Low risk
Intermediate risk
High risk
Seattle
Zelefsky et al. (1998)
(all factors)
T1-2
+ GS ≤6
+ PSA ≤10
(one factor)
>T2
/ GS ≥7
/ PSA >10
2 or 3 factors
>T2
/ GS ≥7
/ PSA >10
Mount Sinai
Sylvester et al. (2003)
(all factors)
<T2c
+ GS< 7
+ PSA ≤10
(one factor)
≥T2c
/ GS ≥7
/ PSA >10
2 or 3 factors
≥ T2c
/ GS ≥7
/ PSA >10
Boston
D’Amico et al. (1998)
T1c-T2a
+ GS ≤6
+ PSA ≤10
T2b
/ GS = 7
/ PSA >10 and ≤20
T2c
/ GS 8–10
/ PSA >20
AUA
Thompson et al. (2007)
T1c-T2a
+ GS ≤6
+ PSA ≤10
T2b not qualifying for high risk
/ GS = 7
/ PSA 10–20
T2c
/ GS 8–10
/ PSA >20
Table 2
Risk group criteria for prostate cancer
Low risk | Intermediate risk | High risk | ||||
---|---|---|---|---|---|---|
Merrick et al. (2008) | ≤T2a + GS ≤6 + PSA ≤10 | (one factor) T2b / GS = 7 / PSA 10–20 | 2 or 3 factors of intermediate / (≥T2c / GS 8–10 / PSA ≥20) | |||
NICE Great Britain Graham et al. (2008) | (all factors) T1-T2a + GS ≤6 + PSA <10 | T2b-T2c / GS = 7 / PSA 10–20 | T3–T4 / GS 8–10 / PSA >20 | |||
CaPSURE USA Cooperberg et al. (2003) | T1-T2a (1997 TNM system) + GS ≤6 + PSA ≤10 | T2b / GS = 7 / PSA 10–20 | T3-T4 / GS 8–10 / PSA >20 | |||
PPC classification Australia (T1c-T3) Huang et al. (2012) | + GS 2–6 + PSA ≤10 Or (GS = 7 ± PSA 10–20) + PPC ≤50 % | not low/high risk | (GS 8–10 ± PSA >20) + PPC >50% | |||
ESMO Horwich et al. (2010) | T1-2a + GS ≤7 + PSA <10 | not low/high risk | T3–T4 / GS 8–10 / PSA >20 | |||
Five Level Risk Stratification (any T) Australia Beasley et al. (2008) | PSA <7.5 + GS ≤6 | Low int. | High int. | High | Extreme | |
PSA 7.5–15 + GS ≤6 | (PSA 15–20 + GS ≤6) / (PSA ≤10 + GS ≥7) | (PSA 20–30 + GS ≤6) / (PSA 10–20 + GS ≥7) | (PSA >20 + GS ≥7) / (PSA >30 + GS ≤6) | |||
NCCN USA NCCN (2012) | Very low | Low | T2b-T2c / GS = 7 / PSA 10–20 | High | Very high | |
T1c + GS ≤6 + PSA ≤10 + PBC <3 + PPC <50 % PSA-density <0.15 ng/ml/g | T1-T2a + GS ≤6 + PSA ≤10 | T3a / GS = 8–10 / PSA >20 | T3b–T4: (locally advanced) | |||
EAU Heidenreich et al. (2011) | cT1-T2a + GS 2–6 + PSA <10 | cT2b-T2c / GS = 7 / PSA 10–20 | cT3a / GS 8–10 / PSA >20 | cT3b-T4 N0 any T, N1 |
The pre-treatment risk stratification is an area of current debate. Critical prognostic parameters such as tumor extension, invasion of the prostate capsule or the seminal vesicles and Gleason score are ultimately available only after surgery. At primary diagnosis, a clinical T stage is stated by the urologist due to pathological findings in digital rectal examination or transrectal ultrasound, which is prone to significant interobserver variability and staging errors.
Reese et al. found errors in assigning the correct clinical stage in 35.4 % of patients. Most of them resulted in patient downstaging, and TRUS findings are frequently disregarded (Reese et al. 2011). 25 % of patients with Gleason 6 in one or two biopsy cores might reveal a higher Gleason score or extraprostatic extension at prostatectomy (Katz et al. 2011).
In comparison to urologists using pathological findings to predict the outcome after prostatectomy, radiation oncologists have to accept obvious uncertainties in clinical staging categories before therapy. This might strongly affect clinical outcomes.
Concerning this matter, nomograms have been developed to give a more precise prediction of treatment success, complications and acute and long-term morbidity (Stephenson and Kattan 2006; Zelefsky et al. 2012; Kaplan et al. 2012; Roeloffzen et al. 2011). Another nomogram is applied to patients with favorable-risk prostate cancer considered for brachytherapy to estimate the risk for Gleason score upgrading (Bowes et al. 2012b). While these nomograms can help selecting a treatment for prostate cancer, they should not be used as a surrogate for physician’s treatment recommendations.
Additional radiological examinations (high-quality magnetic resonance imaging, spectroscopy) could improve evaluation of tumor location and support accuracy in therapy decision and planning. Imaging fusion techniques with ultrasonography have been established for image-guided prostate biopsy (Hoeks et al. 2011) or post-implant dosimetry (Bowes et al. 2013a). In future, image-guided prostate brachytherapy may open up new therapy approaches (e.g. dose escalation in sub-volumes of the prostate, salvage brachytherapy following EBRT). However, in low risk cancer, usually additional examinations including MRT are currently not recommended.
The difficulty in assigning biochemical failure to risk groups in patients following brachytherapy still remains challenging. Some authors discuss the weakness of the existing three-group stratification system in detail (Rodrigues et al. 2012). They mention novel prognostic factors and the introduction of further risk categories, such as very-low-risk and very high-risk strata. Others refer to the inhomogeneity of intermediate-risk group and suggest a division into a low-intermediate risk group and a high-intermediate risk group (Beasley et al. 2008; Williams et al. 2006). However, the author’s stratification system did not integrate essential risk factors such as amount of high-grade cancer, Gleason pattern 4+3 versus 3+4.
3.1 Patient’s Selection
The patient’s decision, which therapy fits their needs best, is often influenced by the personal attitude of their physician as well as their own attitude. In one study by Anandanas et al., 60 % of the patients with early prostate cancer, who chose radical prostatectomy (40 %), did so because they wanted physical removal of their tumor. Twenty-seven percent of men with EBRT (31 %) had fear of other treatment options, and the main reason (39 %) for choosing brachytherapy (21 %) was lifestyle consideration. There was no predominant reason for choosing Active Surveillance (8 %). After 2 years, all patient groups (Surgery, EBRT, PSI, and Active Surveillance) showed comparable satisfaction with their treatment decision (Anandadas et al. 2011). Other studies showed a significant better patient satisfaction following PSI compared to Surgery or EBRT (Crook et al. 2011; Wagner et al. 2011).
