Fig. 1
Cumulative incidence of second cancers among patients treated at the Princess Margaret Hospital for Hodgkin lymphoma, aged ≥30 years at HL diagnosis. The Kaplan–Meier method does not adequately account for competing causes of death that can occur prior to developing a second cancer. As a result, the cumulative incidence of second cancers is overestimtated compared to models that account for competing risks, particularly with prolonged follow-up. Reproduced with permission from Neglia et al. 2006
Several methods to account for competing causes of death that may influence the cumulative incidence of late effects have been described (Pintilie 2006). While a detailed review of these methods are beyond the scope of this review, appropriate clinical interpretation of SC studies requires an understanding that competing risks should be accounted for in study analyses.
3 Radiation Dose-Risk Relationships and Implications for Radiation Therapy
The relationship between low-dose radiation exposures and cancer risk has been studied extensively in studies of Japanese atomic bomb survivors (Ron et al. 1994; Thompson et al. 1994), and those experiencing occupational radiation exposures (Cardis et al. 2007; Lie et al. 2008). Consequently, the relationship between radiation dose and cancer risk is better understood for low-dose exposures than for doses typically prescribed for RT. For most solid tumors and leukemia, there is an approximately linear increase in risk with increasing radiation dose up to about 5 Gy (Boice et al. 1988; Curtis et al. 1994; Preston et al. 2002). At higher doses, cell killing offsets the induction of malignancy in hematopoietic tissue, and the risk of developing leukemia declines (Boice et al. 1987). In part due to this observation, it has been a common clinical precept that second cancers occur at the edge of RT fields because increased cell killing results in lower SC risk for tissues in the “high dose” (i.e., >35 Gy) volume. However, analytic studies of selected second solid cancers among patients receiving RT have demonstrated that this is often not the case (Travis and Gilbert 2005; Travis et al. 2003; van Leeuwen et al. 2003). For example, following mediastinal RT for HL, the risks of breast- and lung cancer have been shown to increase with increasing doses above 30 Gy (Gilbert et al. 2003; Travis et al. 2002), although the slope of the dose-risk curve appears to be less steep in this dose range. Similarly, the risks of soft-tissue sarcoma (Rubino et al. 2005), osteosarcoma (Hawkins et al. 1996), glioma and meningioma (Neglia et al. 2006) have also been found to rise with increasing radiation doses above 20 Gy. In a CCSS study, increasing radiation dose-risk relationships were apparent for both glioma and meningioma, with no downturn of the risk at high doses. At radiation doses of 30–45 Gy to the site of CNS tumor development, the RRs of glioma and meningioma were 21.0 and 96.3, respectively, compared with exposure to less than 1 Gy (Fig. 2). The radiation dose response for glioma was significantly stronger for patients irradiated before age five (Neglia et al. 2006).
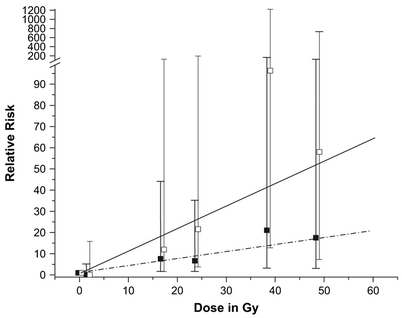
Fig. 2
Relative risk of subsequent glioma and meningioma within the Childhood Cancer Survivor Study cohort by radiation dose (open boxes, mean observed relative risk for meningioma; closed boxes, mean observed relative risk for glioma; solid line, fitted line for meningioma risk; hatched line, fitted line for glioma risk). P < 0.001 (likelihood ratio test, two-sided). Reproduced with permission from Neglia et al. 2006
In contrast, radiation-induced thyroid cancer risk appears to increase with increasing doses up to 10–20 Gy (Ron et al. 1995; Sigurdson et al. 2005), and then plateaus or declines with increasing dose. The dose-risk relationship for radiation-induced leukemia is quite different from that seen for solid tumor induction: the risk of leukemia is much higher at low doses, and there is little or no increased risk at higher dose (i.e. >20 Gy), likely due to bone marrow ablation (Curtis et al. 1992; Little et al. 1999).
