(1)
Department of MRI, Medical Center at Siegerland Airport, Burbach, Germany
Dangerous bioeffects of magnetic resonance imaging can arise from:
1.
Static magnetic fields
2.
Gradient magnetic fields
3.
Radiofrequency fields
4.
Acoustic noise
Potential dangers are:
1.
Magnetic attraction of ferromagnetic objects (missile artifacts)
2.
Magnetic force on body implants (aneurysm clips, pacemaker, intraoperative devices, prosthesis, cardiac valve prosthesis, otologic implants, etc.)
3.
Interaction with cardiac pacemakers, insulin pumps, cerebrospinal fluid valves, etc.
4.
Body heating by gradient field and radiofrequency fields
5.
Exposure to acoustic noise, particularly for psychiatric patients, sedated patients, and neonate patients, which can demonstrate reactions to acoustic noise
Nearly all of the potential dangers mentioned above correlate overproportionally with magnetic field strength of the MR imaging system.
With increasing numbers of diagnostic and interventional MR procedures, there is a critical need for careful investigations concerning acute and cumulative effects of magnetic field exposure on patients and healthcare professionals, to help in establishing guidelines for occupational and patient exposures to static magnetic fields and radiofrequency (Fuentes 2008, DIN EN 60601-2-33).
For X-ray diagnostic imaging, radiation exposure for patients and healthcare professionals has to be reduced to the absolute necessary minimum – it has to be as low as reasonably achievable (“ALARA” principle). A similar principle could be demanded for magnetic resonance imaging (Table 4.1).
Table 4.1
Typical sources of electromagnetic fields
Frequency range | Frequencies | Examples of exposure sources |
---|---|---|
Static | 0 Hz | Video display units; MRI; industrial electrolysis; welding devices |
ELF (extremely low frequencies) | 0–300 Hz | Power lines; domestic distribution lines; domestic appliances; electric engines in cars, trains, and tramways; welding devices |
IF (intermediate frequencies) | 300 Hz–100 kHz | Video display units; antitheft devices and shops; free access control systems, card readers, and metal detectors; MRI; welding devices |
RF (radio frequency) | 100 kHz–300 GHz | Mobile telephones; broadcasting and TV; microwave oven; radar and radio transceivers; portable radios; MRI |
4.1 Static Magnetic Fields
Contemporary MR imaging systems operate at a field strength ranging from 0.2 to 3 T.
For short-term exposure to RF energy, multiple investigations are available including studies on cell alteration and reproduction, teratogenicity, DNA structure and gene expression, blood–brain barrier permeability, nerve activity, cognitive function and behavior, cardiovascular and hematological dynamics, temperature regulation, circadian rhythms, immune responsiveness, visual and auditory functions of the brain, and other biological processes (FDA 2003, Weintraub et al. 2007). Most, but not all, of these studies demonstrated no substantial harm caused by exposure to static magnetic fields (Shellock 2004, 2009; Schwenzer and Bantleon 2007; Von Klitzing 1986, Hong 1989).
Until 2002, MR imaging at field strength larger than 2.5 T was considered to be potentially dangerous (Kuhl et al. 2008).
According to the American Food and Drug Administration (FDA), clinical MRI systems up to 8 T are considered a “nonsignificant risk” for adult patients (Zaremba 2001, Hoult 2011).
Atkinson and coworkers examined the biological effects of strong magnetic at the field strength of 9.4 T (Atkinson et al. 2007).
The increased risk for medical staff exposed to static magnetic fields in MRI units was addressed in 2009 by the Scientific Committee on Emerging and Newly Identified Health Risks (SCENIHR) of the European Commission. A limited use of MRI was discussed. Only by consequent and immediate reaction of the medical scientific societies could this initiative be stopped (European Commission 2009). The present opinion of the SCENIHR is that exposure to electromagnetic fields may increase the risk of adverse health effects. Exposure of patients and medical staff could exceed safety limits for the general public.
