Abstract
This chapter covers the radionuclides, techniques, and indications for musculoskeletal nuclear medicine imaging. Also included are examples of normal and abnormal images for commonly encountered clinical issues such as trauma, neoplasms, infection, and other benign conditions. Bone mineral density applications and therapy for osseous metastases is included.
Keywords
bone scan, skeletal trauma, osseous metastases, benign bone conditions, soft tissue uptake, bone mineral density, therapy of osseous metastases
Chapter Outline
Palliative Therapy of Painful Osseous Metastases
The ready availability of cost-effective technetium-labeled bone-seeking radiopharmaceuticals allows the widespread use of bone scanning for both regional and whole-body skeletal assessment in the evaluation of a variety of benign and malignant disease states. The bone scan often provides an earlier diagnosis and demonstrates more lesions than are found by planar radiographic procedures. Although the presence of a lesion on a bone scan is nonspecific, its monostotic or polyostotic status and anatomic distribution can usually be determined, and these findings often provide important clues to the differential diagnosis, as well as determining the need for further imaging by computed tomography (CT) or magnetic resonance imaging (MRI). For optimal performance of bone scans, both the physician and the technologist need to understand the limitations and uses of skeletal imaging procedures.
Chapter Outline
Palliative Therapy of Painful Osseous Metastases
The ready availability of cost-effective technetium-labeled bone-seeking radiopharmaceuticals allows the widespread use of bone scanning for both regional and whole-body skeletal assessment in the evaluation of a variety of benign and malignant disease states. The bone scan often provides an earlier diagnosis and demonstrates more lesions than are found by planar radiographic procedures. Although the presence of a lesion on a bone scan is nonspecific, its monostotic or polyostotic status and anatomic distribution can usually be determined, and these findings often provide important clues to the differential diagnosis, as well as determining the need for further imaging by computed tomography (CT) or magnetic resonance imaging (MRI). For optimal performance of bone scans, both the physician and the technologist need to understand the limitations and uses of skeletal imaging procedures.
Anatomy and Physiology
Bone is made of an inorganic mineral phase of crystals bound to protein, largely collagen. The crystals consist of a composite of calcium, phosphate, and hydroxyl ions, called hydroxyapatite. Normal bone is in a constant state of remodeling accomplished by osteoclasts resorbing old bone and osteoblasts putting down new bone to mold the bones into shapes and thicknesses that adapt to the recurring mechanical stresses of daily life. This process of “bone turnover” may also be elicited or accelerated by pathologic conditions such as fractures, infections, bone tumors, or metastases. Anatomically, the skeleton is composed of two parts: the axial and the appendicular portions. The axial skeleton includes the skull, spine, and shoulder girdle. The appendicular skeleton includes the upper extremities, pelvis, and lower extremities. This is an important distinction because some diseases favor either the appendicular or the axial skeleton.
Radiopharmaceuticals
Bone-seeking radiopharmaceuticals are analogs of particular components of the mineral portion of bone, hydroxyapatite calcium, hydroxyl groups, or phosphates. By far, the most widely used radiopharmaceuticals for skeletal imaging are technetium-labeled diphosphonates ( 99m Tc-MDP), most often methylene diphosphonate. However, over the past decade, use of the positron emission tomography (PET) imaging agent, fluorine-18 sodium fluoride ( 18 F-NaF), has been increasing in clinical practice. When injected intravenously, these tracers distribute in the skeleton based on differences in regional perfusion and bone turnover, as well as other factors. The primary bone uptake mechanism is by adsorption (chemisorption) onto the bone surface and subsequent incorporation into the structure of hydroxyapatite. In some patients, this uptake can be affected by administered medications.
Diphosphonates serve as a phosphorus analog in the mineral phase of bone. They contain organic P–C–P bonds that are resistant to enzymatic hydrolysis and thus are stable in vivo. Because the diphosphonates have rapid renal excretion, they provide a high target-to-nontarget ratio in 3 to 4 hours after injection, with 50% to 60% of the activity localizing in bone and the remainder being cleared by the kidneys. Factors that impair renal function result in increased soft-tissue activity, which reduces the quality of the bone scan.
While the initial accumulation of technetium-labeled radiopharmaceuticals in bone is primarily related to blood supply and osteoblastic activity, roles are also played by capillary permeability, the local acid-base relation, fluid pressure within bone, hormones, vitamins, the quantity of mineralized bone, and bone turnover. Increased radionuclide activity in bone may result from accentuation of any one of these factors. Factors that may be responsible for greater than usual activity are listed in Boxes 8.1 and 8.2 . For example, regionally increased blood flow causes increased delivery of the radiopharmaceutical to the bone, with resultant increased regional deposition of the agent. The converse is also true: interference with any of these factors may cause decreased skeletal activity. For instance, in cases of decreased cardiac output, bone scans may be of poor quality, owing to inadequate delivery of the radiopharmaceutical to the bone.