These findings suggest two clinically important conclusions relating to RT-related SC risk. First, no single dose-risk relationship will apply to all exposed tissues: there is variation in the sensitivity of different tissues with regard to cancer induction by radiation. Second, for many tissues, the risk of radiation-induced SC increases with increasing doses up to and exceeding doses typically occurring at the edge of treatment fields.
4 Intensity Modulated Radiation Therapy and Second Cancer Risk
An understanding of the radiation dose-risk relationship has important implications when considering the potential therapeutic gains that may be achieved with intensity modulated radiation therapy (IMRT). Compared to conventional RT, IMRT typically achieves more conformal (i.e., smaller) high-dose volumes at the expense of exposing a larger volume of normal tissues to low/intermediate doses. This occurs in part because IMRT often employs more treatment fields than conventional RT, thereby exposing additional tissue to intermediate-dose radiation from these added fields. Further, IMRT may be associated with long “beam on” times (i.e. more monitor units) than conventional RT, and can thereby increase the low-dose total body exposure caused by radiation leakage during treatment (Hall 2006). Finally, use of high energy beams (e.g., 18 MV) may increase total body neutron dose due to the interaction of high energy X-rays with the components of the linear accelerator. A small number of studies have predicted that, in spite of the improved conformality of the high-dose volume, the additional low-dose exposure may increase the risk of second cancers compared to conventional RT (Hall 2006; Schneider 2006).
Radiation oncologists prescribing IMRT should be aware of these issues when weighing the merits of different RT plans. In many cases, a well-designed IMRT plan can deliver an integral dose to the irradiated volume comparable to a non-IMRT plan, with a larger low/intermediate dose volume, but a smaller high-dose volume. In view of the dose-risk data presented above, it remains to be determined how increasing low-dose tissue volume while decreasing the high-dose volume will affect the overall increase in SC risk with IMRT. Also, since externally mounted beam modifying devices (e.g., wedges) attenuate the RT beam, segmented IMRT beams may in some cases require comparatively less “beam on” time to treat the same volume than older techniques. Furthermore, these wedges are often placed in close proximity to the patient and are a source of scatter dose to the patient. (Woo et al. 2006) Consequently, it is not a necessary feature of IMRT that it increases total body exposure compared to conventional RT given historically. (See Fig. 3 comparing IMRT vs. conventional tangent RT for breast cancer treatment).
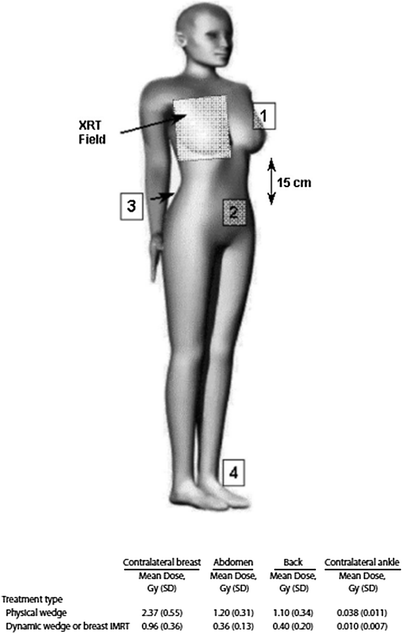
Fig. 3
Scatter dose to normal tissues associated with breast RT, comparing physical wedges and dynamic wedges/IMRT. Because physical wedges are placed close to the patient, they can produce greater scatter dose to normal tissues than techniques that employ segmented multileaf collimation to modify beam intensity. Reproduced with permission from Woo et al. (2006)
Appropriate utilization of IMRT requires both the consideration of primary tumor control as well as the risk of other radiation-related toxicities. The potential increase in absolute risk of SC associated with IMRT over conventional RT (estimated to be <3 % for patients with prostate IMRT (Kry et al. 2007)), should be considered within the context of a patient’s competing risk of death from the primary cancer, and the potential benefit that IMRT may provide in properly treating the primary tumor and reducing the risk of serious chronic organ damage (e.g., xerostomia, rectal toxicity, osteopenia/bone fractures). In particular, clinicians should be aware of the potential for IMRT to increase SC risk, and the features of an IMRT plan that may influence this risk, such as dose to the entire irradiated volume, and the number of monitor units required to deliver treatment. Further research is required to quantify the potential for IMRT to increase the risk of SC compared to conventional RT in order to facilitate more thoughtful balancing of the potential risks and benefits.
