8
Skin Brachytherapy
Anna O. Likhacheva, Phillip M. Devlin, and Ivan M. Buzurovic
Radiotherapy as a scientific specialty was propelled forward at the turn of the 20th century, in large part due to the study of the effects of ionizing radiation on the skin. In 1897, Leopold Freund, a dermatologist, demonstrated the first successful therapeutic application of ionizing radiation before the Vienna Medical Society when he successfully treated a 5-year-old patient afflicted by hairy moles. Later, in 1901, in what may have been the first radiobiologic observation, French physicist Henri Becquerel reported a radiation burn on his chest after leaving a container with 200 mg of radium in his shirt pocket. In current practice, radiation therapy has become an effective therapy for malignancies of the skin, including nonmelanoma skin cancer (NMSC), cutaneous lymphoma, Kaposi sarcoma, Merkel cell carcinoma, and others.
NMSC is the most common malignancy in the United States and affects more than 2 million Americans annually (1). The incidence is rising at 2% per year and is expected to negatively impact the quality of patients’ lives while resulting in substantial costs to both individuals and the health care system (1). Basal cell carcinoma (BCC) is associated with a better prognosis and makes up approximately 80% of NMSC (1). Squamous cell carcinoma (SCC) makes up the rest of the NMSCs and tends to a more aggressive behavior with greater potential to spread regionally and distally than BCC. The first-line treatment for NMSC is surgical excision. Alternatives include radiotherapy, cryosurgery, curettage, Mohs micrographic surgery, intralesional therapy, photodynamic therapy, immunomodulators, and chemotherapy (2). Given this wide selection of therapeutic options, the treatment approach is guided by multiple factors including the type of NMSC, histologic morphology, lesion location, clinical stage, patient preference, comorbidities, and performance status.
Surgery is the first-line option for most skin cancers as it offers the advantage of providing pathological assessment and lacks the low risk of secondary malignancy associated with radiation (3). The advent of Mohs micrographic surgery has contributed to the increase in the proportion of small skin malignancies of the head and neck treated with surgery because this procedure obviates the need for large margins, providing satisfactory cosmetic outcomes even in sensitive locations. However, there remain cases where Mohs is either unable to provide satisfactory results or the patient is not a surgical candidate. Over the last century, radiotherapy has become established as an alternative therapy for these clinical situations.
Various radiation therapy techniques have been developed to treat cutaneous malignancies. These include electron beams, superficial X-rays, orthovoltage X-rays, megavoltage photons, and high dose rate (HDR) brachytherapy. Traditionally, the type of radiation modality used at a particular institution has been based on local experience and resources. As yet, there has not been a vigorous prospective research experience to guide optimal selection among the radiation modalities. This absence of comparative efficacy research stems from the fact that definitive treatment of small SCC and BCC has not been a priority for mainstream oncology research. Skin cancer research instead has been firmly in the domain of dermatologists, for whom radiation treatment is an unfamiliar territory. The absence of a NMSC section or committee on the National Cancer Institute-funded clinical trial organizations is testament to this observation. Likewise, consensus guidelines for radiotherapy for NMSCs have tended to include a broad diversity of techniques, doses, and fractionation schedules, reflecting the dearth of robust comparisons of these options in the published literature.
Over the last decade, however, there has been a significant change in interest in radiotherapy for skin cancer. In fact, the last 6 years (2009–2015) witnessed the highest growth to date in the use of radiation for this disease. This sea change was not influenced by any new data supporting radiation. Rather, the greatest contributor to this increase in utilization was the advent of electronic brachytherapy (EBT) units. In 2009, the U.S. Food and Drug Administration (FDA) approved the use of these devices for skin treatment. As is described later, these machines operate with minimal shielding and do not require compliance with the Nuclear Regulatory Commission requirements imposed on authorized users of radioactive isotopes. Thus, dermatology practices could own these units and directly bill for these services without the restrictions imposed on users of traditional brachytherapy utilizing live radioactive sources. As a result, use of current procedure terminology (CPT) codes related to these services increased 15-fold from 2008 to 2011 and 47% of the time these codes were billed by dermatologists (4).