Increased osteoid formation
Increased blood flow
Increased mineralization of osteoid
Interrupted sympathetic nerve supply
Localized
Primary bone tumor
Metastatic disease
Osteomyelitis
Trauma
Stress or frank fractures
Physical abuse (nonaccidental trauma)
Postsurgical osseous changes
Loose prosthesis
Degenerative changes
Osteoid osteoma
Paget disease, melorheostosis, fibrous dysplasia
Arthritis
Locally increased blood flow
Hyperemia
Decreased sympathetic control
Decreased overlying soft tissue (e.g., postmastectomy)
Soft-tissue activity (see Box 8.5 )
Generalized (Superscan)
Primary hyperparathyroidism
Secondary hyperparathyroidism
Renal osteodystrophy
Diffuse metastases from
Prostate
Lung
Breast
Hematologic disorders
Fluorine-18 sodium fluoride ( 18 F-NaF) is an analog for the hydroxyl ion in the bone matrix. The fluoride ion exchanges for a hydroxyl ion on the surface of the hydroxyapatite matrix of bone to form fluorapatite and migrates into the crystalline matrix of bone, where it is retained until the bone is remodeled. Initial uptake has high first-pass extraction efficiency under most circumstances, approaching 100%, and with only 10% remaining in the plasma at 1 hour. This is considerably higher than diphosphonates. These properties, along with a reasonable decay half-life (110 minutes), result in a very favorable bone-to-background tissue ratio within 1 hour after 18 F-NaF intravenous administration, allowing for imaging considerably earlier than with technetium-99m ( 99m Tc) diphosphonate. Fluorine-18 sodium fluoride was initially approved for bone scanning by the US Food and Drug Administration (FDA) in 1972 but remains under consideration for reimbursement by Medicare for PET bone imaging. With the advent of fast PET/CT scanners and local cyclotron production of 18 F, the methodology has become practical. The quality of the PET/CT 18 F-NaF bone scans is significantly better than 99m Tc-methylene diphosphonate (MDP) in terms of spatial and anatomic resolution, has been shown to be more sensitive and specific in some clinical settings, and allows for quantitative assessment of bone metabolism, if needed. A significant drawback for 18 F-NaF PET/CT scanning is that it is significantly more expensive and has a radiation dose about seven times higher than that of 99m Tc-MDP scans.
Because both 99m Tc-MDP and 18 F-NaF are excreted by the kidneys by glomerular filtration (with some NaF tubular reabsorption), adequate patient hydration before and after administration is advisable to reduce absorbed radiation doses to the bladder and kidneys.
Technique
Technical aspects, sample protocols, and dosimetry for planar and single-photon emission computed tomography (SPECT) and PET/CT skeletal imaging are presented in Appendix E .
For routine planar scans, the patient is normally injected intravenously with 10 to 20 mCi (370 to 740 MBq) of the technetium diphosphonate radiopharmaceutical and imaged 2 to 4 hours later. The site of injection should be distant from any suspected osseous pathology and should be recorded. Often, even a slight extravasation of isotope at the injection site causes a focus of markedly increased soft-tissue activity. In patients suspected of having either osteomyelitis or cellulitis, a radionuclide angiogram and initial blood pool image are performed after injection, and routine images are obtained at about 2 to 3 hours. This is termed a three-phase study . Sometimes, additional images are performed 18 to 24 hours after injection (four-phase study) . A four-phase study is rarely needed but can be useful in patients in renal failure who have poor soft-tissue clearance.
Gamma camera imaging usually employs a moving table that results in whole-body images. If multiple spot images are obtained, the entire skeleton should be imaged. The patient is normally scanned in both the anterior and posterior projections. Detailed spot views of particular regions may be obtained as dictated by patient history or symptoms. In addition, selective pinhole or high-resolution collimator views allow for enhanced resolution in any areas of interest. These are especially useful when imaging small bones and pediatric patients.
The rapid urinary excretion of phosphate radiopharmaceuticals causes large amounts of activity to accumulate within the bladder, which may obscure pelvic lesions; therefore voiding before imaging should be routine. Voiding, however, particularly in incontinent patients, may result in radioactive contamination of skin or clothing; this may obscure underlying pathology or mimic a lesion. Removal of contaminated clothing and cleansing of skin may be necessary to obtain accurate results. After injection and before scanning, patients should be hydrated. The resultant more frequent voiding decreases the bladder and pelvic radiation dose.
SPECT imaging may significantly improve skeletal lesion detection in patients with specific regional complaints and may establish or better localize an abnormality suspected on routine planar images. SPECT is most valuable in complex bony structures, such as large joints, the spine, and the pelvis.
