Fig. 1.
Example of an MRI-compatible animal imaging chamber, with features for accurate body centralization and limb positioning. The sealed chamber ensures stable anesthetic delivery. Slots can be included for fiducial markers (see inset), either by insertion of small plastic tubes that contain a signal standard or by insertion of standard materials. The foam insert is disposable, enabling inherent control of biosecurity and prevention of animal cross-contamination. Images courtesy of Numira Biosciences.
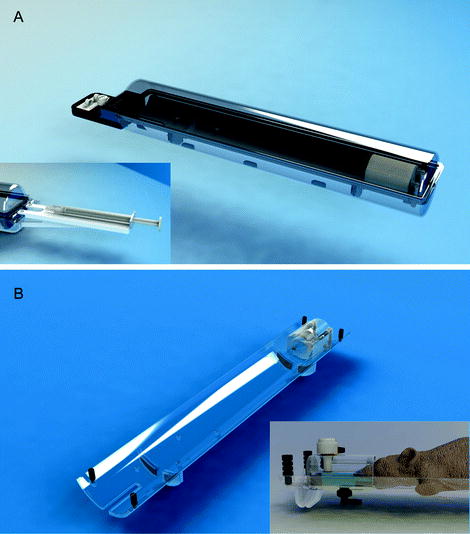
Fig. 2.
(a) An animal management system that incorporates a modular approach that facilitates parallel animal preparation and multiple modality translation. Functionality for an attachable syringe holder is shown (inset). The management system includes a modular “sled” (b) that is inserted into the device. The sled incorporates head restraint features as shown in a close up (inset). Images courtesy of m2m Imaging.
Consistency in animal to animal positioning in order to reduce inter-subject variability and random error in the measurement is as important as accurate and well thought out positioning of the animal in the RF coil. Animal management devices that enable easy and accurate control of the above aspects of positioning also inherently ensure reproducibility. Not only does this increase data quality, it can lead to dramatic decreases in the time spent in preparing the animal physically and preparing the MR scan through software, including the time required for shimming, pulse calibration, and slice positioning. Accurate positioning of both the coil in the magnet and animal with respect to the coil to within ∼1 mm can make slice packet and read offset adjustment redundant as well as ensure that little or no adjustment of tuning, matching, shimming, and pulse powers are necessary.
The critical features of an MRI animal bed are outlined in Table 1. New animal management technologies are becoming available that include integrated packaging of many of the features described in Table 1 (Figs. 1–4). An imaging facility focused on throughput will generally desire to have more than one animal bed, so that while an animal is being imaged, the next animal can be anesthetized and positioned. An attractive method for this is use of a modular “sled” or smaller bed which is inserted into and connected with the larger, more elaborate management system (Fig. 2). This can simplify the process of animal preparation as well as enable animals for future imaging to be prepared in advance. It can also facilitate translation of the bed for use in other imaging systems, even while the animal remains under anesthesia.