5 Second Malignancy Risk in Survivors of Adult-Onset Cancers
Radiation therapy plays a central role as part of curative treatment in a considerable number of adult-onset malignancies. Most of the data on radiation-related second malignancy risks derive from patients with primary cancers in whom RT is routinely (or was historically) used as definitive or adjuvant treatment, and on primary cancers that tend to affect young patients, have a high cure rate, and/or a long natural history. Among survivors of various adult-onset malignancies, the largest amount of data with regard to SC risk exist for survivors of Hodgkin lymphoma. Data on radiation-related second malignancy risks are also available in survivors of testicular cancer, cervical cancer, and more recently breast cancer and prostate cancer.
5.1 Radiation-Related Malignancies After Hodgkin Lymphoma
The cure of Hodgkin lymphoma (HL) using large-field radiation therapy was first demonstrated over 50 years ago (Peters and Middlemiss 1958), with ensuing decades witnessing a considerable evolution of treatment. Excess second malignancies after successful treatment of Hodgkin lymphoma were first reported in the early 1970s (Arseneau et al. 1972). These early reports focused mostly on increased leukemia risks that were largely related to the use of alkylating chemotherapy in a dose-dependent manner. The use of large-field radiation therapy was also initially suggested as a contributing factor to excess leukemias. However, in a case–control study by van Leeuwen et al. (van Leeuwen et al. 1994), the addition of RT to chemotherapy did not significantly increase the risk of leukemia (relative risks of chemotherapy alone versus combined modality therapy: 44.6 vs. 20.9. p = 0.16). Although total-nodal irradiation in combination with chemotherapy was associated with a 2.5-fold elevated risk of leukemia compared with patients treated with chemotherapy alone, the increase was not statistically significant (p = 0.39).
Unlike leukemias after Hodgkin lymphoma, which typically arise within the first 10 years after treatment, solid tumors are associated with a longer latency, ranging from 5 to over 15 years, depending on the subtype of solid tumors. In addition, the risks appear to persist for prolonged periods of time. In more recent studies with longer follow-up time, solid tumors account for over three-quarters of all cases of second malignancies after Hodgkin lymphoma.
The contribution of RT to the development of SC after Hodgkin lymphoma is supported by several studies demonstrating increased risks among patients receiving RT as part of their initial treatment (Aleman et al. 2003; Bhatia et al. 2003; Capra et al. 2007; Hodgson et al. 2007). As noted previously, studies have reported a significant radiation dose–response relationship in the development of specific types of solid tumors after HL. Two case–control studies examined in detail the relationship between radiation dose and the risk of breast cancer after Hodgkin lymphoma therapy (Travis et al. 2003; van Leeuwen et al. 2003). In both studies, the radiation dose at the site of the breast cancer was estimated in the case patients and compared to the dose in a comparable location in the control subjects. In the study by van Leeuwen et al., which consisted of 48 cases of breast cancer and 175 matched controls, the breast cancer risk was significantly increased only after a radiation dose of 38.5 Gy or higher, but not at lower doses (van Leeuwen et al. 2003). In the large, international case–control study by Travis et al. (Travis et al. 2003), which consisted of 105 breast cancer cases and 266 matched controls, a radiation dose of >4 Gy to the breast was associated with a 3.2-fold breast cancer risk compared with women who received lower doses of radiation and no alkylating chemotherapy. The risk increased to 8-fold for women who received >40 Gy to the breast (p trend < 0.001). The comparable results of these studies is due in part to overlap of breast cancer cases evaluated in the Dutch study (40 of 48 cases) (van Leeuwen et al. 2003) that were also included in the international series by Travis et al. (2003).
A significant radiation dose–response relationship has similarly been shown for the development of lung cancer after Hodgkin lymphoma. Travis et al. conducted a case–control study of 222 cases of lung cancer and 444 matched controls in patients who had been treated for Hodgkin lymphoma (Travis et al. 2002). Using patients who received <5 Gy to the area of the lung in which the cancer developed as the reference group, the lung cancer risk increased with increasing dose to the lung (p trend < .001), although the increased risk was statistically significant only after exposure to doses of 30 Gy or higher.