Most documented severe injuries and some even fatal accidents occurred in using MR imaging systems when ferromagnetic objects like oxygen tanks, wheelchairs, or medical equipment were brought under the influence of the strong static magnetic field. Even more critical can be ferromagnetic biomedical implants like aneurysm clips. Dislocation of aneurysm clips has already resulted in fatal injuries.
Influence on ferromagnetic objects is called “missile effect” and represents the most important danger for patients in magnetic resonance imaging units. The underlying electric force is proportional to the square of the magnetic field strength. This means a 3 T magnet has a 100 times stronger magnetic attraction force compared to a 0.3 T magnet (Clark 2006).
A big advantage of open low-field MRI is better access to the patient, particularly for traumatized, unconscious, or pediatric patients. Anesthesiologists appreciate the reduced “missile effects” on ferromagnetic objects, which led to hazardous accidents in the past (Capelastegui et al. 2006) (Fig. 4.1).
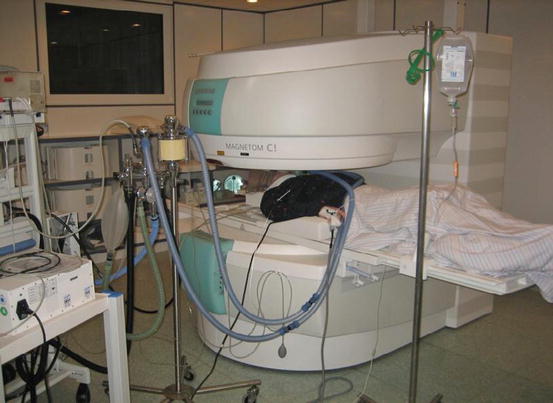
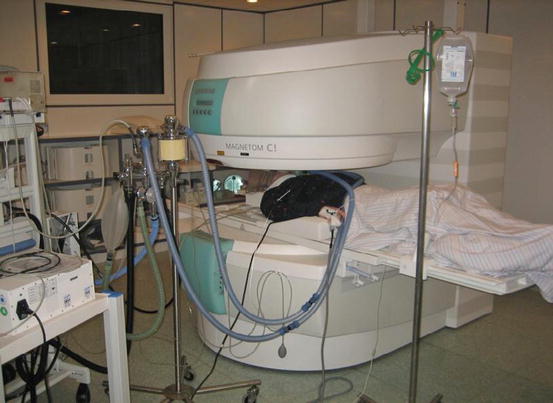
Fig. 4.1
Head examination of a trauma patient. No interaction between anesthesiologist equipment and MRI (Siemens Magnetom C!, 0.35 T)
4.2 Gradient Magnetic Fields
Gradient- or time-varying magnetic fields may induce neuromuscular stimulation by inducing local electric fields (Abart 1997, Cavin 2007). The bioeffects of gradient magnetic fields depend on a variety of factors, including field frequency, magnetic flux density, presence of harmonic frequencies, waveform characteristics and polarity of the signal distribution in the body, electric properties, and sensitivity of the cell membranes (Mansfield 1993).
The US Food and Drug Administration has defined threshold levels for the strength of gradient magnetic fields. These safety standards, combined with contemporary MR technique, are considered adequate for patient protection.
Gradient magnetic fields may stimulate peripheral nerves, producing sensory events experienced as “tingling” or “tapping” (Cohen 1990, De Vocht 2006).
At substantially increased gradient energy level, lying above the perception thresholds, the patient may experience pain. At extremely high levels, cardiac stimulation can be produced; the gradient magnetic field energy necessary for cardiac simulation exceeds the commercially available MR system performance by about one order of magnitude.
Present-day low-field MR systems are equipped with gradient systems, nearly equivalent to high-field installations. The gradient amplitude lies above 23 mT, the rise time is about 400 ms, and the resulting slew rate is about 55 T/m/s (Siemens Healthcare). With increasing gradient performance, neuromuscular stimulation is theoretically possible also in low-field MR systems.
4.3 Radiofrequency Fields
Radiofrequency exposure of the patient is strongly dependent on field strength. Remember our comparison at the beginning: the string tension of a guitar becomes stronger with increasing frequency.