Table 1
Desirable features for animal positioning and handling management technology in MRI (see Note 3)
Feature | Purpose | Advantages for |
---|---|---|
Mechanism for locating coil in magnet | Ensure RF coil is precisely centered in magnet bore | Sensitivity |
Mechanism for 3D location of subject in coil | Ensure animal is centered in all three dimensions, facilitate fine adjustment for multiple exams | Sensitivity and homogeneity |
Nose cone and bite bar | Center animal head and ensure reproducibility between subjects | Image preparation time, robustness of anesthesia |
Head restraint or ear bars | Precise horizontal location of head and motion restriction for brain imaging | Image preparation time, motion artifact prevention |
Mechanism for limb and body localization | Ensure reproducible shape of body and relative positions of all limbs | Sensitivity, homogeneity, image preparation time |
Fiducial marker incorporation | Enable robust knowledge of relative position across different imaging sessions and subjects | Co-registration of images over time, images in different animals or between different modalities |
Anesthesia integration | Remove need for external tubing to carry anesthetic | Anesthesia reliability, animal preparation time |
Warming capability | Maintain animal core temperature | Animal well-being, physiology, data quality |
Physiological sensor integration | Inbuilt sensors for respiration, ECG and temperature | Monitoring reliability, animal preparation time, inherent physiological characterization |
Physiological monitoring electronics integration | Ensure robust monitoring and remove need for external cables for monitoring signals | Monitoring reliability, animal preparation time, inherent physiological characterization |
Intravenous drug injection functionality | Allow guides for localization of a drug infusion line | Robustness of intravenous drug injection or infusion |
RF coil integration | Functionality for changing RF coils efficiently while ensuring bed integration | Flexibility for use across multiple applications |
Multiple modality compatibility | Enable use in other imaging modalities, e.g., PET, SPECT, CT, optical | Multi-modal imaging and co-registration |
Inclusion of modular animal “sled” | Enables rapid animal positioning and use of multiple sleds for positioning of other animals while imaging is ongoing | Efficiency and translation of sled use across other imaging modalities |
4 Integration of RF Coils with Animal Management Technology
Integration of the RF coil and the bed is a key challenge, due to the wide variety of coil designs from a number of different manufacturers. While coil integration may not be possible across all coil platforms, the bed should at least address the need for using different coils for different applications, including both volume and surface coils (see Note 4). One approach is the use of mounting hardware that allows the independent placement of both the coil and the animal bed (Fig. 3). An alternative approach is use of an animal bed platform that can accommodate interchangeable RF coils (Fig. 4).
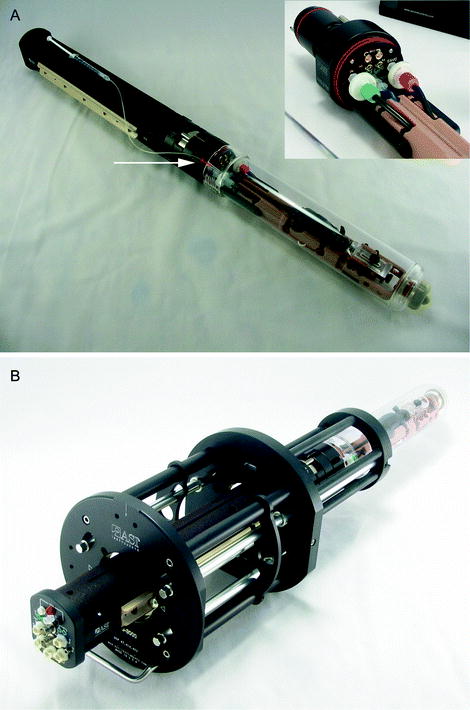
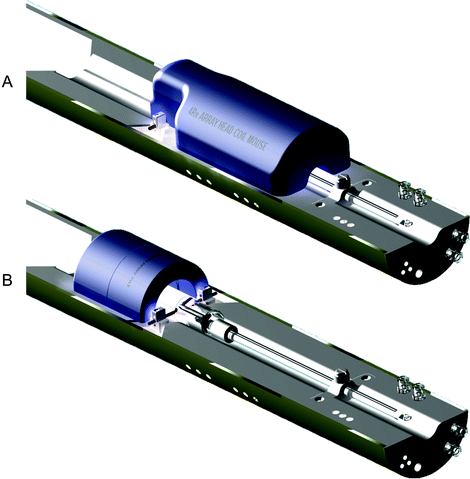
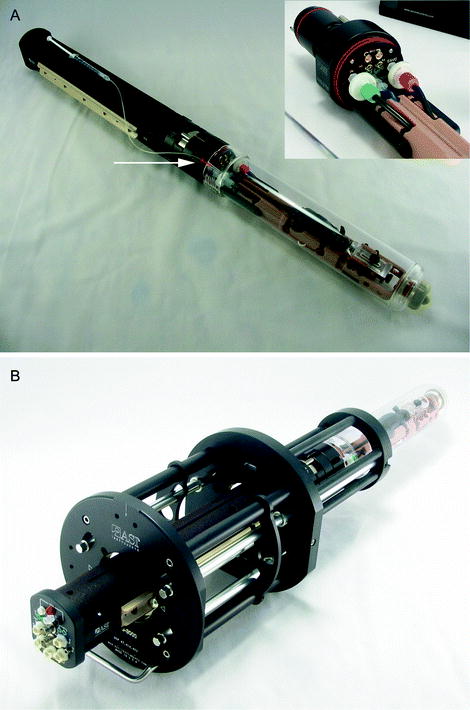
Fig. 3.
