University of Ottawa Heart Institute
Do eat | Do not eat |
---|---|
Beverages: Mineral water, seltzer, water, clear liquids without milk or sugar, coffee, tea, and herbal tea (without milk or sugar) Eggs: Fried, scrambled prepared without milk, omelet prepared without milk or vegetables Condiments and seasonings: Anything that does not contain sugar Fats/oils: Animal fats that are part of the meat you eat, butter, margarine Sweeteners: Aspartame, NutraSweet, Sweet‘N Low, Equal Fish: any fish Fatty unsweetened fish (fried or broiled but not grilled) Meats: Fatty unsweetened (fried or broiled but not grilled) red meat, bacon, meat only sausages, bacon, ham Poultry: Fatty unsweetened chicken and turkey (fried or broiled but not grilled) Shellfish: Any non-processed shellfish Low-carbohydrate vegetables: Cucumber, broccoli, lettuce, celery, mushroom, radish, asparagus, green pepper, cabbage, spinach Sweeteners: Aspartame, NutraSweet, Sweet‘N Low, Equal | No sugar: Sugar in any form is strictly forbidden (including natural sugars in fruit) No starches: Pasta, breads, bagels, cereals, crackers, cookies, muffins, rice, potatoes No candy: Candy, chewing gum, mints, cough drops No processed products: Processed foods such as deli meats. These products often contain hidden sugars No Splenda substitutes No milk or milk products No cheese or cheese products No peanut butter or nuts No alcohol No fruit No vegetables with higher carbohydrate content |
Heparin
Nuutila et al. showed that increased free fatty acid serum levels induced by heparin infusion were associated with decreased myocardial FDG uptake in healthy volunteers [86]. The protocol used in their study consisted of two 200 IU boluses of unfractionated heparin, injected intravenously, 90 min before FDG injection and at time of injection, with continuous infusion of 15 IU/min starting 90 min prior to FDG injection. The increased in FFA levels is mediated via activation of the lipoprotein and hepatic lipase [87]. Heparin injection is now routinely done to suppress myocardial FDG uptake, and typical dosage is 50 IU/kg divided in one or two intravenous bolus injections [25, 74, 88, 89], without reports of increased bleeding risk [25, 89]. However, using heparin infusion alone to suppress myocardial uptake is not sufficient since approximately half of the patients still present diffuse myocardial FDG uptake following heparin injection and 6 h fasting [89, 90]. Administration of unfractionated heparin prior to injection of FDG has not been shown to improve detection of cardiac sarcoidosis yet. Nevertheless, many centers have implemented heparin injection in their imaging protocol to maximize diagnostic accuracy.
Diabetic Patients
While many studies on patient preparation prior for FDG imaging exclude patients with diabetes [80, 82, 83, 85], others include only very small number of patients with diabetes [78]. In the small number of studies that do include patients with diabetes, there are discrepancies between the protocols with some suggesting to continue diabetic medication [79], while others are prescribing to hold diabetic medication on the day of the scan [81]. Currently, there are no data addressing specifically the optimal pretest preparation of patients with diabetes prior to FDG-PET in the context of cardiac sarcoidosis.
Different centers have different protocols to image diabetic patients. At the University of Ottawa Heart Institute, diabetic patients are scheduled as the first case of the day, following 12 h fasting and low-carbohydrate high-fat diet, to minimize patient discomfort and glycemic issues. It is recommended that diabetic patients monitor their blood sugar levels following their usual routine with one measurement obtained the morning of the PET scan. If the glycemia falls to less than 4 mmol/L, patients are instructed to drink juice or take glucose to raise their sugar level to higher than 4 mmol/L. Some oral medications should be held on the morning of the scan, while others should be continued (Table 4.5).
Table 4.5
Oral diabetic medication that should be continued or held on the day of PET scan based on the University of Ottawa Heart Institute protocol
Oral medication that should be continued on the day of the PET scan | Oral medication that should be held on the day of the PET scan |
---|---|
Exenatide (Byetta) Linagliptin (Trajenta) Liraglutide (Victoza) Metformin (Glucophage or Glumetza) Pioglitazone (Actos) Rosiglitazone (Avandia) Saxagliptin (Onglyza) Sitagliptin (Januvia) | Acarbose (Glucobay) Gliclazide (Diamicron) Gliclazide MR (Diamicron MR) Glimepiride (Amaryl) Glyburide (Diabeta) Repaglinide (Gluconorm) |
In many cases, usual doses of long-acting and intermediate-acting insulin can be continued the night before the PET scan. Short-acting insulin dosage and premixed insulin may need to be reduced the evening before the scan given the low-carbohydrate diet. Since the patients will be fasting on the day of the test, the usual breakfast dose of short-acting insulin should not be taken. Finally, for patients with type 1 diabetes using an insulin pump, basal insulin should be continued on the day of the test.