Remarkably, choosing radiation is generally a more difficult decision than choosing surgery (Sidana et al. 2012). Because “Doctor’s recommendation” is the main reason for selecting a treatment, there might be a difference between surgeons and radiation oncologists in terms of persuasive power or argumentation (Patient is “too young for less aggressive treatment”, “Avoid RT: difficult to treat recurrence”).
Holmes et al. presented a patterns of care study of the relationship between age, the likelihood of extraprostatic cancer and primary therapy. Patients younger than 50 years receive more often primary surgery. In older patients, there may be a higher use of conservative treatment, even with >50 % likelihood of extraprostatic cancer (Holmes et al. 2012). Thus, multidisciplinary consultation should be established as a standard, to result in individualized treatment recommendations based on age, risk strata and comorbidities.
4 Risk Groups
4.1 Low-Risk
Traditionally, PSI is a domain of low-risk cancer, mostly defined as cT1c-2a, Gleason Score 2–6, and PSA ≤10 ng/ml. This stage describes a localized growing tumor with estimation of low malignancy. Therefore, every local treatment approaches should result in comparable high cure rates.
Despite plenty of single institutional studies with presentation of long-term results, randomized studies are rare. In the low-risk group, excellent biochemical relapse-free survival up to 95.6 % (12 year) and 85.9 % (15 year) are reported (Taira et al. 2011; Sylvester et al. 2007). Studies with the largest patient numbers report biochemical freedom of failure (BFFF) in about 88 % of the patients (Stone et al. 2011), with clinical stage, Gleason Score, PSA level and biological effective dose as significant predictors.
A matched-pair analysis proved a superior biochemical control of PSI after 5 years in comparison to EBRT (94 vs. 88 %) (Pickles et al. 2010). For low-risk patients with assumed organ-confined disease, there may be no advantage of additional EBRT.
In studies regarding surgery, it is often unclear if the risk stratification is based on the pre-therapy assumed T-stage, or on the histopathological report. With more accurate knowledge on the exact tumor burden, the risk classification is more reliable, and statistics may show better outcomes for “real” early tumor stages. Therefore, comparison of outcomes after prostatectomy with those following PSI is complex.
Older series should not further be used for argumentation, because of lower radiation doses, higher PSA median in EBRT group and ancient techniques used for PSI (D’Amico et al. 1998).
In a comprehensive review of papers published during 2000–2010, PSI provides superior outcome regarding PSA-free progression in patients with low-risk disease in comparison to surgery, EBRT and other treatment approaches. (Grimm et al. 2012).
A cohort analysis of outcomes after radical prostatectomy or brachytherapy in 371 men with low- or intermediate risk prostate cancer recently was published by Fisher et al. Five years after surgery, bRFS rates for low—and intermediate-risk disease were 96.1 and 90.6 %, and 92.5 and 95.8 % after brachytherapy, respectively (Fisher et al. 2012).
Due to the lack of randomized trials, the Deutsche Krebsgesellschaft (German Cancer Society) has started a preference based study to evaluate surgery, EBRT, PSI and active surveillance in low risk and low-intermediate risk patient groups. Patients participating in this study will be randomized in nine substudies according to their preferred treatment options (PREFEREnce based randomized evaluation of treatment modalities in low or early intermediate risk prostate cancer) (Schmedders et al. 2011).
4.2 Intermediate-Risk
Definitions for intermediate-risk criteria may be different from series to series, therefore outcome results may vary slightly. An intermediate-risk tumor is more aggressive than low risk tumors, but still organconfined. The risk for local infiltration of the prostate’s capsula or the base of the seminal vesicles is strikingly increased, thus the extent of periprostatic treatment margins of PSI plays an important role if it is used as a single therapy approach for these patients. Regarding biochemical free progression in the intermediate risk group, PSI alone seems to be equivalent to PSI + EBRT. (Grimm et al. 2012; Merrick et al. 2005).
The direct comparison of surgery with radiotherapy-based approaches like EBRT, PSI or the combination of both to prove the superiorness of any therapy approach is still a reason for intensive disputes between urologists and radio-oncologists (Eastham 2004; Petros 2004). In the lack of prospective, randomized trials, this question still remains open.
While some retrospective surveys reported almost comparable results for BRFS in patients with low- and intermediate risk (Fisher et al. 2012; Merrick et al. 2001), other groups seem to clearly recommend radiotherapy-based options in the treatment of all risk strata of prostate cancer (Grimm et al. 2012).
Survival is still high. In a retrospective single institution analysis, Merrick et al. report that at 8 years, 95.7 % of patients (n = 251) were free of biochemical failure after PSI alone for intermediate risk cancer (Merrick et al. 2005).
Survival results may vary, when different risk group scoring schemes are used. Sylvester et al. presented biochemical relapse-free survival results in patients with intermediate risk prostate cancer, treated with PSI plus neoadjuvant EBRT. After 10 years, biochemical relapse-free survival were 77, 93, 90 % for Seattle risk group, Mt. Sinai risk group and D’Amico risk group, respectively (Sylvester et al. 2003). Disease-specific survival rates range between 87 % (10-year) (Hinnen et al. 2010), 95 % (10-year) (Henry et al. 2010) and 99.3 % (12-year) (Taira et al. 2011).
In the intermediate risk group, PSI is often combined with EBRT.
Long-term (8-years) results of RTOG 0019 (Phase II EBRT+PSI, primary endpoint: grade 3–5 genitourinary and gastrointestinal toxicity, closed in 2001) revealed 15 % grade >3 toxicity (urinary frequency, dysuria, proctitis). Forty-two % complained of grade 3 impotence. Unfortunately, EBRT (45 Gy) consisted of a outdated four-field technique (Lawton et al. 2012). Current techniques for PSI and EBRT are able to reduce side-effects when used in combination. Zelefsky reported 4 years after real-time intraoperative planning for PSI combined with intensity-modulated radiotherapy with no gastrointestinal Grade 3–4 complications and only one of 127 patients developed a Grade 3 urethral stricture (Zelefsky et al. 2008). Therefore, modern techniques should claimed as the standard approach when PSI is combined with EBRT.
Data of the randomised RTOG 0232 study, (accrural closed in 2012) are awaited and will report on differences between freedom from progression (FFP) 5 years after EBRT + PSI vs. PSI alone in intermediate risk patients. Remarkably, intermediate risk in that study is defined as Gleason Score = 7 with PSA <10 ng/ml or GS <7 and PSA 10–20 ng/ml, which is a subgroup of D’Amicos intermediate-risk definition (RTOG 2011).
In a retrospective series, Tanaka et al. reported a significantly higher late gastrointestinal (GI) and genitourinary (GU) toxicity in patients with EBRT+PSI. As expected, pre-implantation-IPSS was a significant predictor for acute GU toxicity and the combination of EBRT+PSI was predictive for late GU toxicity. The rectal volume receiving at least 100 % of the prescribed dose (rV100) was associated with late GI toxicity (Tanaka et al. 2013).
As the combination of EBRT and PSI carries the potential risk of increased side effects and complications, this treatment option should only be performed in experienced centers.