The technique for 18 F-NaF PET/CT scans includes intravenously administered activity of about 20 mCi (740 MBq). Whole-body scanning is done 1 hour post injection, and the CT scan is done without the use of intravenous or gastrointestinal contrast. Limited body scanning is sometimes done for suspected focal entities, such as osteomyelitis. Scans are typically reviewed and interpreted on a workstation, which allows viewing rotating images and also provides automatic fusion of the PET and CT data.
Normal Scan
The normal scan ( Fig. 8.1 ) varies significantly in appearance between children and adults. In children, areas of growth in the region of the epiphyses show intense radionuclide accumulation ( Fig. 8.2 ). In adults, the quality of the bone scan can be related to age; in general, the older the patient, the higher the proportion of poor-quality scans. There usually is good visualization of the skull, with relatively increased accumulation of activity in the region of the nasopharynx, which may be secondary to the high proportional blood flow in this region. Activity in the skull is often patchy, even in normal patients, so care must be taken in assessing skull lesions without an accompanying radiograph. Often, there is focal maxillary or mandibular alveolar ridge activity in adults, owing to dental disease. There is activity throughout the spine, and it is common to see focal areas of increased activity in the lower cervical spine even on anterior images, usually representing degenerative changes or simply a result of the lordosis of the cervical spine rather than activity in the thyroid cartilage or the thyroid itself. Areas of tendon insertion, chronic stress, and osseous remodeling caused by any reason also demonstrate increased activity. On the anterior view, there is prominent visualization of the sternum, sternoclavicular joints, acromioclavicular joints, shoulders, iliac crests, and hips. Increased activity in the knees in older patients is relatively common because of the propensity for arthritic changes. On the posterior view, the thoracic spine is well seen, as are the tips of the scapulae. The spine often demonstrates increased activity in areas of hypertrophic degenerative change, and the sacroiliac joints are usually pronounced.
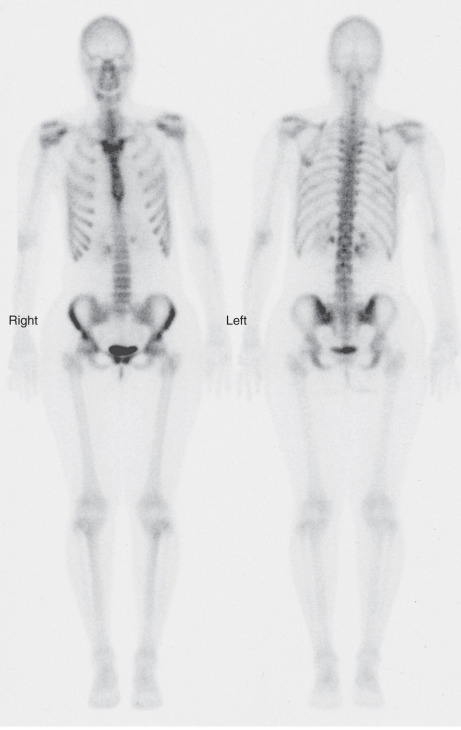
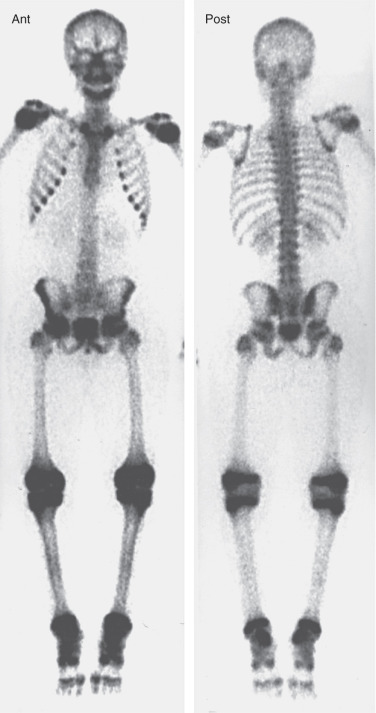
Because the human skeleton is symmetric, any asymmetric osseous activity should be viewed with suspicion. In addition, it is important on the posterior view to examine the scan for the presence and location of renal activity; on the anterior view, for bladder activity. The kidneys and bladder should be routinely scrutinized for focal space-occupying lesions producing photopenic defects in the renal cortex or displacement of the kidneys or bladder. Asymmetric renal activity is not uncommon. Because the scans are usually obtained in the supine position, activity may accumulate in extrarenal pelves. If urinary tract obstruction is suspected, kidney views should be repeated after the patient has ambulated to distinguish obstruction from position-related collecting system activity ( Fig. 8.3 ).