In November 2013, the American Academy of Dermatology released a position statement reiterating that surgical treatment remains the primary intervention for treating BSC and SCC and cautioned that EBT should not be administered by dermatologists. Nonetheless, the rapid adoption of EBT has created the peculiar reality that this technique is the most commonly utilized form of radiotherapy for NMSCs even though it is associated with the least amount of supportive evidence. It is hoped that a side effect of this renewed interested in radiation treatment of NMSCs will be the greater emphasis placed on prospective studies that establish efficacy and optimize radiation technique, target, dose, and fraction size.
PATIENT SELECTION
Skin brachytherapy is well suited to select patients for anatomic, medical, and logistical reasons. First, the dosimetric qualities of skin brachytherapy allow for superficial deposition of a homogeneous dose to cancer targets even if they are on curved surfaces. This allows for effective treatment of difficult anatomic locations such as the face, total scalp, fingers, and ears—and allows the patient to achieve good cosmesis without the need for involved reconstructive surgery. Second, patients who are not surgical candidates for medical reasons are excellent candidates for radiation therapy because radiation generally does not require general anesthesia or even conscious sedation for effective delivery. Third, brachytherapy doses fall off rapidly from the radioactive source, which spares deep structures of the body. Therefore, more dose can safely be delivered at each session (hypofractionation). This logistical advantage reduces the time required to deliver radiation and has special appeal for older patients for whom transportation to a radiation center is a challenge.
In light of the earlier discussion, the ideal patients for skin brachytherapy are elderly individuals with small superficial NMSCs. Indeed, patient series examining skin brachytherapy have reported excellent local control in precisely these populations (5,6). Common entry criteria for these studies are the inclusion of BCCs and well to moderately differentiated SCCs. The depth of invasion is typically no more than 3 to 4 mm (due to the depth dose characteristics of skin brachytherapy) and is typically assessed pathologically or via imaging with ultrasound or CT. Exclusion criteria are high-risk pathological features, such as perineural invasion, previous radiation, compromised lymphatic drainage or vascular supply, collagen vascular disease (especially scleroderma), concurrent or recent cytotoxic chemotherapy, genetic disorders predisposing to skin cancers or radiation sensitivity, and very young age. For patients with deeper lesions, interstitial brachytherapy can be utilized (7).
Although NMSCs comprise the lion’s share of publications for skin brachytherapy, other histologies have also been studied. Kaposi sarcoma, a rare malignancy sometimes associated with AIDS, has been found to be amenable to radiation therapy for local control and palliation (8,9). Similarly, cutaneous lymphomas, including mycosis fungoides and primary cutaneous follicle-center B-cell lymphoma, have been successfully treated with skin brachytherapy in both definitive and palliative settings. In fact, skin brachytherapy can offer dosimetric advantages over external beam radiation therapy when targeting extensive cutaneous lymphomas or those in challenging anatomic locations (10).
TREATMENT TECHNIQUES AND APPLICATORS
Among the radiotherapeutic modalities, brachytherapy has become an especially attractive option because of its ability to deliver a contained, superficial dose to the skin, which by nature is easily accessible to a variety of brachytherapy applicators. Interstitial brachytherapy, custom surface molds, prefabricated multichannel flaps, tungsten alloy cup-shaped applicators (i.e., Valencia and Leipzig applicators), and EBT are among the tools in the armamentarium of the radiation oncologist who treats skin cancer. The ability to tightly conform dose using brachytherapy is exemplified in one report describing custom surface mold brachytherapy. This dosimetric study demonstrated a uniform dose distribution at the skin surface and at 5 mm depth with a variability of less than 5% (11). Furthermore, radiation delivery was precise and conformal with the normal tissue outside the high-dose-radiation zone receiving a negligible dose. In point of fact, the dose gradient fell off so sharply at the edges that no measurable radiation dose was detected 5 mm from the applicator (11).
In the remainder of this section, we briefly survey the different brachytherapy techniques currently used in practice. More detailed information regarding their use is given in the subsequent section on skin brachytherapy work flow.