The most common indication for the combination of PSI with androgen deprivation (ADT) is prostate size reduction to avoid pubic arch interference during needle insertion. There are no randomized studies proving a benefit for survival. In the retrospective study by Lee et al., the 5-year actuarial freedom from biochemical failure rate increased significantly, when ADT was given 3 months before and after PSI. The best outcome (94 %, n = 40) had patients with a higher implant dose (D90 >140 Gy) and ADT (Lee et al. 2002).
New data suggest a need for additive ADT only in lower dose PSI with BED <150 Gy (Stock et al. 2012b).
When a five-level risk stratification system is used, the cut-off for advantageous use of ADT is the high-intermediate risk group, defined as an initial PSA of 15–20 ng/ml with a GS ≤6 or a PSA ≤10 with a GS ≥7. In this group, 5-year biochemical control (bNED) with or without ADT were 72 and 58 % respectively (Beasley et al. 2008).
Beyer et al., in their retrospective review of 2.378 consecutive PSI cases (33 % intermediate risk, 19.5 % with ADT) reported a surprising effect of short-course ADT 3–6 months before and 3 months after PSI: at 10 years, overall survival was significantly worse with ADT (20 vs. 44 % in hormone naïve patients, p = 0.02) (Beyer et al. 2005).
In conclusion, the combination of PSI and ADT should be administered with careful consideration, especially in the intermediate risk group.
4.3 High-Risk
The high-risk group is defined as clinical stage T2c or Gleason >7 or PSA >20 ng/ml and has a high probability of microscopic, clinical occult metastases already at diagnosis. Interstitial PSI for these patients is an insufficient monotherapy approach due to the limited dose range of this treatment modality.
According to the guidelines, patients should receive supplemental EBRT ± ADT. In a meta-analysis, this management appears superior to more localized treatment approaches such as surgery, EBRT or PSI alone (Grimm et al. 2012). Results of studies using the SEER Database showed, that treatment approaches including PSI in addition to EBRT in high-risk cancers lead to a significant reduction in 10-year prostate cancer-specific mortality (PCSM) (13.4 % for PSI+EBRT vs. 21.1 % for EBRT alone) (Shen et al. 2012). The key benefit of brachytherapy (also in combination with EBRT) in high-risk cancers is the ability to deliver a higher biological effective dose than with EBRT alone, however, the high effectiveness in this situation can only be achieved when the dose is able to completely enclose clinical occult tumor.
In case of high risk patients: (Gleason Score ≥7 or PSA >10ng/ml or clinical stage ≥T2b) who elect PSI as their treatment component of choice, seminal vesicle biopsy (SVB) can be offered as a further diagnostic step. If positive for tumor invasion (9.9 %), additional seeds can be positioned in the anterior wall of the seminal vesicles (Stone et al. 2012).
The high-risk group may consist of several subgroups. Fang et al. (Fang et al. 2011) described a series of patients (n = 174) with Gleason Score 8–10 tumors and a relative low pretreatment PSA value of ≤15 ng/ml who received PSI. Most of the patients (91.4 %) were additionally treated with EBRT with 45 Gy. Short-term or long-term ADT was administered in 64.9 % of patients. For this group, ten-year-outcomes were surprisingly excellent: biochemically progression free survival (bPFS) was 90 % and overall survival (OS) was 69.4 %. Interestingly, the use of ADT did not significantly impact bPFS, CSS or OS.
Bittner et al. (2012) defined a “very-high risk group” with the following criteria: any Gleason Score 10 or Gleason Score 8–9 with >50 % of positive biopsy cores (PPC) or Gleason Score 8–9 with PSA>20 ng/ml or any T3 or any PSA>40 ng/ml.
At 12 years, bPFS was 86.5 % and OS 60.5 % (all patients received PSI, 91.6 % EBRT and ADT 76.3 %). Remarkably, death from diseases of the heart was still more than twice as likely as death from prostate cancer.
High-risk patients with EBRT ± PSI may profit by additive ADT, when distant microscopic disease is expected. In a prospective, non-randomised data set, Beasley et al. (Beasley et al. 2008) found an increase of biochemical control, when ADT was added to EBRT (55 % vs. 42 % without ADT, p = 0.004).
One retrospective study demonstrated that combined therapy with EBRT and PSI seems to be superior compared to dose-escalated EBRT. Additional use of ADT decreases biochemical failure and prostate cancer specific mortality. The authors report the greatest benefit was observed for long-term ADT (Shilkrut et al. 2012).
In the newest publication of Stone and Stock, the factors influencing 15-year cause-specific and all-cause survival (ACS) of patients treated with PSI were analyzed. In the analyzed patient group, long term ADT (>6 months) has a significant negative impact on ACS in both younger and older men. Therefore long term ADT has to be administered carefully, especially when other risk factors such as cardiovascular comorbidities or diabetes are present (Stone and Stock 2014a).
4.4 Gleason Score 3+4 Versus 4+3
After radical prostatectomy, patients with a higher primary Gleason pattern have higher rates of extracapsular extension or seminal vesicle invasion. Consequently, the risk for biochemical failure is significantly higher (Alenda et al. 2011). It is assumed, that tumors with Gleason 3 pattern are more benign than those with Gleason pattern 4 (Lavery and Droller 2012).
In a survey, the difference in primary Gleason pattern played only a subsidiary role in brachytherapy (Stock et al. 2012a; Herbert et al. 2012), although conflicting results have been reported (Uesugi et al. 2012; Bittner et al. 2013). The authors detected no significant effect on biochemical failure after 10 years independent from supplemental EBRT or ADT (Stock et al. 2012a). Anyhow, patients with Gleason pattern 4+3 deserve careful consideration of additional risk factors (PSA >10 ng/ml, clinical Stage, volume of cancer on biopsy). PSI alone, and a biologically effective dose (BED) ≤160 Gy2 might lead to increased biochemical failure, while combined therapy (e.g. ADT and PSI or PSI and EBRT) seems to be the treatment of first choice (Stock et al. 2012a).
4.5 Does Age Matter?
Younger men (<50 years) with prostate cancer are more frequent candidates for surgery, because urologists typically recommend surgery as the best chance of long-term cure. Younger patients also feel that radiation is a less aggressive therapy than surgery or they are concerned about difficulties in case of tumor recurrence after radiotherapy (Sidana et al. 2012).
In fact, younger patients (in this study defined as ≤60 years), at average have smaller, better differentiated tumours, with a consequently better prognosis than patients over 60 years (Hinnen et al. 2011). Additionally, younger patients (<50 years) seem to have a lower incidence of extraprostatic extension, seminal vesicle involvement and non-organ-confined disease rate compared with older men aged from 60–69 years (Khan et al. 2003). Therefore, with a higher prevalence of organ-confined disease, all local treatment approaches like radical prostatectomy—but also PSI—may lead to similar survival results in the treatment of younger patients with prostate cancer. Ten years after surgery (PSA <0.2 ng/ml) and PSI (Phoenix, +2 ng/ml above nadir), biochemical free survival of younger patients (<60 years) is about 78–81 and 91.3–95 %, respectively (Khan et al. 2003; Burri et al. 2010; Shapiro et al. 2009). However, this raw comparison does not qualify to deduce any treatment preference.