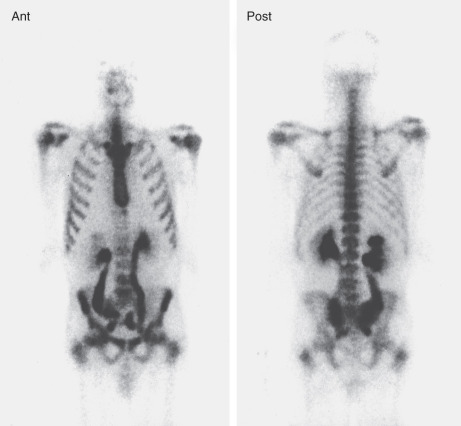
If there is extravasation of the radiopharmaceutical at the site of injection, the radiopharmaceutical will be slowly resorbed. In such cases, lymphatic drainage may also occur, resulting in the visualization of one or more lymph nodes, not infrequently seen in the axilla or supraclavicular region on the side of an upper extremity injection ( Fig. 8.4 ).
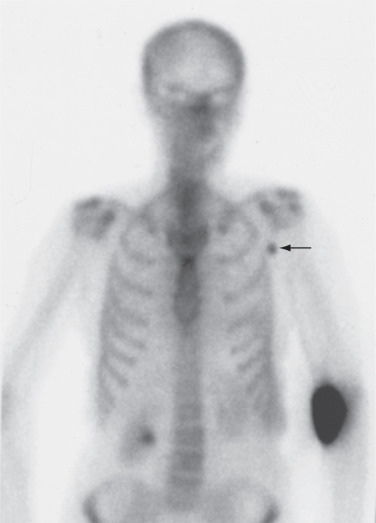
Localized areas of increased soft-tissue or skeletal activity in an extremity distal to the site of injection (the glove phenomenon ) may be a result of inadvertent arterial injection of the radionuclide. Regional blood flow changes may also be reflected in the scan (either relative ischemia if the activity is decreased, such as with atherosclerotic disease or gangrene, or hyperemia if the activity is locally increased, such as with cellulitis or other inflammation). When pathology is suspected in the hands, wrists, or forearms, and a three-phase study is being performed, it is important to release the venous tourniquet and wait for about 1 minute before injecting the radiopharmaceutical. If this is not done, there can be confusion of actual pathology, with transient hyperemia resulting from vasodilatation caused by the tourniquet ( Fig. 8.5 ). Differential blood flow also may be secondary to neurologically or autonomically mediated abnormalities (sympathectomy or neuropathy), or even to altered stress.
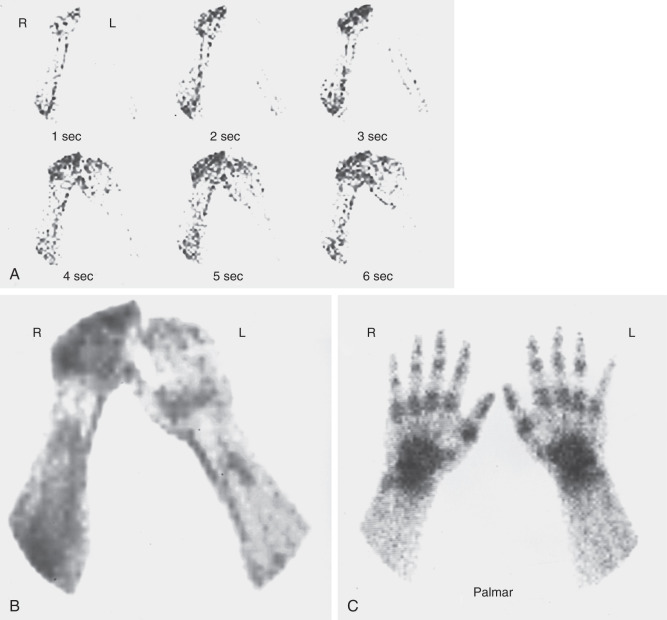
Recognition of the details of normal imaging anatomy becomes even more important when SPECT images of specific skeletal regions are obtained. Reviewing the images in three orthogonal planes generally aids interpreter orientation and thus allows more accurate localization of pathology. The specific reconstructions of greatest value depend on the area being evaluated. The complexity of the spine makes it particularly amenable to SPECT imaging to localize an abnormality in the vertebral body, disc space, or posterior elements. Transverse images of the spine resemble those of CT sections, whereas coronal and sagittal SPECT images are analogous to anteroposterior and lateral radiographic tomograms, respectively. The curvature of the thoracolumbar spine results in sequential rather than simultaneous visualization of the anatomic parts of adjacent vertebrae. With a careful view of the sequential images on a computer monitor display, proper orientation generally is not difficult.