A number of factors have modifying effects on radiation-related second malignancy after Hodgkin lymphoma. For breast cancer after Hodgkin lymphoma, young age at mantle irradiation has consistently been shown to be associated with significantly increased risk for breast cancer in women (Ng et al. 2002; Travis et al. 2003). In addition to age at treatment, ovarian hormonal exposures also have a significant modifying effect on breast cancer risk after Hodgkin lymphoma therapy. In the studies by both Travis et al. 2003 and van Leeuwen et al. 2003 patients who received chemotherapy and radiation therapy had significantly a reduced risk of breast cancer compared with those treated with radiation therapy alone, and the radiation-related risks were also attenuated by a radiation dose of 5 Gy or more delivered to the ovaries. The Dutch study also clearly showed that the substantial risk reduction associated with chemotherapy was attributable to the high frequency of premature menopause in chemotherapy-treated patients (van Leeuwen et al. 2003). Taken together, results in both studies showed that ovarian hormones play a critical role in promoting breast tumorigenesis once radiation has produced an initiating event.
The striking effect of smoking on the treatment-related risk for lung cancer among HL survivors merits emphasis. In a case–control study by Travis and colleagues (2002), in which the reference group consisted of patients who had HL who had minimal radiation exposure and who were nonsmokers or light smokers, those patients who received either alkylating agent chemotherapy alone or 5 Gy or more of radiation therapy alone to the area of the lung in which cancer developed later experienced 4.3-fold and 7.2-fold increased risks for lung cancer, respectively. These relative risks increased to 16.8-fold and 20.2-fold, respectively, in those patients who also smoked at least one pack of cigarettes per day. For cigarette smokers (at least one pack per day) who had also received alkylating chemotherapy and 5 Gy or more of radiation therapy to the area of the lung in which cancer developed, the relative risk for subsequent lung cancer was 49.1, consistent with a multiplicative effect of tobacco use on the risk for treatment-related lung cancer. In view of these findings, clinicians should be diligent in facilitating smoking cessation among both new HL patients and survivors.
In considering SC risk after Hodgkin lymphoma, it should be noted that while RT is a major contributor to excess risks, a number of other factors also influence risk, including chemotherapy (Swerdlow et al. 2000; Travis et al. 2002), underlying immune dysfunction and genetic predisposition. Further, much of the data on solid tumors after radiation therapy for Hodgkin lymphoma was based on patients treated in an era when large treatment fields and higher radiation doses were routinely employed. A significantly reduced volume of normal tissue is exposed with the current standard of care, which includes involved-field radiation therapy as part of combined modality therapy (Fig. 4). A comparative study of mantle RT fields versus contemporary IFRT for patients with mediastinal disease found that the latter was associated with a reduction in radiation dose to female breast tissue of approximately 65, and a 35 % reduction in lung dose (Koh et al. 2007). Radiobiologic modeling predicted comparable reductions in the breast- and lung cancer risks associated with the transition from mantle RT to IFRT (Hodgson et al. 2007). A meta-analysis showed that the risk of breast cancer is significantly higher after extended-field than involved-field radiation therapy (OR, 3.25, p = 0.04), which is likely related to the reduced amount of breast tissue in a more limited treatment field (e.g., exclusion of the axillae) (Franklin et al. 2006). Ongoing clinical efforts are exploring the possibility of further reductions in radiation treatment doses for HL. More recently, there is a trend toward involved-node radiation therapy that further reduces the exposure of normal tissue to radiation (Girinsky et al. 2006). It is thus expected that patients who receive RT in the modern era will likely experience lower risks for second malignancies than those reported in long-term follow-up studies of now outdated treatment.