Merrick et al. presented a data set of 42 consecutive patients ≤50 years with PSI ± supplemental therapies. All risk groups were represented in this cohort. Due to the small patient number in the particular risk groups, they reported the cause-specific survival, bPFS, and OS only for the entire cohort, which was: 100, 97.7, and 100 %, respectively (Merrick et al. 2008). The same group presented a retrospective report of young patients (≤54 years, n = 108) who underwent PSI or EBRT+PSI for clinically localized prostate cancer (cT1c-T2N0M0). At 8 years, biochemical progression-free survival was 96, 100 and 75 % (three of four patients) for the groups with low, intermediate and high-risk tumor, respectively (Merrick et al. 2006).
Another study presented the results of 175 patients (≤55 years) with PSI for T1-3 prostate cancer. Additionally EBRT + ADT was administered to selected low-, intermediate- and high-risk patients. Freedom from biochemical failure (FFBF) after a median follow-up of 4.7 years was 98.7, 97.1 and 89.3 % in the low, intermediate and high risk group, respectively (Boo et al. 2012).
Hinnen et al. presented 10-year outcomes in patients aged ≤60 years with PSI as monotherapy for locally confined, intermediate or well-differentiated tumors: FFBF, disease-specific survival and overall survival were 63, 87 and 81 %, respectively (Hinnen et al. 2011). For low, intermediate and high risk patients, a 10-year freedom from progression was reported from Shapiro et al. as 91.3, 80 and 70.2 %, compared to 91.8, 83.4, and 72.1 % respectively, for men 60 years or older (Shapiro et al. 2009). Similar results were published from other groups (Burri et al. 2010).
Only few studies have analysed survival rates of elderly men, but the data suggest an effective and safe monotherapy in over 75 years old men. In one study, the 5-year FFBF rate was 91.3 % (OS 95 %) with 5 % grade 3 genitourinary toxicity (Chiumento et al. 2013).
In conclusion, young men achieve excellent biochemical control rates with PSI for localized prostate cancer. With regard to comorbidities and adverse effects, administering PSI to elderly patients should be practiced with careful consideration. Patient age alone should not influence treatment decisions.
4.6 Does Size Matter?
Patients with prostate gland size <20 cm3 are often considered poor candidates for prostate brachytherapy. Some studies have published the association between small glands and inadequate dosimetry, larger 1-month edema, lower D90 and higher intracapsular seed density (Liu et al. 2010; McNeely et al. 2004).
Small prostate sizes seem to be associated with an increased risk of occult higher-grade disease and extracapsular extension. An increased risk for Gleason upgrade was described by Turley and revised later as an effect of the biopsy technique (Turley et al. 2008a, b).
It has to be emphasized, that for high-quality PSI of small sizes, brachytherapy team experience and the achievement of adequate treatment margins is substantial. Under this condition, PSI of smaller glands is at no higher risk of treatment failure than in men with larger prostates (Liu et al. 2010; Taira et al. 2012; Mayadev et al. 2010).
The common recommendation is to be cautious with PSI of large prostates >60 cm3 at the time of implantation (Davis et al. 2012). Depending on prostate size, but also on individual pelvic anatomy, intraoperative patient position and technique, some prostate glands may be covered by the pubic arch on both lateral or ventral sides (“pubic arch interference”). Thus, these regions can not be punctured with the implantation needles, which may result in insufficient dose coverage.
Larger prostates, up to 100 cm3 are technically challenging, but toxicity and outcomes are acceptable (Dallas et al. 2012). Despite of conflicting results, there are broad hints for an increased urinary retention after PSI in patients with large gland size (Crook et al. 2002a). A nomogram can be used to predict the risk for acute urinary retention. Besides prostate size, factors such as IPSS and ADT are elements of the scoring system. Prostate protrusion is a substantial predicting factor for post-PSI urinary retention, especially in larger glands (Roeloffzen et al. 2011, 2012).
Larger glands may result in an overdosage of the anterior rectum and the bladder neck, as a result of a broader contact area.
In case of larger glands, several techniques are used to overcome technical issues such as pubic arch interference: exaggeration of intraoperative lithotomy position, downsizing with 5-α reductase inhibitors or angling the rectal probe tip anteriorly. With the use of special equipment, oblique needle guidance supports a more precise implantation approach than free-hand insertion of the needles (Ryu et al. 2012).
Second, conventionally used ultrasound transducers generate an angulated image field in the axial plane. Larger glands tend to “wrap” around the rectum, especially when the ultrasound tip is forced ventrally or the rectal balloon is overfilled with water for better imaging. Some modern ultrasound devices are equipped with a greater image field angle in the axial plane, which is necessary to display dorsolateral regions of very large prostate glands.
Neoadjuvant ADT reduces gland size by an average of approximately 30 % in 3–4 months (Petit et al. 2007), but involves the risk of urinary retention and prolonged catherization (Crook et al. 2002a; Lee et al. 2010).
On the other hand, a survey with a larger patient number didn’t confirm this observation. There was no significant increase in urinary retention, although the ADT-group had higher pre-treatment IPS-Scores. Furthermore, IPSS increased less and returned to pre-implantation baseline more rapidly when ADT was used. Only a subgroup of men with an IPSS ≥15 showed beneficial influence of the use of neoadjuvant ADT on the risk of urinary retention. In summary, patients with large glands and minimal urinary symptoms lack the need for neoadjuvant ADT, as long the brachytherapy team has the expertise to treat them (Stone et al. 2010a; Blasko 2004).
In conclusion, no clear relationship exists between large prostate size and increased urinary toxicity. Anyhow, due to existing hints, patients should be informed about the potentially increased risk.
4.7 Does Dose Matter?
Commonly, whole prostate D90, V100 and BED are accepted as dosimetric values for defining PSI implant quality. These metrics can be stratified in different subcategories as explained further below (see “Postplanning”) and a lower dose has an obvious impact on biochemical and local control (Miles et al. 2010; Ho et al. 2009; Stock et al. 1998). Nevertheless, this correlation may not be assumed in general. Ash et al. reported that there is only a significant dose response relationship in low risk, but not in intermediate or high risk prostate cancer (Ash et al. 2006). This may be attibuted to different causes. Firstly, there are different criteria for PSA or local failure (ASTRO, Phoenix, post-treatment biopsies), which may result in different statistical outcomes. Secondly, a low whole-prostate D90 is not always associated with treatment failure, when enough dose is delivered to the tumor-affected parts of the prostate (Sidhu et al. 2002). Further on, in intermediate and high risk cancers there might be a higher prevalence of clinical occult microscopic disease outside the prostate which cannot be treated by PSI alone. In this case, an optimal implant with high D90 will possibly result in biochemical failure. At least, data sets regarding intermediate or high risk patient groups with additional ADT need longer follow-up periods to appreciate the impact of higher doses on biochemical control (Stone and Stock 2014b).