Leipzig and Valencia Applicators
Leipzig and Valencia applicators are the simplest way to deliver skin brachytherapy. They are essentially cup-shaped applicators made of tungsten alloy designed to hold a radioactive source near the skin while shielding structures outside the cup (Case 8.1). They are ideally suited for flat, well-circumscribed lesions. The Leipzig applicator has a simple bell design while the Valencia has a flattening filter to provide a flatter, more homogeneous distribution (Figure 8.1). These applicators provide optimal dose distribution to 3 mm with the surface dose being around 138% (Figure 8.2) (12).
Custom Molds and Flaps
For skin areas that are too large or irregular to be treated with a shielded surface applicator or external beam radiation, an alternative is a prefabricated multi-lumen catheter flap such as the Freiburg Flap (Elekta, Stokholm, Sweden) and the Harrison–Anderson–Mick (HAM) applicator (Mick Radio-Nuclear Instruments, Inc., Eckhert & Ziegler, New York, NY, USA). These custom molds offer an elegant solution to curved or irregular surfaces because they can be laid directly onto the surface being treated in a way that conforms to its curvature (Case 8.2). Regions well suited for custom molds include the ear, nose, or fingers.
Custom surface molds are typically constructed from specialized polymers to fit a tumor surface area (Figure 8.3). Radioactivity is then integrated either by using afterloading catheters or by integrating isotopes into the mold itself. When using an afterloader, the time the radioactive source spends at each dwell position is determined at treatment planning with the use of three-dimensional (3D) planning using CT or MRI simulation images, and care must be taken to ensure that the catheters meet the afterloading unit’s minimum turn radius.
Figure 8.1 Tungsten alloy–shielded applicators (Elekta, Stokholm, Sweden). Leipzig applicators in available sizes of 1 cm, 2 cm, and 3 cm (A) and Valencia applicators in sizes of 2 cm and 3 cm (B).
Figure 8.2 Graph to show the dose profiles of the Valencia and Leipzig applicators.
Figure 8.3 Custom mold. (A) A custom surface mold applicator consisting of a layer of thermoplastic annealed to the skin with 11 blind ended interstitial catheters sandwiched between the lower layer and an outer layer of thermoplastic matching the edge of the clinical target area delineated by the dotted blue line. (B, C) An axial and sagittal CT representation of a computer-optimized HDR isodose distribution demonstrating excellent dose conformity and fidelity to the clinical target as delineated. The yellow isodose line is 100% and shows rapid fall off to 50% at the blue isodose line. Clinically placed external eye shields further prevent scatter to orbital structures.
Custom molds and flaps allow for treatment of various target sizes and shapes, but they do not provide the integrated shielding of surface applicators. Adjacent skin surfaces outside the target volume may inadvertently receive the dose from the isotropy of the nearby dwell positions as exhibited in Case 8.5. At times, it may be necessary to use lead shielding to protect uninvolved structures as will be described in more detail subsequently.
Electronic Brachytherapy
EBT employs a miniaturized HDR X-ray source that emits photons with a maximum energy of 50 keV from the tip of a catheter. The controller supplies a high-voltage filament current and circulates cooling water to the X-ray source, which can be moved through linear dwell positions. Skin applicators are available in different sizes (ranging from 10 to 50 mm; Figure 8.4). Importantly, there is no radioactive isotope source involved in this device, which negates the shielding and regulatory requirements associated with traditional brachytherapy techniques. In 2009, the FDA granted marketing clearance for the first EBT unit (the Axxent Surface Applicator for use with the Axxent eBX, Xoft, CA, USA) for delivering skin surface treatments.
Figure 8.4 Electronic brachytherapy unit.
Variations in Practice
The ideal dose, fractionation, and treatment technique for NMSCs have not been determined in prospective randomized trials. Therefore, there is a large degree of variation in practice standards throughout the world and even within localities. For instance, in a survey of the current practice of external beam radiotherapy for NMSC in the United Kingdom, 24 different dose fractions were used (13). A survey of Canadian radiotherapy centers that use surface mold brachytherapy also found a wide degree of variation in patient selection, dose schedules, and dosimetric constraints (14). These variations in practice highlight the need for national practice guidelines informed by prospective studies.