4.3.4 Imaging Protocol
Imaging protocol includes three components: (1) cardiac rest perfusion scan, (2) whole-body FDG scan, and (3) dedicated cardiac FDG scan. The imaging protocol used at the University of Ottawa Heart Institute (UOHI) is illustrated in Fig. 4.7.
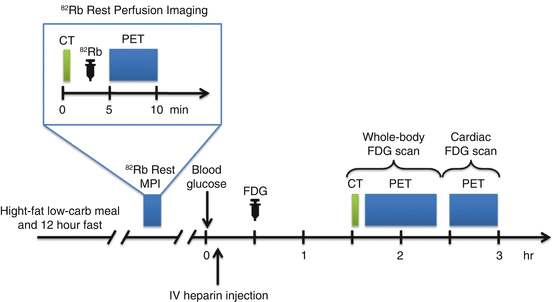
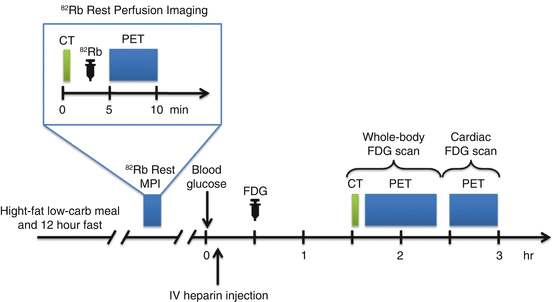
Fig. 4.7
Typical imaging protocol combining rest Rb-82 perfusion imaging and whole-body and dedicated cardiac FDG-PET scan
Cardiac rest perfusion scan is typically performed using PET perfusion tracers such as rubidium-82 or ammonia-N13 and follows regular procedure guidelines for rest myocardial perfusion [91]. With Rb-82, dosages of 10–20 MBq/kg up to 2,200 MBq are used and ECG-gated images are acquired over 4–8 min after injection. Ammonia-N13 rest images are acquired over 15–30 min approximately 5 min after intravenous injection of 5–10 MBq/kg (up to 750 MBq). Images are reconstructed using CT-based attenuation correction and displayed in the usual short and long axes.
Approximately 1 h after intravenous injection of 5 MBq/kg (up to 550 MBq) of 18F-FDG, whole-body PET scan is acquired. The patient is positioned supine with his or her arms up above his head. A low-dose CT for attenuation correction and localization is first acquired. Then, whole-body FDG-PET images are acquired from the base of the skull to mid thighs, 3 min per bed position. Following whole-body imaging, dedicated cardiac PET scan is performed. This acquisition includes a static and, if available, ECG-gated acquisitions. Images are reconstructed by OSEM with attenuation correction and displayed along the perfusion images in the usual short and long axes (Fig. 4.6).
4.3.5 Image Interpretation
Cardiac FDG interpretation must ensure adequate patient preparation. Indeed, poor patient preparation can result in equivocal study due to physiological myocardial FDG uptake. For interpretation, cardiac FDG-PET images are displayed along with rest perfusion images with conventional cardiac display software in the usual short and long axes. Ishimaru et al. described four patterns of FDG uptake: none, focal, focal on diffuse, and diffuse (Fig. 4.8) [89]. This classification has been adopted by other studies and is now frequently used [88, 92]. Focal and focal on diffuse uptake patterns are considered suspicious for cardiac sarcoidosis, whereas diffuse or absence of uptake are considered negative. In order to increase specificity of the diagnostic test, correlation with clinical history is crucial in the interpretation of FDG-PET scan. The interventricular septum is known to be frequently affected in patients with CS and is associated with second- and third-degree atrioventricular block [53, 54]. Therefore, uptake in that region in a patient presenting with atrioventricular block is highly suspicious for sarcoidosis (Fig. 4.9) [93].