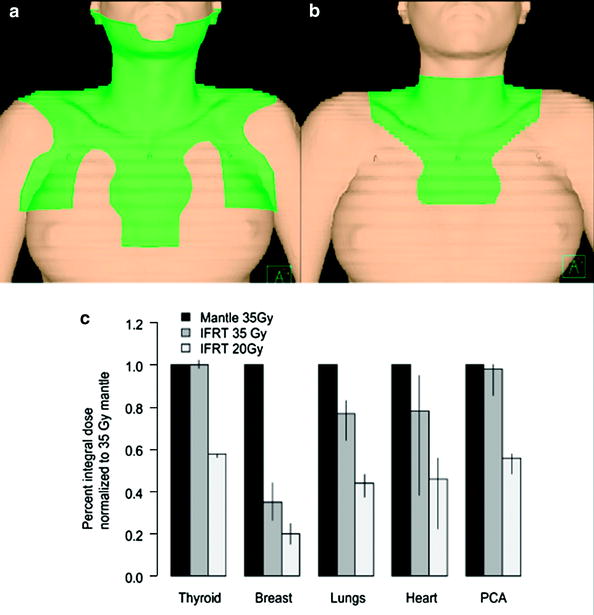
Fig. 4
Dose to normal tissues associated with mantle field (a) and involved-field RT (b) for a female patient with mediastinal HL. Historically used mantle fields contributed significant dose to normal tissue by electively treating the axillae. Restriction of RT to lymph node regions initially involved with disease can significantly reduce normal tissue exposure (c). Many north american pediatric protocols also reduce dose to ≈20 Gy, further reducing normal tissue dose compared to 35 Gy mantle RT. Reproduced with permission from Koh et al. (2007), and Hodgson et al. (2007b)
5.2 Radiation-Related Malignancies After Testicular Cancer
Historically, treatment for testicular cancer included the use of large-field radiation therapy including treatment to the mediastinum and paraaortic and pelvic lymph nodes. Similar to Hodgkin lymphoma, testicular cancer typically affect young adults and has a high cure rate. In addition, as in survivors of Hodgkin lymphoma, second malignancy has been associated with excess mortality among selected long-term survivors of testicular cancer (Schairer et al. 2007). The types of second malignancy documented in survivors of testicular cancer include leukemia and several solid tumors, including mesothelioma, cancers of the lung, thyroid, esophagus, stomach, pancreas, colon, rectum, kidney, bladder, and connective tissue (Pedersen-Bjergaard et al. 1991; Travis et al. 2005; van den Belt-Dusebout et al. 2007). Much of the existing data on treatment-related second malignancy after testicular cancer may reflect the historical routine use of large field RT, although chemotherapy has also been shown to contribute to the risk. An excess risk for contralateral testicular cancer has also been observed, which is likely related to underlying predisposition rather than prior treatment (Fossa et al. 2005).
A population-based case–control study by Travis et al. explored treatment-associated leukemia in men with testicular cancer who had survived at least one year (Travis et al. 2000). The risk for leukemia increased significantly with increasing radiation field size, which was reflected in dose to active bone marrow. In addition, after taking into account the amount of radiation exposure to active bone marrow, the risk for leukemia was also significantly associated with cumulative dose of cisplatin received.
In the largest cohort study on testicular cancer survivors to date, Travis et al. reported on the solid tumor risk among 40,576 patients (Travis et al. 2005). At a mean follow-up of 11.8 years, the relative risks of developing a solid tumor were significantly increased after both radiation therapy and chemotherapy alone (RR, 2.0 and 1.8, respectively). Among 10-year survivors of testicular cancer initially treated with radiation therapy, the relative risks of solid tumors at sites included in typical infradiaphragmatic fields (e.g., bladder, stomach, pancreas, and kidneys) were considerably higher than those at sites not in field. Significantly increased risks of cancers of the esophagus, pleura, or lung were also found, likely reflecting the historical use of prophylactic mediastinal irradiation. In a cohort study from the Netherlands based on 1,909 patients with testicular cancer, the relative risk of second malignancy was 1.6 (95 % CI, 1.3–2.1) (Travis et al. 1993). Patients who had received radiation therapy to the paraaortic lymph nodes and who survived testicular cancer for more than 5 years were at particularly high risk of developing stomach cancer (RR, 6.9; 95 % CI, 3.3–12.7). In a update from the same group based on 2,707 5-year testicular cancer survivors (van den Belt-Dusebout et al. 2007), followed for a median of 17.6 years, the relative risk of second malignancy was 1.7 (95 % C.I., 1.5–1.9), with subdiaphragmatic radiotherapy being strongly associated with increased risk of SC.