Clinical Applications
The following are some common indications for bone scanning:
- •
Detection and follow-up of skeletal metastases
- •
Differentiation between osteomyelitis and cellulitis
- •
Determination of bone viability: infarction or avascular necrosis
- •
Evaluation of fractures difficult to assess on radiographs (stress fractures, fractures of complex structures, and possible fractures in children who are physically abused)
- •
Evaluation of prosthetic joints for infection or loosening
- •
Determination of biopsy site
- •
Evaluation of bone pain in patients with normal or equivocal radiographs
- •
Evaluation of the significance of an incidental skeletal finding on radiographs
Metastatic Disease
The wide availability and high sensitivity of radionuclide bone imaging in determining the presence and the extent of metastatic disease makes it an extremely important tool in disease staging and treatment decisions ( Fig. 8.6 ). This may prove of particular value in the detection of lesions in critical weight-bearing areas, such as the femur. A primary strength of whole-body bone scanning is its ability to survey the entire skeleton on a single study.
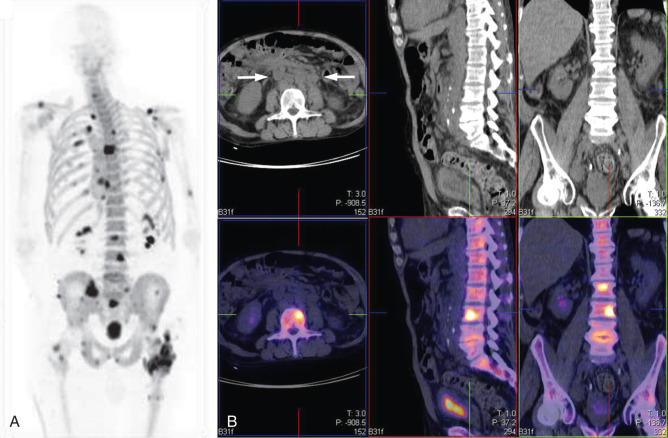
For a lytic lesion to be visualized by radiography, localized demineralization of about 30% to 50% must occur, whereas only a 5% to 10% alteration in the ratio of lesion to normal bone radiotracer activity is necessary to identify an abnormality on bone scan. Thus there is little question that bone scans usually demonstrate metastatic lesions much earlier than radiography does. Further, the false-negative rate of radiographic skeletal surveys may be as high as 50% with certain tumors, whereas the overall false-negative rate of bone scanning for the most common neoplasms may be as low as 2%. Comparing 99m Tc-diphosphonate planar bone imaging with 18 F-NaF PET imaging, most studies have shown a clear advantage of 18 F-NaF PET/CT in terms of sensitivity (~ 100%) and specificity (97%) compared with conventional 99m Tc-MDP planar bone imaging with or without SPECT with sensitivity of 70% (92% with SPECT) and specificity of 57% (82% with SPECT). Clearly, regardless of the radiopharmaceutical used, cross-sectional imaging and hybrid imaging with CT providing structural assessment adds to both the sensitivity and specificity of bone imaging in this setting.
Some tumors are more likely than others to produce a false-negative bone scan. These include highly aggressive anaplastic tumors, reticulum cell sarcoma, renal cell carcinoma ( Fig. 8.7 ), thyroid carcinoma, histiocytosis, neuroblastoma, hepatocellular carcinoma, and especially multiple myeloma. Because the typically lytic lesions of multiple myeloma are characterized by decreased vascularity and low osteoblastic activity, bone-seeking radiopharmaceuticals have a low sensitivity for the early detection of bone lesions, with a false-negative rate as high as 50%. When multiple myeloma is seen on a bone scan, it is often secondary to a pathologic fracture or impending fracture. In general, 18 F-fluorodeoxyglucose (FDG) has higher sensitivity for lytic lesions than bone-seeking tracers and has been shown to have early sensitivity for marrow metastases.
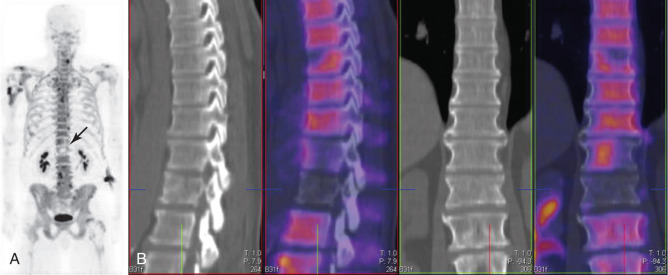
About 80% of patients with known neoplasms and significant bone pain have metastases documented by the bone scan. Because 30% to 50% of patients with metastases do not have bone pain, a good case may be made for scanning patients with asymptomatic tumors that have a propensity to metastasize to bone (e.g., breast, lung, and prostate); however, the procedure may not be cost-effective for tumors with low rates of osseous metastases (e.g., colon, cervix, uterus, head, and neck).