Whereas the SNR increases nearly with field strength, SAR increases exponentially (as a function of the square of field strength) (Bottomley 2008, Quick 2011).
This limits the use of SAR intense imaging sequences, particularly in ultrahigh-field systems (>3T) (Chen 1986, Atalar 2005). If field strength is increased two times, the resulting RF frequency exposure is increased by 22.
That means, if you increase the field strength from 0.3 to 3 T, the resulting radiofrequency exposure of the patient is increased by 102, or 100 times higher. Most of the radiofrequency power transmitted in MR imaging is transformed into heat within the patient’s body as a result of resistive losses (Bottomley 1981, Shellock 1988, 2000, 2009).
Before 1985, when the systems were mostly equipped with permanent magnets with low field strength, there were no reports concerning thermal physiologic problems in MR imaging. With increasing number of clinical high-field systems (equal or larger than 1.5 T), a dosimetric term was defined: the specific absorption rate (SAR). The unit is Watts per kilogram body weight (W/kg). Usually the whole-body averaged SAR is measured by RF energy dosimetry. An RF energy dose of more than 4 W/kg is considered to expose the patient to significant hazard (US Food and Drug Administration, Table 4.2) (Hoult 2000, Schaefer 2001).
Site | Dose | Time (>min) | SAR (W/kg) |
---|---|---|---|
Whole body | Averaged | 15 | 4 |
Head | Averaged | 10 | 3 |
Head or torso | /g of tissue | 5 | 8 |
Extremities | /g of tissue | 5 | 12 |
Particularly for pregnant women, a whole-body averaged RF exposure >2 W/kg for more than 6 min can result in a heating of the fetus >38 °C (Hand et al. 2010).
Thermoregulatory response of the patient to radiofrequency energy exposition is dependent on several physiologic factors and surrounding conditions, like underlying health condition (cardiovascular disease, hypertensive, diabetes, fever, old age, and obesity), the individual thermoregulatory system, duration of exposure, and rate of energy deposition.
Furthermore, several drugs can influence thermophysiologic response, including beta-blockers, diuretics, Ca antagonists, and amphetamines.
In an experimental study, 26 pigs were exposed to different amounts of RF energy in a whole-body birdcage coil at 3T. SAR (specific absorption rate) was below 6 W/kg and exposure time is between 30 and 60 min. Severe thermal damage of body tissues was found, depending on exposure time and applied energy levels. The SAR was found to be unreliable, concerning grade end extension of tissue damage. As an explanation for the surprisingly high RF damage, it was assumed that porcine thermal regulation is different from human physiology (Kobelt 2011).
The potential genotoxicity (DNA damage) of RF radiation is subject of an ongoing discussion (Duan et al. 2015).
All these potential hazardous effects of exposure can be avoided or at least minimized using low-field magnetic resonance imaging.
4.4 Acoustic Noise
Why is MR imaging so loud? We can come back to our initial comparison of magnetic and musical resonance.
Principally, the MR system behaves like a loudspeaker (Counter 1997). The gradient coils and gradient housing of the MRI represent the speaker coil and membrane. Each gradient field-switching procedure in the presence of a strong static magnetic field induces a force in the coil (Lorentz force). The switching frequency represents the frequency of the resulting MR noise (Bowtell and Mansfield 1995).
The intensity of the gradient output influences the level of acoustic noise. Enhancement is achieved by decreasing section thickness, field of view, repetition, and echo time. Presence and size of the patient himself can also affect the level of acoustic noise.
Therefore, one way to reduce acoustic noise is reduced magnetic field strength and gradient power (Brummet 1988).
Usually, low-field systems produce less noise. However, an open system with a gradient amplitude of up to 25 mT and a slew rate of 55 T/m/s can produce a significant sound level (<115 dB in the scan room; see Chap. 6).
Another way to reduce acoustic noise is to attenuate the gradient coils, for example, to enclose them into a vacuum. This way has been pursued by Toshiba (pianissimo system, Fig. 4.2).