Example of an advanced animal management system that incorporates modular technology for (a) the animal bed and (b) a mounting device that holds an RF volume coil and localizes it in the axial direction. The animal bed with sealed chamber is then conveniently inserted using the guiding rungs of the mounting device, which is semi-permanently fixed to the magnet bore. The animal bed shown (a) incorporates multiple channels for physiological monitoring and use of multi-channel surface coils (see inset). A feature for drug infusion is also included (see arrow). A head fixation device and body mold with inclusion of basic limb restraint ensures central and reproducible animal positioning and motion restraint. Images courtesy of ASI Instruments.
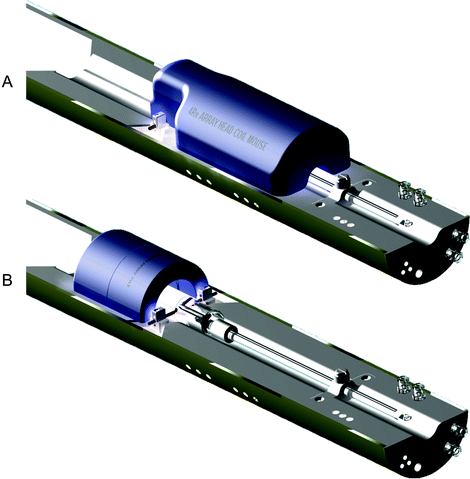
Fig. 4.
Example of an MRI animal bed platform with integrated anesthesia and functionality for animal axial positioning and head immobilization. The bed is designed to allow straightforward removal and exchange of multiple phased array RF coils for specific applications including (a) head imaging and (b) cardiac imaging. Images courtesy of m2m Imaging.
While RF coil integration can provide an optimal method for addressing the unique demands of MRI as it relates to animal position and ease of animal preparation, it can also lead to necessary compromise in sensitivity as the coil may need to be larger than ideal, due to having to accommodate hardware for animal positioning. Another disadvantage of this approach is that it may restrict compatibility with imaging systems across other modalities, unless a modular approach is taken, where the bed itself can be separated from the coil. A core facility that operates in multiple disease areas and in a variety of models may prefer manageable compromises on sensitivity to enable maximum flexibility for use with different coils and across modalities. On the other hand, a smaller facility focused on more specific research topics may prefer customized animal management for each RF coil in use.
4.1 Co-registration and Fiducial Markers
Multi-modality capabilities are rapidly becoming the standard for in vivo imaging facilities. As the preclinical imaging field has matured, the power of multi-modality imaging has been demonstrated, and today PET, SPECT, optical, ultrasound, and CT imaging modalities are commonly used in tandem with MRI. Additionally, MRI itself is commonly used in a longitudinal fashion in the same animals over time. The application of multiple scans or MRI protocols in a given imaging subject is also becoming the standard, rather than the exception. All of these trends highlight the importance of image co-registration. While software based methods for co-registration using non-rigid body approaches such as mutual information (4) are now commonly available (see Note 5), the use of fixed fiducial markers has become prevalent and can aid simple, robust image co-registration between MRI scans and between modalities. This functionality can be conveniently incorporated into the animal bed (Fig. 1) through the use of fixed standard materials or fillable volumes where liquids can be used to provide standard localized signal for later co-registraton.