Although most metastases are multiple and relatively obvious, there are times when the interpretation may be difficult. If a single lesion is identified, the false-positive rate for attributing the finding to a metastasis is high. Only about 15% to 20% of patients with proven metastases have a single lesion (most commonly in the spine). A single focus of increased activity elsewhere is often secondary to benign disease, especially in a rib where this is attributable to metastasis in only about 10% of cases. A notable exception to this is a single sternal lesion in a patient with breast cancer ( Fig. 8.8 ), which can be a result of metastasis in almost 80% of cases. In a patient with a known malignancy and a solitary abnormality on bone scan without an obvious benign explanation on radiographs, additional imaging is often warranted to exclude the possibility of metastases. If two consecutive ribs are involved by adjacent discrete foci of increased activity, the lesions are almost always secondary to trauma. When multifocal areas of increased activity are seen in noncontiguous ribs, especially if in a linear configuration along the rib, the likelihood of metastatic disease is high. In the spine, multiple foci of linear activity in the vertebral bodies more likely suggest osteoporotic fractures. Lesions extending into the posterior elements or involving the vertebral pedicle are more likely metastatic.
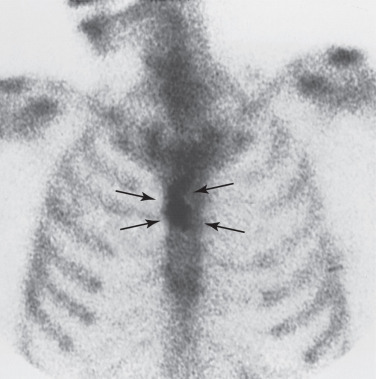
In multifocal metastatic disease, the regional distribution of lesions for common bone-seeking primary tumors is as follows: thorax and ribs, 37%; spine, 26%; pelvis, 16%; limbs, 15%; and skull, 6%. The reason for this distribution is that most bone metastases are caused by hematogenous spread to the red marrow, with subsequent erosion of the surrounding bone.
Follow-up bone scans to assess response in patients undergoing treatment for advanced breast and prostate cancer should be interpreted with caution. Whereas 18 F-FDG measures tumor metabolism (which usually decreases with treatment success), bone-seeking tracers may provide an indirect indication of tumor improvement through repair of the adjacent bone; this response may substantially lag tumor response. Contrarily, within the first 3 months of chemotherapy, a favorable clinical response by focal bone metastases may result in avid bone healing through active osteoblastic repair that causes increased uptake at involved sites, known as the flare phenomenon , which is usually a good prognostic sign. However, if not clinically correlated, this response can give the false impression of new lesions that were too inactive to be seen previously or the extension of existing metastatic sites. This flare response may be seen as early as 7 to 10 days after successful hormonal therapy in osseous metastatic disease from breast and prostate cancers and usually lasts for approximately 6 months. New bone lesions that appear 6 months or later after treatment or an interval increase in activity or the extent of existing lesions suggests disease progression.
Diffuse involvement of the skeleton by metastases can be deceptive; it may initially appear as though there has been remarkably good, relatively uniform uptake in all of the bones. This has been referred to as a superscan ( Fig. 8.9 ). A hallmark of the superscan caused by metastases is significantly decreased renal activity with diffusely increased activity noted throughout the axial skeleton. A superscan is most commonly a result of prostatic carcinoma, although diffuse metastases from other tumors, such as breast cancer and lymphoma, may also cause this appearance. In the absence of neoplasm, a superscan involving bones throughout the entire skeleton (both axial and peripheral) should raise suspicion of metabolic conditions, such as primary or secondary hyperparathyroidism. Increased activity primarily in the peripheral skeleton may be seen in hematologic disorders. For example, a patient with chronic anemia, such as occurs in sickle cell disease or thalassemia, may show increased activity in the skull and around the knees and ankles as a result of expanded marrow and an accompanying increase in blood flow to these regions.
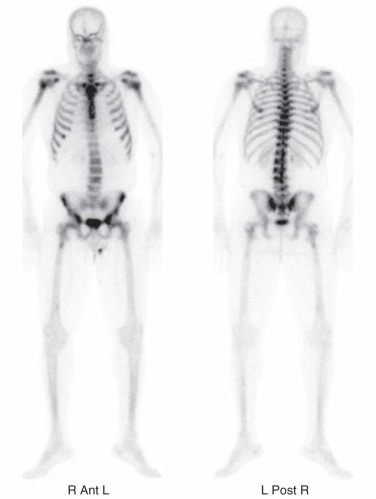
In some patients who have metastatic disease, chemotherapy results in immunosuppression, and treatment may involve the use of granulocyte-macrophage colony-stimulating factor. This treatment causes marrow hyperplasia and increased marrow blood flow, resulting in bone scans with symmetrically increased activity around the major joints (particularly the knees). This may also be seen on 18 F-FDG PET imaging.