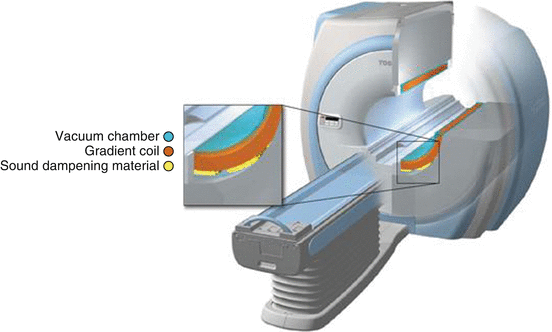
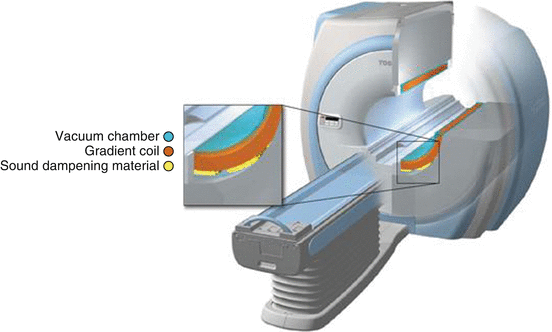
Fig. 4.2
3T MR system with acoustic noise reduction using a vacuum chamber (Pianissimo, Vantage Titan 3T, Toshiba Inc.)
The most innovative way to reduce noise in MRI has been introduced in 2013 to the medical community. The so-called “silent MR” technique (GE Healthcare Inc.) uses gradually increasing gradient field amplitudes to perform MR imaging at 1.5 T without any acoustic noise (Alibek et al. 2014).
4.5 Claustrophobia
Every patient visiting the doctor for a diagnostic procedure experiences a certain amount of psychological stress. The MRI environment increases the stress level. A mild dysphoric psychological reaction has been reported by about 65 % of all patients examined with MR imaging. In extreme cases, claustrophobia can lead to severe anxiety and panic attacks (Shellock 1996, Murphy 1997).
Symptoms of panic attack and emotional distress are conducted by catecholamine output which can result in cardiac arrhythmia or ischemia in susceptible patients.
According to contemporary literature, as many as 20 % of individuals trying to undergo an MRI examination cannot complete the procedure due to serious distress like claustrophobia or unwanted sensations (Dewey 2007).
The most important factors contributing to emotional distress are the physical environment of the MR system, combined with acoustic noise, as well as temperature variations inside of the MR system. Furthermore, the restriction of motion causes distress (Eshed 2007). The feeling of sensory deprivation, particularly in narrow-bore magnets, is experienced negatively by the patient. The duration of the examination plays another important role.
MR systems, using vertical magnetic field, enable to design more open MR units, reducing the frequency of claustrophobic reactions during MR procedures.
Furthermore, the new so-called wide bore provides larger gantry opening, also reducing spatial restriction.
In 1993, a specially designed 0.2 T MRI was made available for MR imaging of the musculoskeletal system. Image quality for diagnosis of the extremities was reported to be comparable to mid- or high-field-strength MR systems and is therefore acceptable as an alternative to whole-body MR systems for musculoskeletal diagnostic imaging.
ONI Inc. developed a one Tesla dedicated extremity MR system which is designed for optimal imaging of the ankle and knee joint as well as the elbow and hand. The system was acquired by GE Healthcare. The plan was to improve magnet and coil performance, as well as image- and signal-processing devices. However, production was stopped recently, since the market acceptance was too low to justify the development costs.