5 Anesthesia
Today, the vast majority of imaging applications rely on anesthesia (5–8). The breathable halogenated ether isoflurane (6, 7) is the most commonly used anesthetic due to rapid effect, rapid recovery time, excellent tolerance by mammalian species, and low cost. Sevoflurane (7) is another halogenated ether used for this purpose. Both have little or no known toxic side effects as they are not metabolized. They are also relatively insoluble in the blood, which promotes rapid recovery when the gas delivery is turned off.
Isoflurane can be delivered in either oxygen or air, with dosage level accurately controlled in real time by a vaporizer that mixes vapor-phase isoflurane with the breathable gas of choice. This provides advantages over injectable methods of anesthetic, as the dosage level can be easily adjusted based on the specifics of the species, model, and treatment being used. The dose can also be adjusted over time after an initially high induction dose of up to 4–5% concentration, allowing fine control of respiratory rate and health of a research animal over several hours if necessary. Modern imaging labs are therefore normally fitted with centrally supplied compressed air and/or oxygen, multiple isoflurane vaporizers and gas piping that integrates the anesthetic with the imaging system(s). It is then a simple matter to open the appropriate valve to deliver the anesthetic to the induction chamber, animal bed or imaging bore.
The predominant alternative to breathable anesthesia is injectable anesthetic and most commonly the dissociative anesthetic ketamine is used in combination with the sedative/relaxant, xylazine. Use of ketamine/xylazine anesthesia can be preferred in cases where cardiac physiology is critical, as isoflurane is known to affect cardiac function (9). Ketamine/xyalzine also results in more relaxed respiration, compared with isoflurane, which can cause erratic respiration. In MRI, this can lead to undesirable motion artifacts, and therefore isoflurane anesthesia may not be preferred in thoracic imaging applications. The potential physiological side effects of short- or long-duration anesthesia and use in oxygen versus air should be well understood within the context of the end points being quantified (8). Animal dehydration during anesthesia is a primary concern and can be addressed by the use of eye lubrication and post-anesthetic intraperitoneal saline injection for rehydration and recovery.
6 Anesthesia System Design
A basic anesthesia system comprises just a few simple components, including these:
Air or oxygen supply: by a centralized compressor delivered through piping or by individual gas tanks. Centralized compressed air removes the need for frequent tank exchange and the risk of a tank emptying during an imaging run.
Vaporizer: accurately mixes anesthetic with input air or oxygen with output of anesthetic gas at known concentration and flow rate.
Induction chamber: takes gas from the vaporizer for induction of anesthesia
Tubing from vaporizer to animal bed: takes anesthetic gas to the animal bed/RF coil/magnet
However, design and implementation of a more elaborate system, while more costly, can result in large increases in animal anesthesia reliability, efficiency, and flexibility, and also in enhanced occupational health and safety of laboratory workers. Thought should be given to capacity for induction of multiple animals simultaneously, capacity for lab bench animal procedures under anesthesia (e.g., catheterization), and delivery of anesthetic via ceiling drops at the magnet bore opening and other animal work areas. New products are now available that provide management of multiple anesthetic lines via a small consolidated unit with easy adjustment and accurate control of flow rates, and switching between multiple lines that control supply for induction, procedure, and imaging (Fig. 5). The following components are features of an optimally designed MRI anesthesia system:
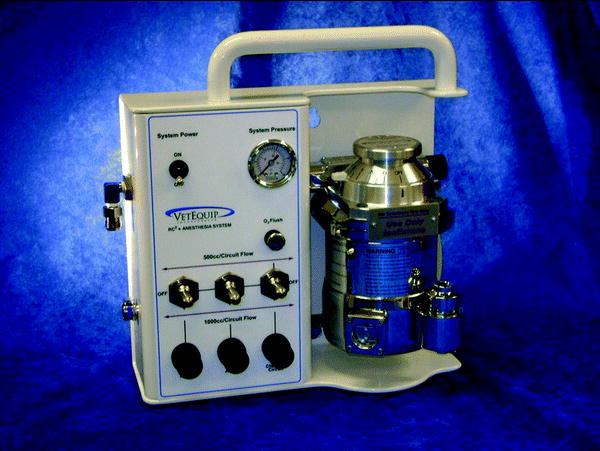
Robust delivery: The breathable anesthetic gas to the animal must be delivered efficiently to the animal’s snout. Commonly, this is achieved using a well-designed nose cone (Figs. 2 and 3) or by using a sealed imaging chamber (Fig. 1).