In searching for metastatic disease, it is important not only to delineate the areas of increased activity (see Box 8.2 ), but also to look for cold lesions ( Box 8.3 ), which are usually much more difficult to identify. In cancer patients, focal photon-deficient lesions are attributable to metastatic disease in more than 80% of cases. They may occur if the tumor is extremely aggressive, if there is disruption of the blood supply to the bone, or if there is significant marrow involvement, particularly in a vertebral body. When multiple adjacent bones have a decreased radionuclide accumulation, other causes, such as radiation therapy, should be considered ( Fig. 8.10 ). Other causes of cold lesions include infarction (particularly in patients with sickle cell anemia) and avascular necrosis. Both infarction and aseptic necrosis in the healing phase can show increased activity.
Localized
Overlying attenuation artifact caused by pacemaker, barium, etc.
Instrumentation artifact
Radiation therapy
Local vascular compromise
Infarction
Intrinsic vascular lesion
Early aseptic necrosis
Marrow involvement by tumor
Early osteomyelitis
Osseous metastases from:
Neuroblastoma
Renal cell carcinoma
Thyroid carcinoma
Anaplastic tumors (e.g., reticulum cell sarcoma)
Cyst
Generalized
Older age
Inadequate amount of radiopharmaceutical
Chemotherapy
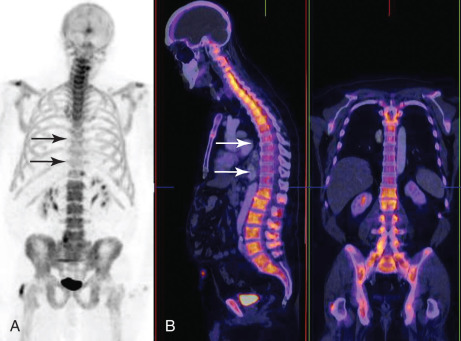
With the widespread use of 18 F-FDG PET/CT in the initial staging and follow-up of many common malignancies, the identification of any bone metastases is frequently established, such that bone tracer imaging may not be required for staging purposes. Further, the use of standardized uptake values (SUVs) for quantitation of metastatic lesions is showing to be reliable in the prognostic assessment of treatment response. In breast cancer , skeletal metastases are present in only 1% to 3% of patients with early stage disease (stages I and II), so that bone scans in asymptomatic patients are not generally recommended. However, in more advanced disease (stages III to IV), metastasis to bone ranges between 15% and 40% and is seen in up to one-third of patients with recurrent disease. Bone imaging may play a significant role in these patients in the absence of definitive 18 F-FDG PET imaging.
In non–small cell lung cancer (NSCLC), bone metastases are present in approximately 20% to 30% of patients at diagnosis. Some studies have shown that 18 F-FDG PET/CT has as high or higher sensitivity and specificity than bone scans for the detection of bone metastases, with an overall accuracy of 95% (versus 90%) and may well be the dominant modality for detecting bone metastases in NSCLC.
In prostate cancer , bone scans are highly sensitive in detecting the typically osteoblastic bone metastases from prostate cancer. Due to its availability and low cost, 99m Tc-MDP bone imaging has long been a staple in evaluation of a disease in which 18 F-FDG CT has not assumed a major role. SPECT and SPECT/CT can optimize the use of planar bone scintigraphy, with improved sensitivity ranging from 88% to 92% and an accuracy of 90%. 18 F-NaF PET/CT bone imaging has been shown to have superior sensitivity and specificity to 99m Tc-MDP imaging and is an excellent, but more expensive, alternative. The rate of positive bone scans is directly related to the prostate-specific antigen (PSA) value and the aggressiveness of the disease as assessed by lesion biopsy (Gleason score). In patients presenting with moderately or well-differentiated prostate cancer and PSA levels of ≤ 10 ng/mL, the likelihood of bone metastases is very low (less than 2%), such that in patients without skeletal symptoms, a staging bone scan is unnecessary. Further, in patients with PSA levels ≤ 20 ng/mL and Gleason scores < 8, positive bone scan results are also low (1% to 13%). However, patients with PSA levels ≥ 20 ng/mL, Gleason scores ≥ 8, or locally advanced disease should undergo radionuclide bone scans. In general, patients with significant regional skeletal symptoms should also be considered for bone scans, as should patients with PSA levels rising significantly from successful treatment baselines. It should be noted that PSA levels can be normal in patients with osseous metastases receiving hormonal therapy.
Malignant Bone Tumors
While radionuclide bone scans play a limited role in the imaging of primary bone tumors, these tumors may be incidentally encountered when imaging patients with regional bone pain or equivocal radiographic findings and may play a role in the evaluation of metastases in osteogenic lesions. The bone scan appearance of osteogenic sarcoma varies depending on the vascularity and aggressiveness of the tumor and on the amount of neoplastic and reactive bone production ( Fig. 8.11 ). Increased activity in these lesions is usually quite intense and often patchy with photopenic areas. Only about 2% of these patients present with osseous metastases from the primary site. Exact assessment of tumor extent by bone scanning is often complicated by reactive hyperemia in the affected limb, which may produce increased activity in the entire extremity. Evaluation of various bone tumors with 18 F-FDG PET/CT is discussed in Chapter 11 .