5.3 Radiation-Related Malignancy After Cervical Cancer
Both definitive RT and radical surgery are accepted options in the treatment of nonbulky early-stage cervical cancer, while primary RT is typically the treatment of choice for women with bulky stage IB and IIA cervical cancer. Survivors of cervical cancer are at increased risk for developing several second malignancies, some of which can be explained by shared infectious causes or environmental risk factors, whereas others are attributable to previous treatment exposures. Kleinerman and colleagues were among the first to report on the risk of second malignancies following cervical cancer (Kleinerman et al. 1982). Compared with the general population, survivors of cervical cancer had a 1.4-fold increased risk for a second malignancy. A history of radiation therapy was associated with significant excesses of several cancers arising in the pelvic radiation field, including tumors of bladder, kidney, rectum, corpus uteri, and ovary. Since then, several studies have confirmed these findings (Boice et al. 1985; Chaturvedi et al. 2007). Chaturvedi and colleagues updated and expanded these data based on 104,760 1-year survivors of cervical cancer reported to 13 population-based cancer registries in Denmark, Finland, Norway, Sweden, and the United States (Chaturvedi et al. 2007). In this larger study with longer follow-up time, a significantly elevated relative risk (RR = 1.3; 95 % CI, 1.28–1.33) for second malignancy was again found. Moreover, the authors showed that following radiation therapy for cervical cancer, the risks for second cancers of the rectum/anus, colon, urinary bladder, ovary, and other female genital sites remained significantly elevated for more than 40 years. Several second cancers were likely related to shared etiologic factors rather than radiation therapy, because an increased risk was also observed among the non-radiation therapy cohort. These included cancers of the pharynx, genital sites, and rectum/anus, which are related to human papillomavirus infections; and cancers of the lungs, pancreas, and urinary bladder, which are related to tobacco use. In this study, younger age at cervical cancer treatment was associated with a significantly higher cumulative risk for second cancer. After adjustment for competing mortality, the 40-year cumulative risk of any second cancer was higher among women diagnosed with cervical cancer before age 50 than among women diagnosed after age 50 (22.2 vs. 16.4 %).
5.4 Radiation-Related Malignancy After Breast Cancer
Radiation therapy is an important modality in the treatment of breast cancer, either as part of breast-conserving therapy or postmastectomy treatment. Among the various types of second cancers observed in survivors of breast cancer, the largest amount of data exists for contralateral breast cancer, which is partly related to preexisting breast cancer risk factors (Boice et al. 1992; Gao et al. 2003; Hemminki et al. 2007; Kirova et al. 2007; Storm et al. 1992). The increased risk has been estimated to range from two- to five-fold among breast cancer survivors, with conflicting data on the contribution of RT to these excesses. In a case–control study by Boice and colleagues (1992), the overall relative risk for contralateral breast cancer was not significantly increased after radiation therapy (RR = 1.19; 95 % CI, 0.94–1.15). Among women who were younger than 45 years of age at the time of irradiation, however, the relative risk was significantly elevated (RR 1.59; (95 % CI, 1.07–2.36). In the Early Breast Cancer Trialists’ Collaborative Group report, which evaluated the effects of RT, a significantly increased risk for contralateral breast cancer was found, mainly during the period 5 –14 years after randomization (RR = 1.43; P = .00001), and the increased risk associated with RT was significant elevated among women aged 50 years or older when randomized (RR = 1.25; P = .002) (Clarke et al. 2005). In contrast, in another large case–control study from Denmark, there was no significant difference in the risk for contralateral breast cancer in women who did and did not receive RT, regardless of age at treatment (Storm et al. 1992). In the Danish study, it was found that the second breast cancers were evenly distributed in the medial, lateral, and central portions of the breast, which also argued against a causal role of radiotherapy in tumorigenesis. A large-scale, single-institution study from Institut Curie which included 13,472 women, similarly failed to show an increased risk for contralateral breast cancer when comparing women who did or did not receive radiation therapy (RR = 1.1; 95 % CI, 0.96–1.27). Analysis by age, however, was not performed in that study (Kirova et al. 2007).