In conclusion, there is obvious evidence to regard dosimetric values like D90, V100 or BED as suitable metrics for the estimation of implant quality, but when these metrics are correlated with biochemical treatment failure, multiple aspects have to be taken thoughtfully into account.
5 Indications
Patients with low-, intermediate- and high-risk (Table 1) localized prostate cancer may be candidates for PSI alone or in combination with additional therapy approaches like EBRT and/or ADT.
In short, patients with low-risk disease are ideal candidates for PSI as monotherapy. In intermediate risk, the combination of EBRT and PSI appears equivalent to PSI alone, on the condition that the brachytherapy team provides high expertise and dose margins are selected wide enough. For high-risk patients, combination therapies involving EBRT and PSI ± ADT appear superior to localized approaches (Grimm et al. 2012; Davis et al. 2012).
Before decision-making, patient’s life expectancy should be estimated, especially if comorbidities are present. Generally, a life expectancy of >10 years for low-grade and >5 years for intermediate risk patients is suggested (NCCN 2012; Davis et al. 2012; Frank et al. 2011).
Patients with a PSA >50 ng/ml are unlikely to benefit from local treatment due to the high risk for extraprostatic or metastatic disease. Some authors, however, have demonstrated reduction of prostate cancer mortality even in patients with highly elevated PSA-levels (Bittner et al. 2012).
According to the NCCN and German guidelines (NCCN 2012; Deutsche Gesellschaft für Urologie 2011), additional imaging is not mandatory in low-risk disease. A bone scan is indicated if the following factors are present: T1 and PSA >20 ng/ml, T2 and PSA >10 ng/ml, GS >7, T-Stage >T2 or symptomatic skeletal disorders. Pelvic CT or MRI is indicated with a PSA >10 ng/ml, in higher T-stages, or when the nomogram-predicted risk for lymh node involvement is above 20 %.
If brachytherapy is considered as a monotherapy and clinically atypical aspects are present (e.g. 1 positive biopsy core and PSA>10 ng/ml or 100 % positive biopsy cores in T1c), MRI with an endorectal coil and additional sequences, may be helpful. For treatment planning purposes, a standard body array coil MRT is advantageous over endorectal coil MRI, due to its anatomic distortion of the prostate (Albert et al. 2013).
In selected cases, a seminal vesicle biopsy can provide additional information especially if a lesion is detected in MRI that might originate from bleeding after initial prostate biopsy.
PET/CT is not recommended for primary staging, but may help to exclude patients with distant metastases in high-risk groups from local therapy approaches.
6 Contraindications
Several conditions are considered as absolute or relative contra-indications for prostate brachytherapy.
After transurethral resection of the prostate (TUR-P) PSI should be postponed for 6–12 moths. It depends on the size of prostatic defect, whether PSI succeeds with adequate dose distribution, but these patients are at a higher risk for seed loss, urethral necrosis, strictures and incontinence (Ishiyama et al. 2012; McElveen et al. 2004). Limited TUR-P or transurethral incision of the prostate 6 months before PSI for patients with lower urinary tract syndromes (LUTS), elevated IPS-Score or postvoid residual volume >100 ml appears to be safe and doesn’t result in retention, urethral necrosis or incontinence (Ivanowicz et al. 2012).
Patients with anticoagulants should stop it at least seven days before implantation. Surprisingly, patients with anticoagulants show a superior biochemical control, since anticoagulants may modulate tumor proliferation, angiogenesis and metastasis (Choe et al. 2010).
The Management of pubic arch interference is discussed elsewhere (see “Does Size Matter?”).
The International Prostate Symptom Score (IPSS) is a validated tool of the American Urological Association (AUA) to quantify benign prostatic hyperplasia symptoms.
In short, the use of IPSS is a commonly accepted tool among prostate brachytherapists for scaling acute urinary morbidity due to its simplicity. The preimplant IPSS correlates with the duration of postimplant obstructive symptomatology, patients with a score >20 are reported to be in 29 % risk of retention (IPSS<10: 2 %, IPSS 10–20: 19.11 %) (Terk et al. 1998). Therefore patients with an IPSS above 15, and post-void residual volumes above 100 cm3 are regarded as poor candidates for PSI (Frank et al. 2011).
Other groups defined a three-tier system: patients with an IPSS of 0–8 do well after PSI, patients with an IPSS of 9–19 do fair, and PSI is not recommended above an IPSS of 20 (Ash et al. 2000).
To predict the risk for acute urinary retention after PSI, a nomogram may be used, which includes the pre-implantation IPS-Score (Roeloffzen et al. 2011).
The flow maximum in uroflowmetry Qmax is another component of treatment decision recommendations. Patients with Qmax >15 ml/s are optimal for PSI. PSI is not recommended for patients with Qmax <10 ml/s (Ash et al. 2000).
This parameter is typically reduced in patients with urethral strictures and higher age, therefore it has a great value in recognising and quantifying existing urinary impairments before PSI, if age is taken into account.
In patients with an IPS-Score ≥7, measurement of uroflow and postvoid residual volume should be carried out when missing (Crook et al. 2002a).
Early voiding disorders after PSI are caused by the traumatic effect of needle implantation and mild swelling of the gland, late disorders are caused by the effects of radioactivity. Therefore, Qmax decreases in the first 1–3 months after PSI, and returns to baseline one year after seed implantation. The changes of IPSS correlate well with the objective parameters Qmax and voided volume (Tanaka et al. 2009; Mallick et al. 2003).
7 Implantation Methods
The technique of permanent seed implantation has been described in many publications. While the basic technique of real-time, rectal ultrasound assisted PSI is similar in all centers (Fig. 1), slight modifications may consist in the type of applicator (Needles vs. Mick-Applicator) and seeds used (stranded vs. single vs. both types used).