TREATMENT TOXICITY AND COSMESIS
The side-effect profile of skin surface brachytherapy is similar to that of external beam radiotherapy (EBRT). Acute reaction is limited to erythema, pruritis, dryness, and desquamation. The timing of onset varies and depends on fractionation. When hypofractionation schedules are used, the acute reaction may peak in severity weeks after the treatment course is over (Case 8.3). The acute reaction usually resolves in 4 to 6 weeks after completion of therapy. Late effects include alopecia, telangiectasia, skin atrophy, and scarring (15). The degree of severity tends to be mild (16).
TREATMENT EXPERIENCE AND RESULTS
Currently, the NMSC radiotherapy literature is limited mainly to case reports and retrospective series. Moreover, comparative effectiveness of surgery and radiation in this setting has been quite limited. Of interest, a single randomized trial has been published comparing surgery and definitive radiation. Avril et al enrolled patients between 1982 and 1988 in a trial comparing local excision to definitive radiation for BCC of the face. Radiotherapy techniques were heterogeneous and included 50 kV superficial contact therapy, conventional radiotherapy (85–250 kV), and interstitial brachytherapy. They found radiotherapy to be inferior to surgery with an actuarial failure rate of 0.7% in the surgery group compared to 7.5% in the radiotherapy group (17). Retrospective studies comparing surgery and radiation are abundant and report local control rates at or beyond 95% regardless of the treatment (18). Selection bias has been blamed for marginally worse outcomes associated with definitive radiotherapy in some of these studies because patients not receiving surgery tended to be older, frailer, and afflicted with more aggressive cancers.
Published experiences are summarized in Table 8.1.
Table 8.1 Published experiences of brachytherapy for skin cancer
The data supporting Valencia and Leipzig applicators are also somewhat limited. The largest series reports on 520 patients treated between 1987 and 1998 with the Leipzig applicator (22). Local control was 92%. Total doses ranged between 30 and 40 Gy, delivered in 5 to 10 Gy fractions. Another large series reported on 200 patients with 236 lesions treated with a Leipzig applicator at 36 Gy in 12 fractions (21). With a median follow-up of 66 months, cosmesis was good or excellent and 98% local control was observed in this population. The main late complication was skin hypopigmentation, which was observed in 13 cases (5.5%). Recently, Tormo et al reported on 32 patients with 45 NMSC lesions treated with the Valencia applicator from January 2008 to March 2010 (6). The prescription dose was 42 Gy in six or seven fractions. Local control was reported to be 98% with most patients having a follow-up of more than 3 years. No grade 2 or higher acute or late events were observed.
As stated previously, the data for EBT are not extensive. The majority of published peer-reviewed data for this modality come from small, retrospective studies with limited follow-up, and there is insufficient evidence in the literature to compare EBT outcomes to standard radioisotope-based brachytherapy. Among the largest series is that of Bhatnagar, who described clinical outcomes at 1 year after surface applicator EBT for the treatment of 122 patients with 171 NMSCs between July 2009 and April 2012 (16). The treatment dose was 40 Gy over eight fractions, and at a mean follow-up of 10 months, the author observed a 100% local control rate. He also reported excellent cosmesis in 93% of patients and good cosmesis in 7% of patients.
GENERALIZED WORKFLOW FOR TREATMENTS USING SURFACE APPLICATORS
In this section, the clinical workflows are presented for:
1. Treatment using flap applicators such as the Freiburg Flap (Elekta Brachytherapy), end Catheter Flap Set (Varian Medical Systems, CA, USA), and custom-made applicators
2. Treatment using skin and surface brachytherapy applicators with fixed-geometry Leipzig Applicator Set and Valencia Skin Applicator (Elekta Brachytherapy), and Surface Applicator Set with Leipzig-style Cone (Varian Medical Systems)
Clinical Workflow for Multichannel Flap and Custom-Made Applicators
The detailed process map of skin treatments using flap and custom mold applicators is presented in Figure 8.5.
During the first phase (pretreatment preparation and simulation), the presence of a physician, a medical physicist, and a radiation therapist is required. General preparation in advance of the patient visit requires assembly of the applicators, tools for immobilization and shielding, radiopaque marking wires for the flap applicators, and/or mold materials.