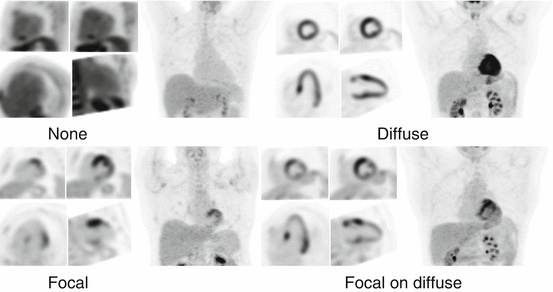
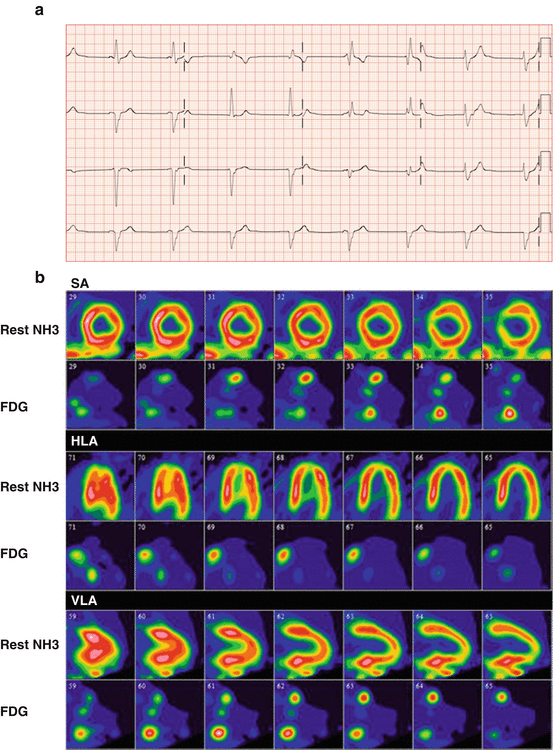
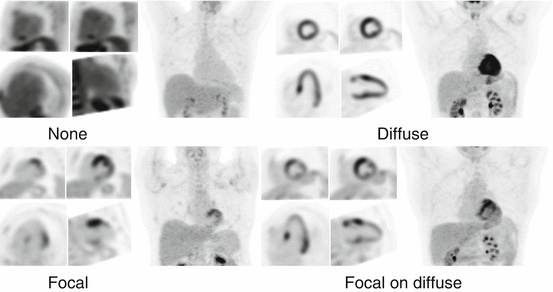
Fig. 4.8
Four different patterns of myocardial FDG uptake: none, diffuse, focal, and focal on diffuse
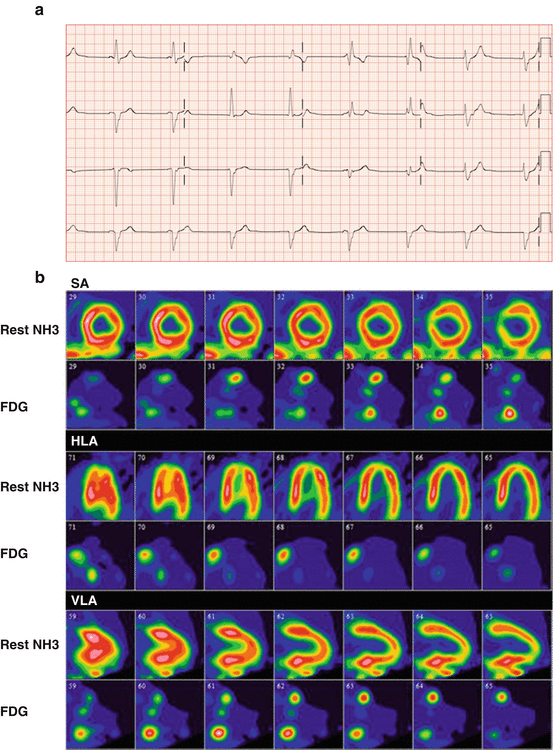
Fig. 4.9
(a) ECG of a patient without known history of sarcoidosis presenting with complete atrioventricular block and junctional escape beat with right bundle branch block. (b) Subsequent FDG-PET/CT with ammonia-N13 rest imaging shows focal increased uptake in the basal septal wall, right ventricular insertion, as well as right ventricular free wall
Similarly, if the onset site of VT demonstrates focal FDG uptake on FDG-PET, the likelihood that the uptake represents active cardiac sarcoidosis is increased [76]. Although not routinely used, quantification of heterogeneity of uptake can be used to increased specificity. Quantification of heterogeneity of uptake using SUV coefficient of variation has been shown to be significantly higher in patients with CS compared to normal healthy subjects and in patients with DCM [92].