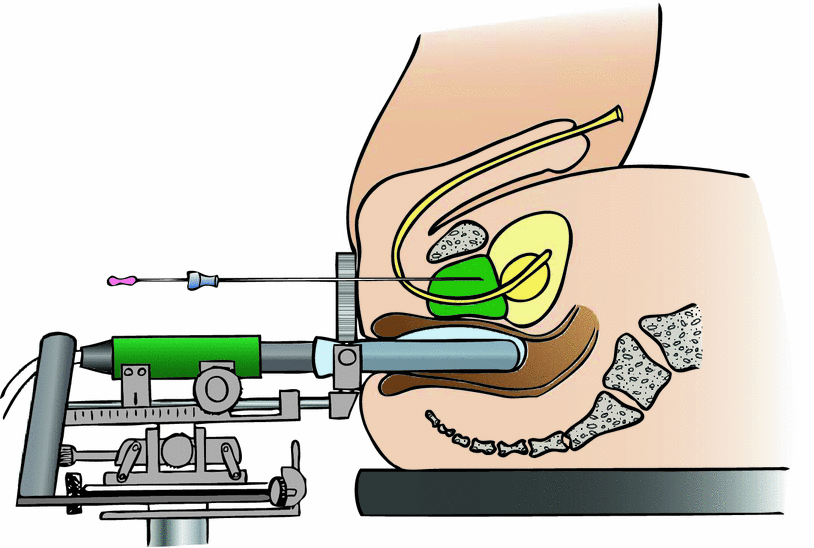
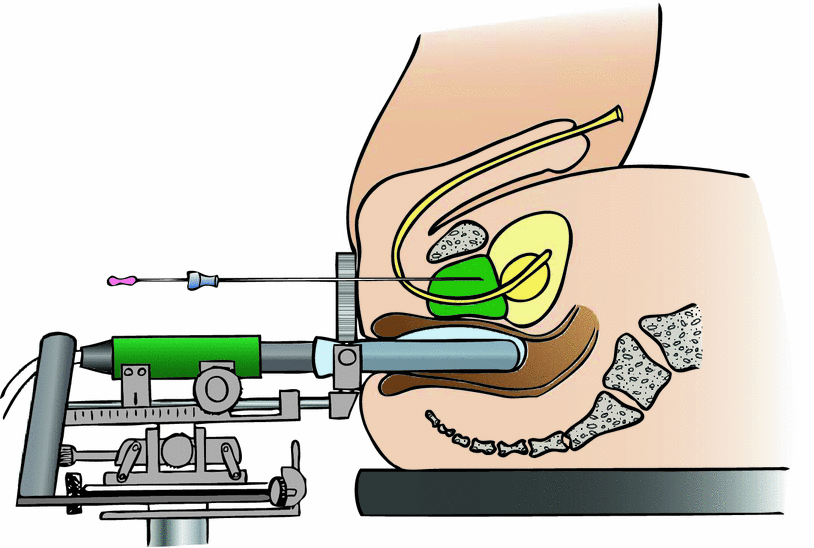
Fig. 1
View of setup for permanent seed implantation. The patient is placed under general anesthesia in exaggerated lithotomy position. A biplane rectal ultrasound system with template (two-directional coordinate system with prefabricated perforations at regular 3–5 mm intervals), stepper (mounting device) and rectal balloon is applied. The urethra may be visualized on the ultrasound images by a urinary catheter or aerated ultrasound gel. Special fixation needles may be used to minimize prostate motion during procedure (not illustrated). After image acquisition of the prostate in 3–5 mm-steps (plus one slice in cranial and caudal direction: “cut above” and “cut below”), brachytherapy planning is performed. During the implantation procedure, the needles with preloaded (stranded) seeds are inserted at the planned coordinates of the template. The precise position of the needle is detected on the real-time ultrasound images (axial plane). Then, in the longitudinal ultrasound plane, the needle is pushed forward to the intended depth. While the wire stylus is fixed, the needle is slowly pulled out, and the seeds are ejected in the tissue of the prostate gland
7.1 Loose Versus Stranded Seeds
Stranded seeds are fabricated with absorbable spacer material, which fix the distance of 1 cm between the centers of two serial seeds. In some models, loose spacers of different length can be used to build individual designed strands. The main advantage of stranded seeds is the capability of minimizing the risk for seed loss.
Newer studies using free seeds reported about seed loss in about ¼ of patients: 22.8 % (Taussky et al. 2012), 25.6 % (Miyazawa et al. 2012), 24.7 % (Sugawara et al. 2011), and 30 % (Reed et al. 2007).
Loose seeds can migrate through the venous system to the lungs, abdomen and pelvis or are excreted with urine or ejaculate. Rare locations are the coronary artery (risk of myocardial infarction) (Zhu et al. 2006; Davis et al. 2002), the renal artery (Nguyen et al. 2009) or the vertebral venous plexus (Hau et al. 2011; Wagner et al. 2010).
Most of the seeds get lost right after PSI (day 1–day 30), but few patients can loose it up to one year later (Kono et al. 2010; Miyazawa et al. 2012).
Factors predicting seed loss are the number of needles, number of seeds, preoperative prostate volume or expertise of the brachytherapy team (Miyazawa et al. 2012; Kono et al. 2010; Sugawara et al. 2009; Taussky et al. 2012).
Dosimetry is not significantly altered, when only a few seeds are lost (Sugawara et al. 2011; Taussky et al. 2012; Stone and Stock 2005).
Unfortunately, one patient experienced a secondary small cell lung cancer 10 years after PSI with detection of the seed in middle of the pulmonary tumor (Chen et al. 2012).
One study compared stranded seeds versus loose seeds. While loose seeds migrate preferably in the lung, stranded seeds are mostly excreted with urine (Saibishkumar et al. 2009). Surprisingly, overall seed loss was more frequent in the patient group with stranded seeds, which might be biased by low patient number (n = 40) or wrong technique. Another randomized study with 64 patients reported seed loss tendentially twice as often in patients with loose seeds (Reed et al. 2007).
However, it is necessary to distinguish between the implantation of single seeds in “safer” areas, like the centers of both prostate lobes, which can be clearly identified in real time ultrasonography. “Unsafe” areas like the peripheral prostate capsule, periprostatic venous system (Stone and Stock 2005) or the direct adjacent parts of the base of bladder or intraprostatic urethra may lead to a higher risk for seed loss.
As standard, the seed size is approximately 4.5 mm in length and 0.8 mm in diameter. When a thinner seed model is used, the edema after implantation might be reduced, which may result in lower toxicity. On the other hand, visibility in ultrasound might be impaired. Roberts et al. demonstrated that the main advantage of thinner seeds is a higher resolution in postplanning-CT, which leads to better visual separation of closely spaced seeds (Roberts et al. 2012). Of notice, seed loss to the lung seems not to depend on seed diameter (Wong and Sylvester 2012).
Some authors compared dosimetrical values of stranded vs. loose seeds. Due to the fact, that the natural prostate length is not a multiple of 1 cm (distance between stranded seeds), differences are to be expected. Therefore stranded seeds have a trend toward lower D90 and V100 values (Reed et al. 2007; Saibishkumar et al. 2009).
Obviously, with loose seeds the isodose contour might enwrap the prostate in a smoother way. But when larger treatment margins are indispensable (e.g. in intermediate-risk groups), this might be achieved easier and safer with stranded seeds in the outer periphery of the prostate gland than with single seeds (or with higher activity). Unfortunately, there are no studies evaluating this issue.
In some areas of the prostate, single seeds are advantageous. Due to the natural bending of the intraprostatic urethra, the use of stranded seeds in the center of the prostate may carry the risk of an overdosage of the middle urethra. In this case, single seeds might be placed in the apical or basal region. To prevent seed lost, single seeds with both-sided attached spacer material may be used.