Figure 8.5 Detailed process map of skin treatments using flap, mold, or custom-made applicators.
Target definition is the process in which the physician delineates the clinical target volume (CTV). The radiation therapist places the CT simulation skin markers on the outlined contours to aid in CTV definition on CT imaging (Figure 8.6).
Figure 8.6 Delineation of the CTVs using CT simulation skin markers presented on various sites: arm, abdomen, flank, scalp, and neck.
Figure 8.7 Custom-made applicator for treatment of the face. The flap applicators are attached to the immobilization mask.
Applicator preparation is the process consisting of several tasks such as catheter numbering, placement of the markers inside each catheter, and, if required, abutting the catheters for the treatment of large surfaces. Catheter numbering is crucial in order to avoid a misadministration. The purpose of the immobilization process is to make a reproducible and stable setup for treatment.
Fabrication of a thermoplastic shell over the target surface area serves several purposes. It provides immobilization, ensures a constant source-to-skin distance, and provides a matrix to which a flap or individual catheters can be secured (Figure 8.7). During simulation, the physician outlines the CTV, which is then transposed onto the shell. The physicist prepares the treatment device by attaching the flap applicators to the shell with respect to the marked CTV. During the mask placement and CT scanning (at Day 2 of simulation), a radiopaque wire should outline the CTV, and a CT scan should be obtained. Patient feedback related to discomfort should be taken into account, especially in cases of long fractionated treatment regimes.
The treatment setup should make use of shielding, especially on the face. Eyes, nose, and uninvolved skin can all receive radiation from a nearby source. In order to avoid collateral damage, we suggest liberal use of shielding. Case 8.6 illustrates the use of pliable lead shielding with a Freiburg flap.
The preferred slice thickness for CT simulation should be 1.5 mm to deliver more accurate catheter reconstruction and planning.
A clinical report should be made by a trained radiation therapist. This report should contain setup notes and clinical photography. Documentation should be comprehensive so that those not present at the simulation should be able to visualize and reproduce catheter position.
During the second phase (treatment planning), a medical physicist or a trained medical dosimetrist should be able to generate a valid treatment plan based on the information provided in the clinical report. There are several methodologies for making the treatment plan in surface brachytherapy, such as inverse planning engines, a combined method in which automatic optimization is performed only in the initial planning phase, or manually optimized planning. Each of the approaches has its advantages and disadvantages. For instance, contouring of the CTV and organs at risk (OAR) is necessary for the utilization of inverse planning engines. This requires more time; therefore, it can be inconvenient for large targets. The method we present here has proven in clinical practice to be efficacious and effective.
General planning principles are summarized as follows:
1. In most cases, it is not necessary to contour the CTV.
2. There is no need to contour the skin.
3. The skin markers (wires) are crucial for planning, and they have to be contoured.
4. One hundred percent of the prescription dose should be delivered at a depth of 3 mm under the skin surface (to reach the dermis). It is possible to generate the plan with a nonconstant depth of the prescription isodose line, that is, the central part of the prescription isodose line can measure up to 8 mm in depth, whereas the coverage of the target at the periphery can be 3 mm in depth.
5. When planning a complex surface applicator, the 125% isodose line should stay off the skin or just touch it (to spare stem cells and vasculature). In some cases, the skin dose can be up to 138% of the prescription dose for a treatment depth of 3 mm. This decision should be made by the physicians for a specific case.