In addition to contralateral breast cancer, several other solid tumors have also been linked to a history of radiation therapy for breast cancer. These include cancers of the lung, thyroid and esophagus, and soft tissue sarcoma (Huang and Mackillop 2001; Huang et al. 2001; Levi et al. 2005; Neugut et al. 1993; Roychoudhuri et al. 2004; Zablotska and Neugut 2003). An increased risk for leukemia after breast cancer has also been observed, which is associated with exposure to both chemotherapy and radiation therapy.
Women who receive RT for breast cancer have been shown to be at a 1.5- to 3-fold increased risk for developing lung cancer compared with women who did not receive radiation therapy (Neugut et al. 1993; Zablotska and Neugut 2003). The increased risk seemed to be more clearly related to postmastectomy radiation therapy, in which the radiation target volume often also include the supraclavicular, axillary, or internal mammary nodal region, thus exposing a larger volume of underlying lung tissue to the radiation, whereas the risk after post-lumpectomy RT is less certain (Deutsch et al. 2003; Zablotska and Neugut 2003). The observation that lung cancer after breast cancer therapy is more frequently found in the ipsilateral lung also supports the contributing role of radiation to the risk (Zablotska and Neugut 2003). Several studies showed further increases in the risk for lung cancer among smokers who received breast irradiation (Ford et al. 2003; Neugut et al. 1994), although the interaction between tobacco exposure and prior radiation therapy on subsequent lung cancer risk is not as well elucidated as in survivors of HL.
Adjuvant radiation therapy for breast cancer has also been related to excess esophageal cancers (Levi et al. 2005; Roychoudhuri et al. 2004). In a cohort study from the Thames Cancer Registry on 64,782 cases of breast cancer diagnosed between 1961 and 2000, the incidence of esophageal cancer among the 33,763 patients who received radiation therapy and the 31,019 patients who did not receive radiation therapy was compared (Roychoudhuri et al. 2004). The relative risk for esophageal cancer was found to be significantly increased at 2.19 (95 % C.I. 1.10–4.62) at 15 or more years after initial breast cancer treatment. A significantly increased excess risk of esophageal cancer after breast cancer therapy with increasing follow-up time from initial diagnosis was also demonstrated in other population-based studies (Ahsan and Neugut 1998; Levi et al. 2005).
Sarcoma after breast cancer is a rare event, with a 15 year incidence rate of less than 0.5 %, although the relative risk has been estimated to be as high as seven, because of the low background incidence in the general population (Huang and Mackillop 2001; Kirova et al. 2005). In a study by Rubino and colleagues (2005), all observed sarcomas occurred among women who had initially received radiation therapy, and in all cases the sarcomas were located in the irradiated fields or in the upper extremity of the arm ipsilateral to the treated breast. Further, a significant dose–response relationship was demonstrated. By estimating the initial radiation dose to the site of sarcoma development, using a dose of 14 Gy or less to the site as reference, women who received 14–44 Gy had a 1.6-fold increased risk for sarcoma, whereas those who received 45 Gy or more to the site had a 30.6-fold increased risk (P < .001). Angiosarcoma was initially shown to be associated with chronic lymphedema following radical mastectomy (Jessner et al. 1952). With the increasing use of radiation therapy, there have been a growing number of reports of cutaneous angiosarcoma of the breast arising in the radiation field (Brenn and Fletcher 2005; Esler-Brauer et al. 2007; Fodor et al. 2006; Virtanen et al. 2007). Unlike other radiation-related soft tissue sarcoma, angiosarcoma has a short latency and can occur in the first 5 years after therapy.