Langley and Laing (Langley and Laing 2012) presented a new real-time technique called “4D Brachtherapy”. This technique uses a combination of stranded seeds around the periphery of the prostate gland and loose seeds within the central area. The planning procedure is based on simple ultrasound based measurements, and the number of stranded and single seeds is calculated by a nomogram. During the implant, the precalculated nomogram-based plan is transferred to the patient, and dosimetry is recalculated and optimized. Single seeds are used to bring dosimetry to perfection. The authors commend their technique, because it’s fast and less time consuming than common real time methods. Second, 4D-Brachytherapy is causing lesser edema, and is reliable and easy adjustable to irregular-shaped glands. The risk of migration is lower, because loose seeds are not placed near the venous plexus, and the dosimetry is beneficial, for the reason that some stranded seeds can be used just outside the capsule (Langley and Laing 2012).
7.2 Postplanning
Approximately one month after PSI, volume changes of the prostate from temporary prostatic edema have diminished. At this time, post-implant quality assurance computed tomographic scans (QA-CT) are recommended to verify proper dosimetry (Davis et al. 2012). Alternatively, QA-CT is performed on day 0 or day 1 after implant.
The ideal moment to perform the CT depends on the type of isotopes as well. For I-125 and Pd-103 implants with longer half-life period, 1 month interval has proven adequate. For Cs-131, with a half-life decay of 9.69 days, the day 14 QA-CT may be most accurate (Smith et al. 2012).
On CT images, prostate gland contours are often obscured by metallic seed artefacts and edema. Accurate prostate contouring is a challenging work, and small inaccuracies may lead to distinct deviation of dosimetry values such as D90 and V100. Experienced brachytherapists may interpret contours of prostate gland more precise, but still interobserver variations in D90 between 9.3 and 30.3 Gy are reported in the literature (Crook et al. 2002b). Another study of the same group revealed a systematic overestimation of CT-contoured prostate volume. Apparently, adjacent normal tissue is erroneously designated as prostate, especially in basal and apical regions (Petrik et al. 2012; Maletz et al. 2012).
When possible, an MRI-CT fusion should be employed to verify prostate contours post implant. But the technique of MRT-CT fusion is also prone to errors, since clear landmarks may be absent (seeds are hardly visible in T2). Special MRT sequences (T2, contrast-enhanced T1-weighted images) should first be established and optimized in close collaboration with the radiologists (Ohashi et al. 2012; Bowes et al. 2013b).
Fortunately, processing seed reconstruction results only in few mistakes and interobserver varability, when CT-MRT fusions are used (De Brabandere et al. 2012).
If MRT is not available, fusion of preimplant TRUS with 1-month postimplant CT appears as a reasonable alternative for the postplanning procedure (Bowes et al. 2013a).
Publications with detailed information about suboptimal dosimetric postplanning results are very rare. Keyes et al. classifies three groups of implant quality: a post-implant-V100 of >85 % is “good”, whereas a V100 of 75–85 % is “suboptimal” and a V100 of <75 % is “poor”. For the last 400 patients of a vast PSI program, the percentage of good, suboptimal and poor implants were 88, 10, and 2.3 %, respectively. Only 0.7 % of patients underwent a second top-up implantation (Keyes et al. 2004).
In an Australian data set of 255 patients, 6 patients (2.4 %) were identified with suboptimal implant reasoned in equipment/technical problems, patient movement during implantation or extensive post-implant edema. Those patients underwent re-implantation. In this publication, implant quality was defined as a D90 of ≥145 Gy (“good”), 145 Gy > D90 ≥ 130 Gy (“adequate”), 130 Gy > D90 ≥ 110 Gy (“potentially acceptable”) and an “inadequate” D90 < 110 Gy (Marcu and Lawson 2013).
7.3 Salvage Brachytherapy After Radical Prostatectomy
Treatment for patients with evidence of local recurrence after radical prostatectomy usually consists of EBRT. There are only few studies with low patient numbers concerning PSI as salvage option. All of them presupposed a biopsy proven local recurrence after radical prostatectomy, which had to be clearly recognizable on ultrasound images. As a first side note, sensitivity and specificity of digital rectal examination (DRE) and TRUS are 72.4 and 64.8 % versus 86.2 and 53.5 %, respectively. Biopsy is most probably negative, when PSA <0.5 ng/ml and TRUS / DRE are unsuspicious (Naya et al. 2005). Second side note, most local recurrences after surgery occur at the anastomotic site (56–76 %), bladder neck (26 %), retrovesical space (4–33 %) and more than one site (14 %) (Leventis et al. 2001; Nguyen et al. 2012; Liauw et al. 2012). In endorectal MRI, recurrence was seen in 37 % of men with a PSA >0.3 ng/ml versus 13 % if the PSA is ≤0.3 ng/ml (Liauw et al. 2012).
In a Spanish survey 42 patients were treated with low dose rate brachytherapy (145 Gy) in the anastomotic region. Five-year biochemical disease free survival was 88.6 % and cancer-specific survival was 97 %. No Grade 3 or 4 gastrointestinal and genitourinary toxicity has been described (Gomez-Veiga et al. 2012). In a case report published by Gaztañaga and Crook, dose escalation with combined external beam (46 Gy) and low-dose (LDR) brachytherapy (115 Gy) was presented as a feasible treatment option (Gaztañaga and Crook 2012).
7.4 Salvage Brachytherapy After EBRT
Radical prostatectomy, cryosurgery and brachytherapy are current salvage treatment options for prostate cancer recurrence after primary radiation therapy.
In a Dutch study, a total of 129 patients with biopsy proven recurrence after primary EBRT or PSI were retrospectively analyzed. Biochemical failure (PSI, cryosurgery: Phoenix, surgery: PSA > 0.1 ng/ml) occurred in 81, 61 and 66 % of men treated with salvage LDR-brachytherapy, salvage cryosurgery, or salvage surgery, respectively. In all salvage therapy groups, 30 % of men suffered from grade >3 genitourinary and gastrointestinal toxicity, therefore patients should be selected with care before offering salvage options (Peters et al. 2012).
High toxicity is expectable, when seeds are placed too close to the rectal wall or the urethra. One method of minimizing these problems is to use an intraoperative dosimetry and planning system (Stock 2004). The poor outcome of local salvage therapy is explainable with a lack of diagnostic tools to discriminate between patients with truly localized recurrence, and those with presence of microscopic disseminated disease.
Aaronson et al. presented a very plausible proceeding. The prescribed dose to the recurrent cancer site (determined by magnetic resonance spectroscopy and biopsy results) was 144 Gy, while 108 Gy were delivered to the remaining prostate gland. With this approach, no notable severe complications were reported, and after 30 months, cancer-free survival was 96 % and biochemical relapse-free survival was 88 % (Aaronson et al. 2009).
A prospective phase II trial of the radiation therapy oncology group (RTOG 0526) was activated in 2007 and closed to accrual on January 2014.
One hundred Patients after primary EBRT of T1-T2c, GS 2-7, PSA ≤20 ng/ml prostate cancer, a local recurrence >30 months after EBRT and a current PSA <10 ng/ml were to be included. The treatment concept involved a PSI with 140 Gy encompassing the PTV, which was defined as the prostate CTV plus margin (anterior, lateral 2–3 mm, posterior 0 mm, cranial and caudal 5 mm) (Crook 2007). The results are expected with attention.