6. The dose distribution at a depth of 3 mm under the skin surface should be as homogeneous as possible.
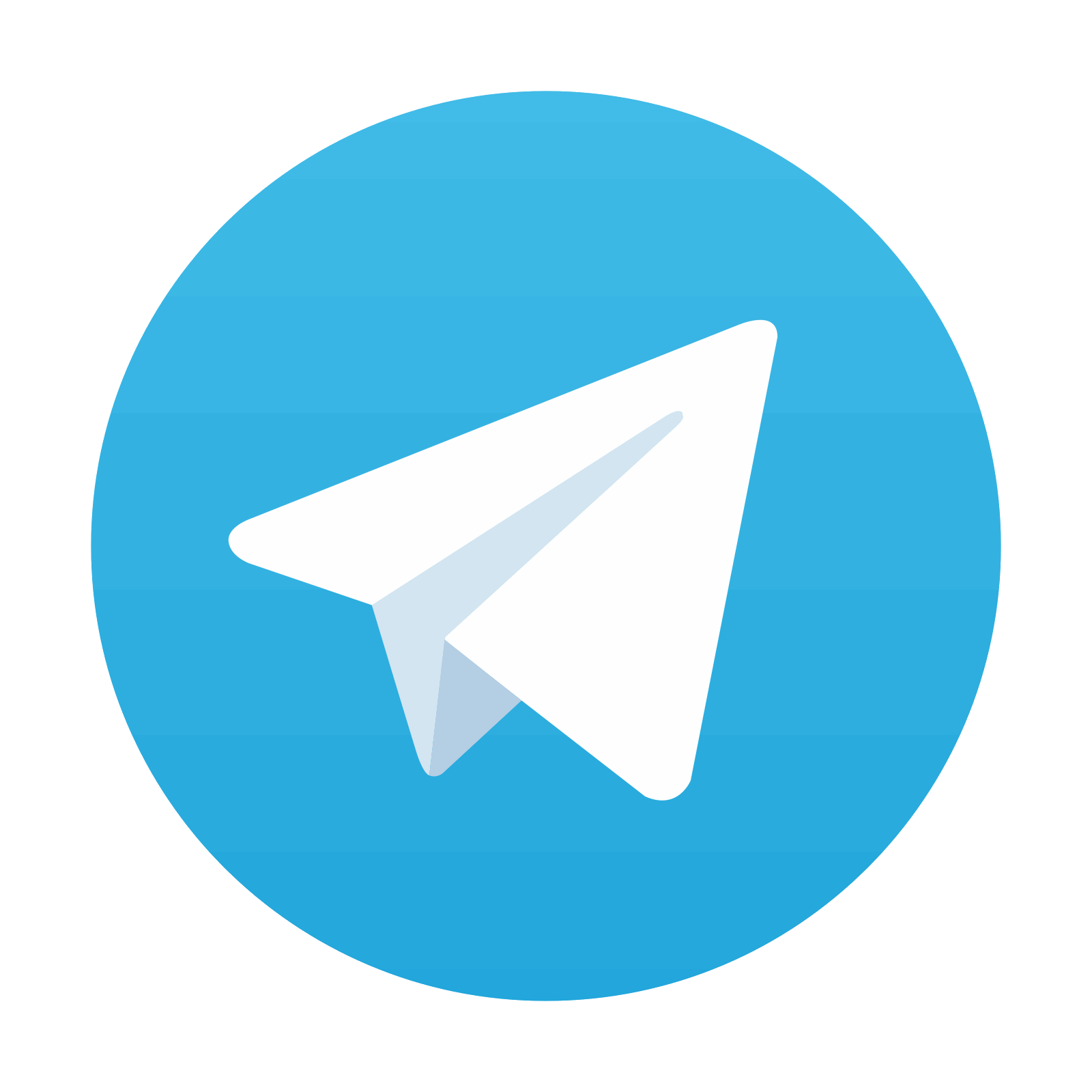
Stay updated, free articles. Join our Telegram channel

Full access? Get Clinical Tree
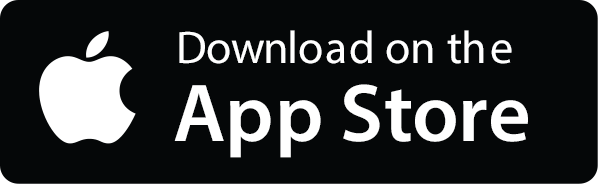
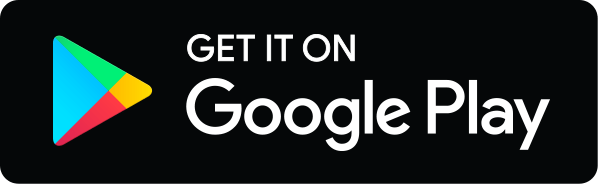