The increased risk for leukemia following breast cancer is related to both chemotherapy and RT (Campone et al. 2005; Curtis et al. 1992; Praga et al. 2005; Smith et al. 2003). In a case–control study by Curtis and colleagues of women treated for breast cancer between 1973 and 1985 (Curtis et al. 1992
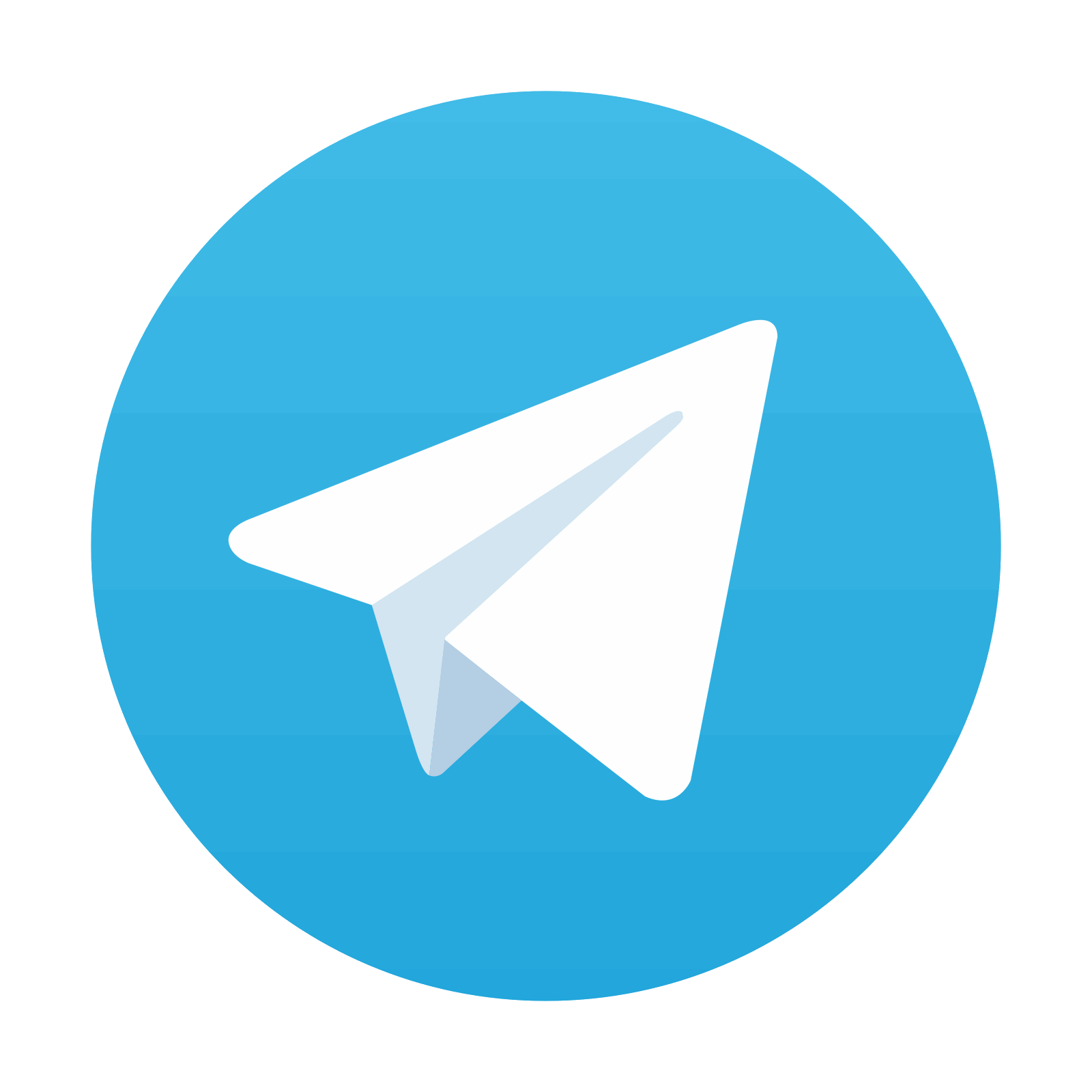
Stay updated, free articles. Join our Telegram channel

Full access? Get Clinical Tree
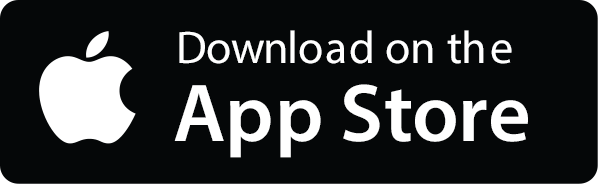
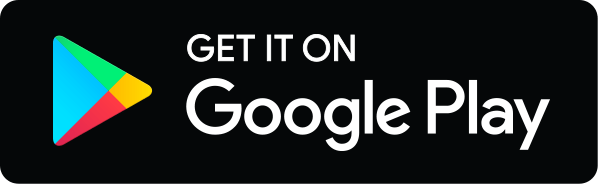