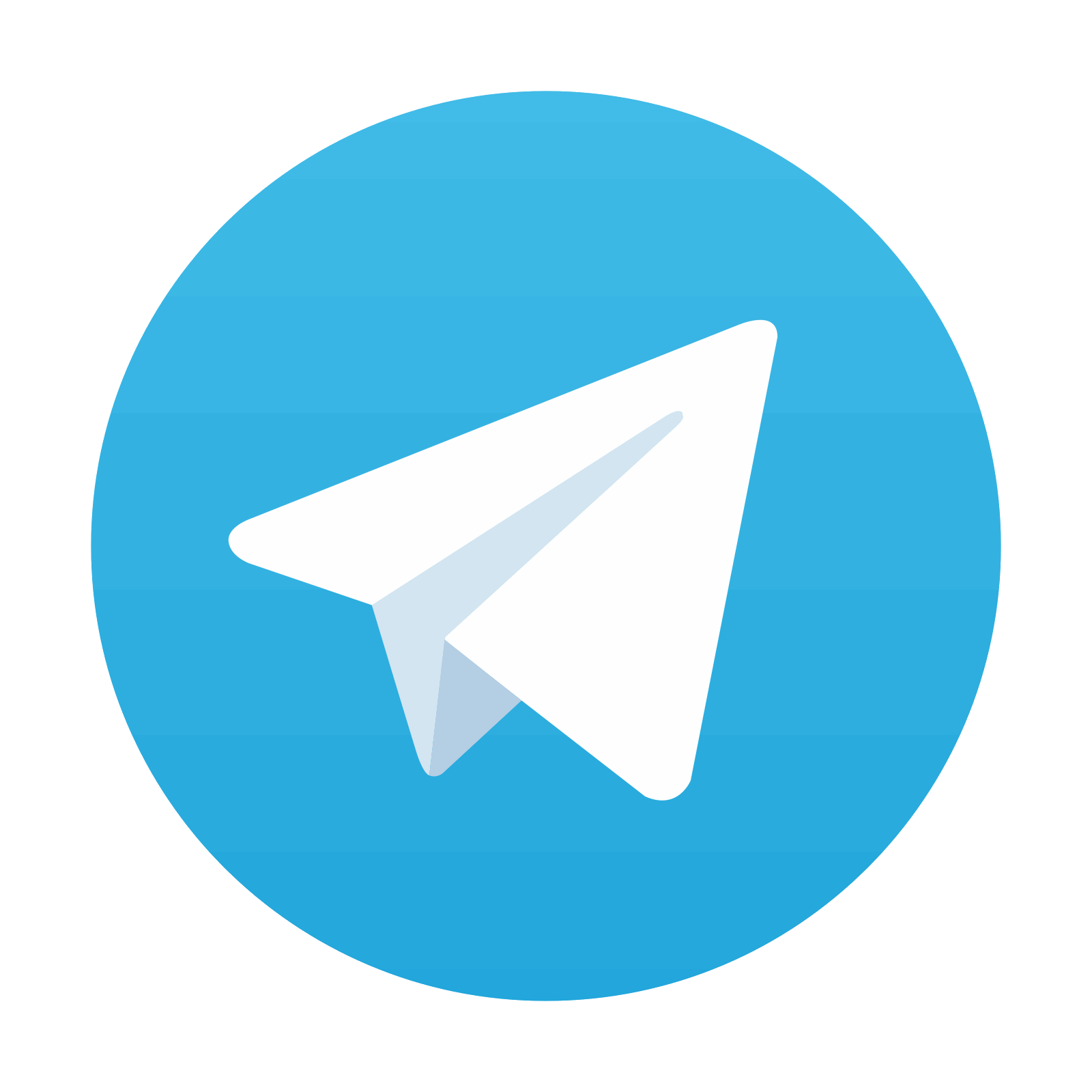
Stay updated, free articles. Join our Telegram channel

Full access? Get Clinical Tree
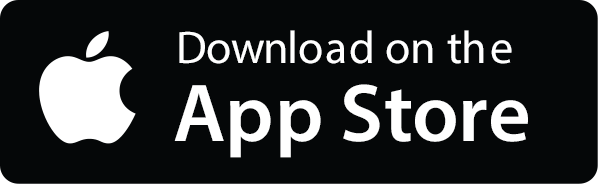
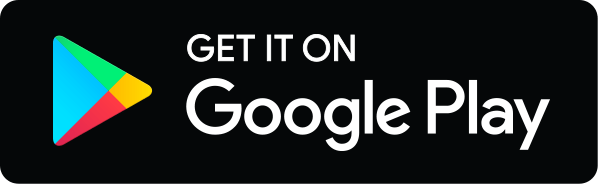