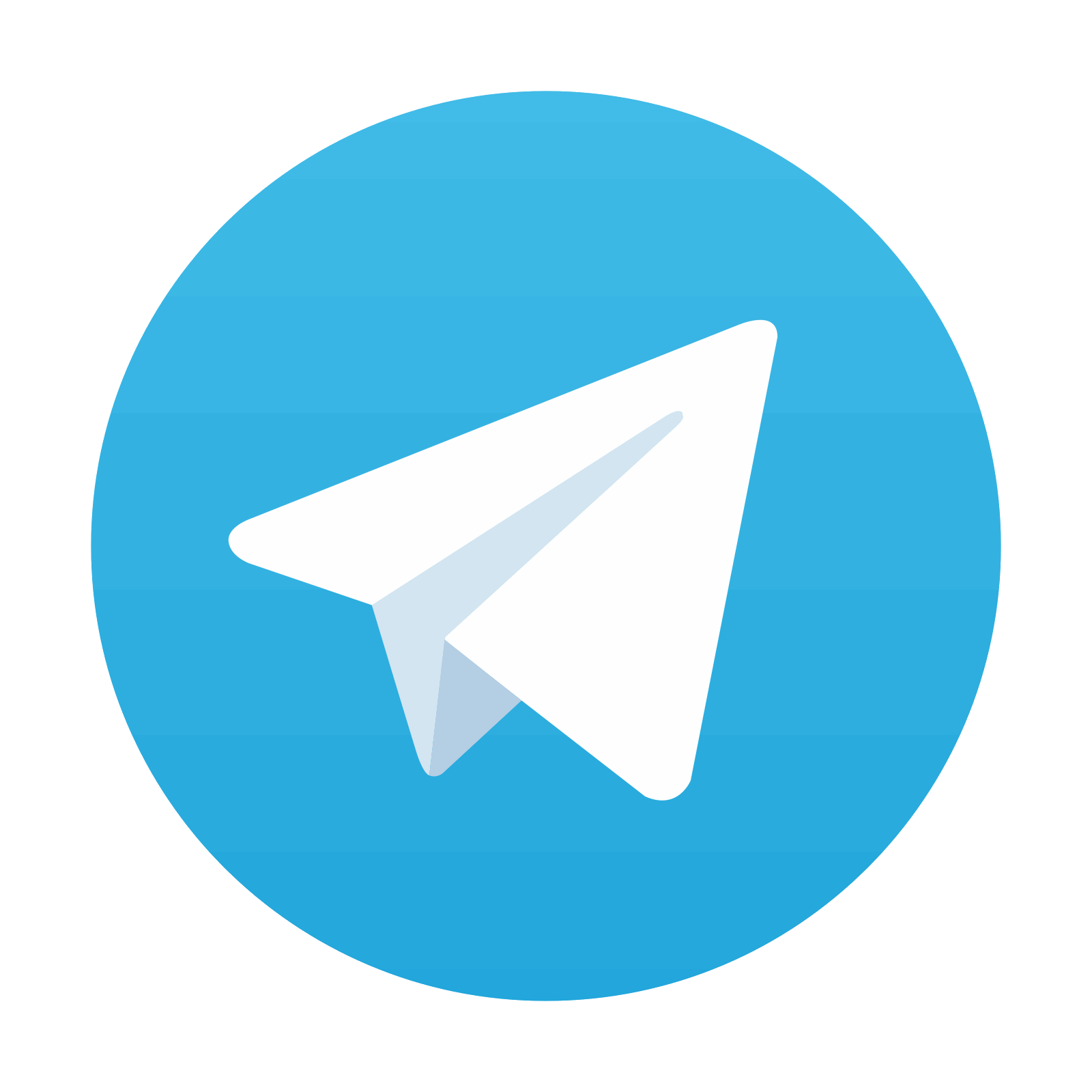
Stay updated, free articles. Join our Telegram channel

Full access? Get Clinical Tree
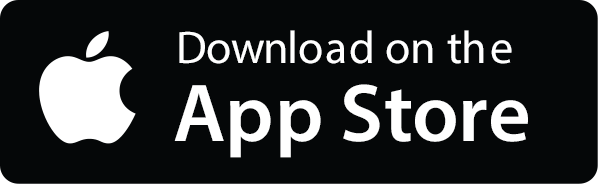
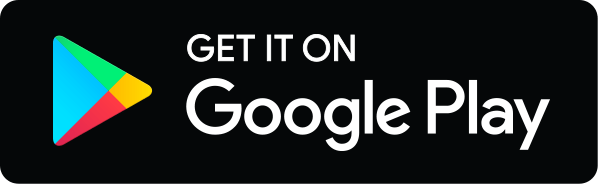