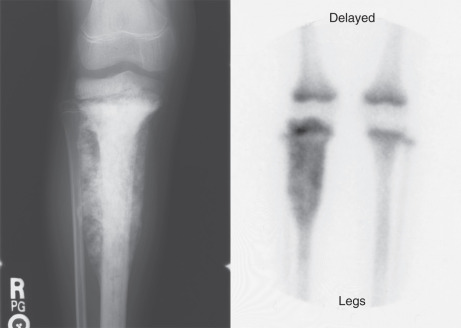
In the past, follow-up bone scans were not thought to be worthwhile in patients with osteosarcoma because pulmonary metastases almost always developed before osseous metastases. Aggressive chemotherapy, however, has altered the natural history of osteosarcoma, and now about 20% of patients develop osseous metastases before pulmonary disease. Because osteosarcomas are bone-forming lesions, soft-tissue metastases may be seen as foci of extraskeletal increased activity, especially in the lung and liver. In interpreting postsurgical bone scans, care must be taken not to mistake postamputation reactive changes at the amputation site for tumor recurrence.
Ewing sarcoma is a relatively common primary bone tumor, frequently occurring in the pelvis or femur. Activity is often intense and homogeneous. The tumor is very vascular and may mimic osteomyelitis on three-phase bone imaging. Up to 11% of patients present with osseous metastases ( Fig. 8.12 ). About 40% to 50% of patients with either Ewing sarcoma or osteosarcoma develop osseous metastases within 2 years of presentation, and follow-up bone scans may be useful. Reactive hyperemia producing increased activity in the adjacent uninvolved bone is not usually seen with Ewing sarcoma.
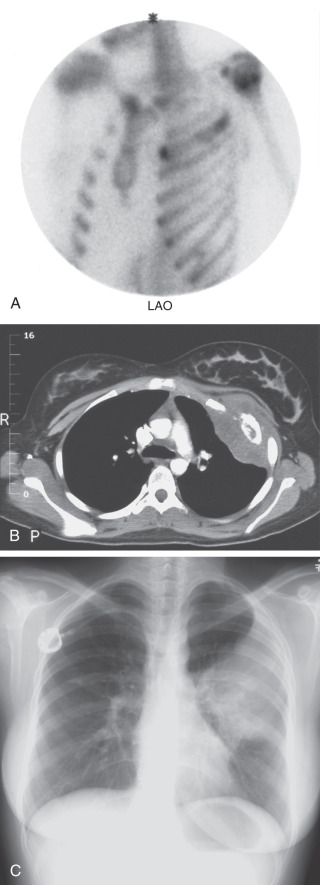
Benign Osseous Neoplasms
Benign osseous neoplasms have variable appearances on bone scan ( Box 8.4 ). Angiographic and blood pool images obtained shortly after injection of bone scanning agents indicate that most malignant lesions are hyperemic and that most benign lesions initially accumulate little radiopharmaceutical. An early blood pool image may therefore be helpful in identifying benign lesions, because it may show little or no increased uptake. The major exception to this is osteoid osteoma ( Fig. 8.13 ), which demonstrates intense activity at the site of the vascular nidus. Bone scans are often valuable in detecting these lesions when they occur in sites that are difficult to evaluate on a radiograph, such as the spine. On delayed images, various benign lesions show a wide range of activity. Osteoblastomas, osteoid osteomas, chondroblastomas, and giant-cell tumors usually have intense activity on delayed images. Enchondromas can have moderately or occasionally intensely increased activity ( Fig. 8.14 ), chondroblastomas are usually of intermediate activity ( Fig. 8.15 ), and fibrous cortical defects and nonossifying fibromas are of normal or near-normal intensity. Bone islands, hemangiomas, and cortical desmoid tumors rarely show increased activity and usually cannot be distinguished from normal bone. Uncomplicated bone cysts are usually cold centrally, but may have a mild rim of activity caused by increased bone remodeling. Bone cysts that undergo pathologic fracture due to excessive thinning of the bone may have significantly increased activity in the region of the fracture. Fibrous dysplasia is a benign disease of bone that may present as single ( Fig. 8.16 ) or multiple areas of variable increased activity. Both polyostotic fibrous dysplasia and Paget disease are sometimes confused with multifocal metastatic disease, although the distribution and radiographic presentation of lesions are frequently characteristic. Both osteochondromas and enchondromas are frequently seen as areas of increased activity.
