Fig. 7.1
A 81 year old man with painful spine metastases at T6 and T7 of metastatic melanoma as demonstrated on sagittal MRI (a) and sagittal CT treatment plan (b). Axial treatment plan for T6 (c) outlined in red with each target treated to 20 Gy in 1 fraction the 75 % isodose line (ISL) shown in green with 50 % ISL in cyan and 25 % ISL in blue. Target volumes: 3.043 and 3.091 cm3. Maximum spinal cord dose 12.2 Gy. Maximum esophagus dose 10 Gy. Axial PET/CT images for each lesion (d, e)
7.2.2 Case 2
A 50-year-old man with metastatic malignant peripheral nerve sheath tumor who developed two separate areas of vertebral column tumor spread; T5–6 and T12. Multi-modality treatment was employed in this case. As the patient presented with symptomatic spinal cord compression resulting from the T5–6 lesion, he underwent laminectomy and resection at this site with spinal instrumentation. The unresected tumor involving the right T12 pedicle was treated by radiosurgery to 20 Gy in a single fraction to the 75 % isodose line with 90.66 % coverage of target volume. The spinal cord maximum dose was 11.10 Gy. A follow up PET/CT at 4 months demonstrated no hypermetabolic uptake at either site. This case illustrates general principle that surgery remains the standard management option for patients presenting with progressive, symptomatic spinal cord compression (Fig. 7.2).
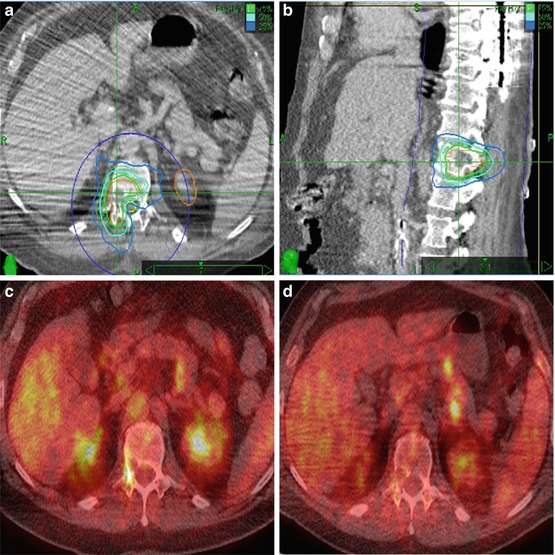
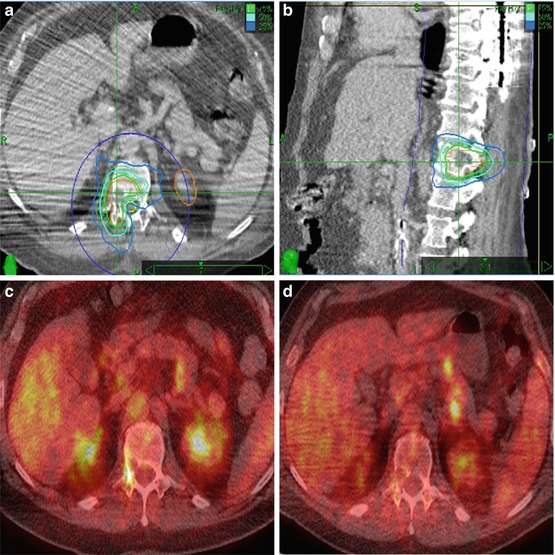
Fig. 7.2
A 50-year old man with right T12 spinal metastasis of malignant peripheral nerve sheath tumor who had previously undergone laminectomy and resection of T5-T6 tumor due to spinal cord compression. Panel a (Axial), b (sagittal). The unresected tumor involving the right T12 pedicle (contoured in orange; note the contralateral kidney also contoured in orange) was treated to 20 Gy in a single fraction to the 75 % isodose line (ISL). Prescription dose illustrated by the green line with the 50 % ISL (cyan) and 25 % ISL (medium blue) 90.66 % coverage of target volume. The spinal cord maximum dose was 11.10 Gy. Panel c-Pretreatment PETCT scan demonstrating FDG-avidity at the right T12 pedicle. Panel d: Follow-up PETCT at 4 months: T12 lesion demonstrates near complete resolution of the hypermetabolic activity
7.3 Literature Review
As evidenced by the dramatic increase in publications on the topic in the past 5 years, review of the literature shows that spinal radiosurgery is increasingly being utilized. Most reports are single institutions studies of <100 patients with a heterogeneous mix of patients with spinal and paraspinal lesions of various primary histologies. Despite these limitations, these studies collectively have demonstrated the effectiveness of spinal radiosurgery with most series reporting nearly 85 % or higher local control and pain improvement [4, 5, 9–14]. Table 7.1 [4, 5, 9–15] summarizes the results of selected series containing >25 patients. In the largest single study of patients with spinal metastases treated by radiosurgery, investigators at the University of Pittsburgh reported long term overall pain improvement in 86 % of the 336 patients with pain as a presenting symptom at up to 53 months follow-up [9]. Although most series have not rigorously used quality of life assessments, a few have attempted to quantify the effectiveness of spinal radiosurgery on quality of life [4, 15, 16]. For example, in a report by