Integrated animal warming: Warming should be incorporated in all induction chambers, procedure stations, and animal beds by warm water, warm air or heat lamp.
Excess anesthetic gas removal: Non-breathed waste gas must be removed either passively or actively using a suction pump and can be absorbed by charcoal canisters or vented to the outside air. This includes the animal bed nose cone, which should be designed as a closed circuit, such that any non-breathed anesthetic gas is channeled for absorption or to the outside world. Centralized suction can greatly facilitate excess gas removal, including waste gas removal from the magnet bore.
Devices for mounting charcoal canisters and tubing management can greatly aid organization and make optimal use of space, which is commonly limiting.
Gas flow regulation: Use of devices that regulate gas flow increase the robustness of the anesthesia, ensuring adequate volume delivery while also not allowing excess volume delivery that could result in unnecessarily large waste gas volumes and potential environmental exposure. Commonly, the effect of tubing bore size and length in determining resistance and flow rate is neglected leading to potentially inadequate or excessive flow rates. Technology that relies on a pressure-driven approach that is independent of traditional flow meters can ensure accurate flow regardless of tube size, length, and load (Fig. 5).
Filters: Filters for particulates, water, and oil should be incorporated into all incoming gas lines to ensure animal health and also to reduce fire hazard where oxygen is being used.
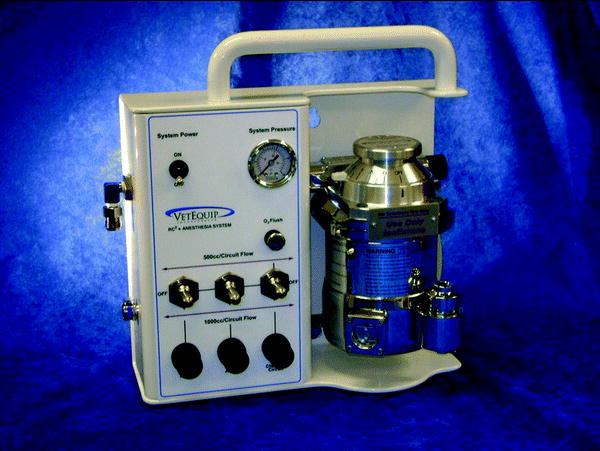
Fig. 5.
A multi-channel isoflurane anesthesia device for control of multiple anesthesia lines using constant pressure technology that ensures accurate simultaneous flow of anesthetic gas to induction chambers, procedural workstations and imaging systems. This device was designed to control simultaneous anesthesia delivery to multiple imaging systems. Image courtesy of VetEquip.
While cheap and commonly available materials can provide functional anesthesia delivery temporarily, it pays to use tested materials that will resist wear and rupture, preventing compromised anesthesia delivery and potential exposure hazards. While isoflurane is considered a non-toxic chemical, studies have demonstrated potential safety concerns and minimizing environmental exposure of technicians during usage should be an area of focus for an MRI lab. For example, induction boxes that utilize active suction to minimize exposure during chamber opening are now available (see Note 6). Monitoring actual exposure through sensors that are worn on external clothing can be a reliable way to demonstrate acceptable exposure and track improved exposure in problem areas (see Note 7).