8 Dosimetry
Guidelines for dose selection involve the intraoperative planning and the postplanning procedure. For seed order, the seed number is estimated preoperatively by performing an ultrasound examination with computer planning (“Pre-Plan”), which should give the best results. Alternatively, seed number can be taken from a look-up table based on inhouse analyses or published literature (Cohen et al. 2002).
8.1 Seed Quantity
The number of seeds depends from the size of the prostate and single seed activity used. Usually, nomograms are used to determine the approximate seed count based on prostate volume, and additional 5–10 % are added for uncertainties. When loose seeds are implanted, the used total activity may be 15–23 % higher in comparison to stranded seeds (Kudchadker et al. 2008). Somehow, this effect may be caused by three-dimensional reasons: stranded seeds are allowed to be placed near the surface of the prostate capsule and loose seeds must be placed deeper, while both have to deliver enough dose to the PTV surface.
8.2 Seed Activity and Total Activity
Most centers use low activity of seeds (0.4 mCi per seed), but there is no consensus regarding optimal seed activity. By experience, low activity might beneficial in smaller glands, whereas higher activity (0.6 mCi) reduces the number of needles in larger prostates and possibly the procedure costs. In fact, seed implants with a higher source strength seem to improve the probability of excellent implant quality due a better dose coverage, while there are no differences in the rectal and urethral doses between high- and low activity seeds (Narayana et al. 2005). Another study has shown that neither dose homogenity nor conformality is compromized with a lower source strength (Thomas et al. 2007). However, finally the own experience determines which activity of seed is used.
8.3 EBRT Combined with PSI
If EBRT is combined with PSI (110 Gy), the prescribed dose for EBRT is in the range of 41.4–50.4 Gy (1.8 Gy–2 Gy/d). Neither the optimal sequencing of PSI and EBRT is clear, nor the ideal time interval. PSI before EBRT possibly exposes tissues to radiation simultaneously, but EBRT dose may be adjusted in inadequate dosimetrical results of brachytherapy. Secondly, in times of image guidance, the implant may be used as well-identifiable landmark. Indeed, current practice and clinical trials favor EBRT followed by PSI (RTOG 2011; Bittner et al. 2012; Merrick et al. 2011).
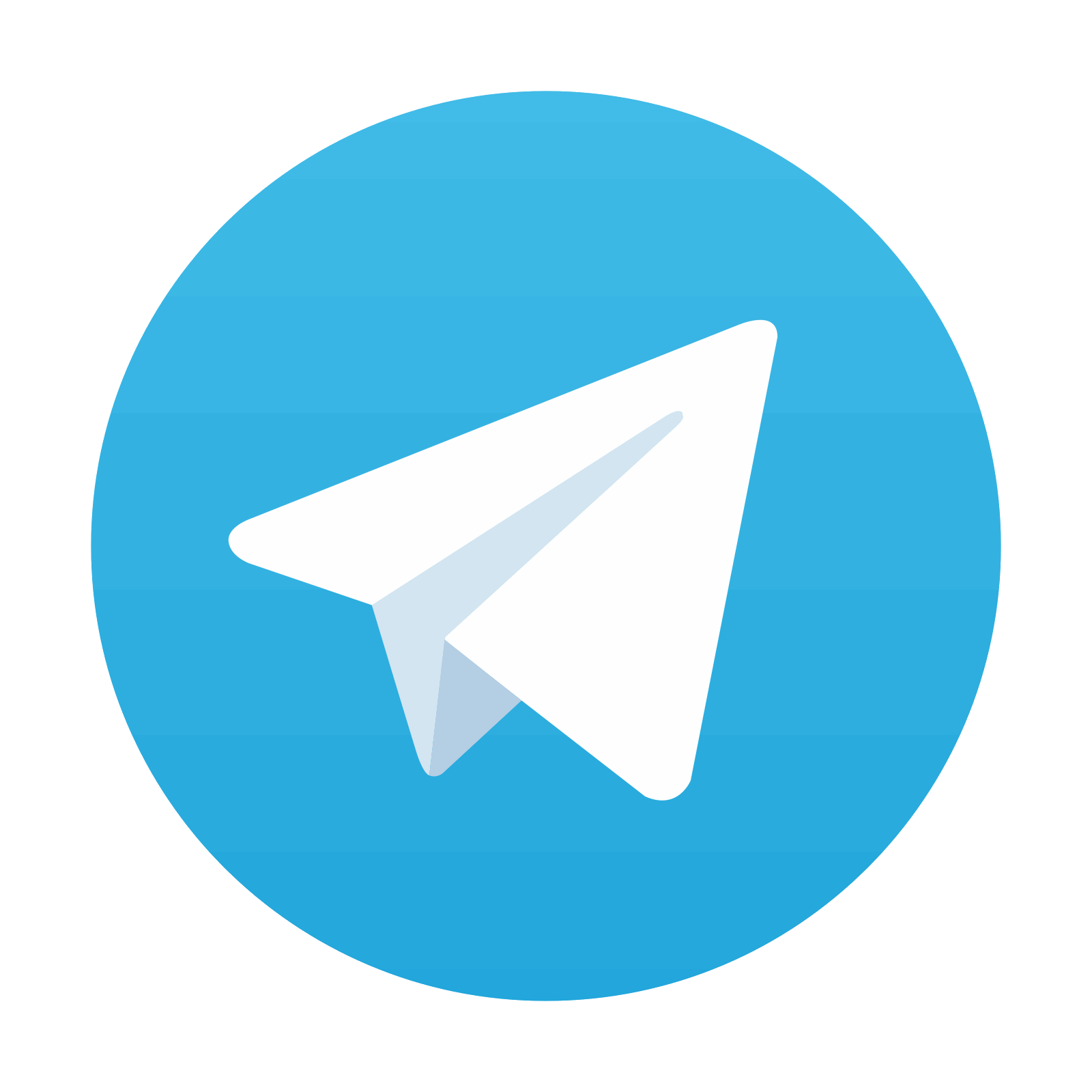
Stay updated, free articles. Join our Telegram channel

Full access? Get Clinical Tree
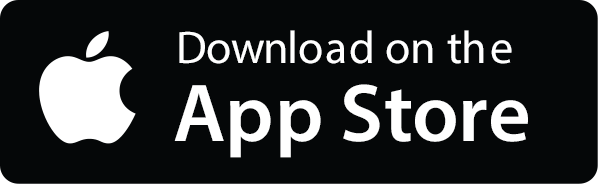
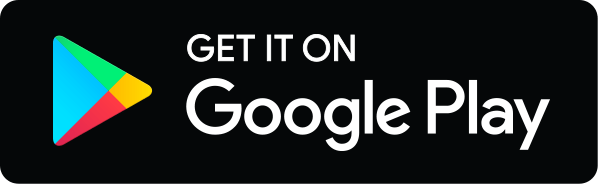