7 Motion Restriction and Imaging of Conscious Animals
While in vivo MRI is predominantly achieved using anesthetized animals, the use of MRI in conscious animals is increasing in parallel with recent advancements in MRI-compatible motion restriction devices (see also Chapter 17). Imaging in conscious animals is a particularly important consideration in functional MRI applications where brain activation in response to stimulus is key to the experiment. As functional MRI protocols are extended increasingly to small animal models, imaging in awake animals will also increase (10–12). Devices for this purpose are usually based on traditional stereotactic technology (Fig. 6) such as bite bars, nose cones, and ear bars (3, 13).
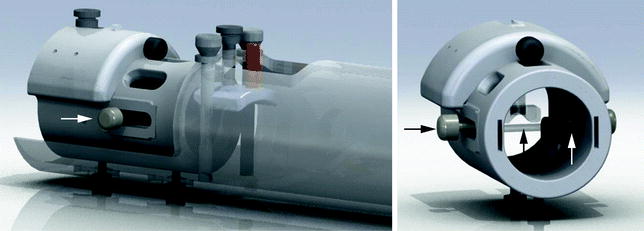
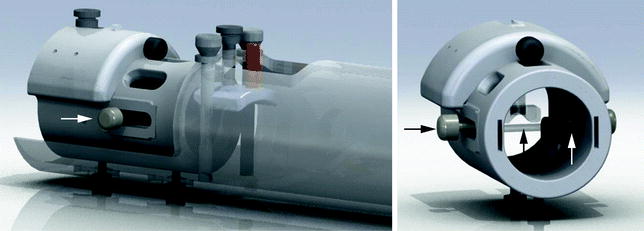
Fig. 6.
Example of an RF volume coil with integrated stereotactic functionality (see arrows) that can be used for awake animal brain imaging. This strategy can also be used with anesthetized animals for precise brain imaging. Images courtesy of EKAM Imaging.
Of course, these methods have more general applications in motion restriction for MRI, even in anesthetized animals. Respiratory motion is a large concern in MRI, where even small motion during the scan can translate to significant phase artifacts that increase data variability or may even render a slice or entire image as being non-quantifiable. Respiratory motion commonly extends throughout the anesthetized animal’s body and can even create severe motion artifacts in brain imaging. In small animals, even the use of tape and immobilization splints can localize full body respiratory motion to the thorax, immobilizing the head or lower abdomen. Fortunately, the incorporation of simple mechanisms for motion restriction such as bite bars and nose cones are now commonly incorporated into MRI animal bed and/or RF coil design negating the need for an enormous inventory of surgical tapes in today’s imaging lab! These features not only help to reduce animal respiratory motion, but also inherently increase the reproducibility of the animal position. For example, stereotactic devices ensure the animal’s head is fixed in space both during a procedure and between procedures, providing inherent registration of images and minimizing animal preparation and setup time.
8 Maintenance of Core Body Temperature
Maintenance of animal body temperature is an important consideration during imaging procedures, especially during the use of anesthesia which generally causes a decrease in core body temperature. There are therefore a variety of MR-compatible techniques that are used for warming of the animal while in the RF coil and magnet. These include the following:
Warm water: This relies on a warm water bath and pump. The warm water is pumped and recirculated to a water-filled pad that makes contact with the animal. The temperature of the water in the bath may need to be adjusted to be above body temperature to account for heat loss from the bath to the animal pad. A simple water-heating pad can be constructed using standard thin plastic tubing or there are a number of commercially available water-filled pads available today (see Note 8) that are thin to minimize space usage and flexible to enable use within imaging bores and coils. It is important to ensure good contact between the pad and the animal and prevent any blockage of the water flow, which will reduce the effective temperature of the water in the pad.Stay updated, free articles. Join our Telegram channel
Full access? Get Clinical Tree