Gagnon et al. who prospectively evaluated pain control and quality of life in 200 patients with benign or malignant tumors treated by Cyberknife spinal radiosurgery, there was statistically significant improved pain levels which was durable over 48 months of follow-up [15]. Using the SF-12 validated instrument to evaluate QoL, they also found statistically significant improvements particularly in the mental component of the measurements. Sheehan et al., evaluated the outcomes of 40 previously un-irradiated patients treated by spinal radiosurgery using a helical tomotherapy technique [16]. There was significant improvement of pain relief as assessed by a 10-point visual analog scale (VAS) with a mean score of 3.2 post-treatment compared to a mean pre-treatment level of 6.2. Additionally, function as assessed by Oswestry Disability Index was also improved with the mean pre-treatment score of 43 decreasing to 25 following radiosurgical intervention.
Table 7.1
Selected series of spinal SRS/SBRT
Author and year | Number of patients/lesions | Age (years) | Method | Prior treatment % | Total dose (Gy)/fractions/isodose | Spinal cord dose limit | Follow up months | PI, % | LC, % | Major non-neurologic toxicity | |
---|---|---|---|---|---|---|---|---|---|---|---|
Surgery | Radiation | ||||||||||
Degen et al. [4] | 51/72 | Mean 53 | CyberKnife | 0 | 53 | 10–37.5/1–5/100 % | 2.2–27.1 Gy | Mean 12 | 97 | 100 | 0 |
Gibbs et al. [5] | 74/102 | Mean 59 | CyberKnife | 3 | 49 | 16–25/1–5/80 % | 10 Gy in single fraction | 84 | |||
Gerszten et al. [9] | 393/500 | Mean 56 | CyberKnife | 2 | 69 | 12.5–25/1/80 % | <8 Gy to mean 0.6 cm3 | Median 21 | 86 | 88 | 0 |
Jin et al. [10] | 196/270 | n.r. | Novalis | n.r. | n.r. | 10–18/1/90 % | <10 Gy to 10 % volume | n.r. | 85 | n.r. | 0 |
Yamada et al. [11] | 93/103 | Median 62 | Linac/IMRT | 0 | 0 | 18–24/1/100 % | 12–14 Gy point dose | 2–45 | n.r. | 90 | 1 |
Ryu et al. [12] | 177/230 | Median 61 | Novalis | 0 | 0 | 8–18/1/90 % | <10 Gy to 10 % volume | Median 6.4 | n.r | n.r | n.r. |
Chang et al. [13] | 63/74 | Median 59 | Exact varian | 38 | 55.6 | 30/5 or 27/3/n.r. | <10 Gy | Median 21.3 | Decreased narcotic usage | 84 | 5 |
Nelson et al. [14] | 32/33 | Median 60 | Linac/IMRT | n.r. | 69 | 5.1–16/1–4/n.r. | Varied | Median 7 | 97 | 87 | 0 |
Gagnon et al. [15] | 200/274 | Median 56 | CyberKnife | 7 | 46 | 21–26/3/75 | n.r. | Median 12 | n.r. | 44-significant improvement in pain score average | 3 |
In general, epidural spinal cord compression is considered a relative contraindication to spinal radiosurgery. However, investigators at Henry Ford Health System reported a study of 62 patients with 85 metastatic spinal lesions causing epidural spinal cord compression treated with spinal radiosurgery [17]. With 80 % overall response in the epidural tumor (27 % complete response), significant improvement of the thecal sac patency (from 55 to 76 %), and only 16 % exhibiting neurological progression following treatment, the authors conclude that radiosurgery may be a viable option.
Based on the effectiveness of radiosurgery for benign intracranial tumors such as nerve sheath tumors and meningiomas, spinal radiosurgery has been evaluated in similar tumors occurring in the spine [18–21]. The two largest series of radiosurgery for benign intradural spinal tumors were reported by Sachdev et al. of Stanford and Gerszten et al. [20] of University of Pittsburgh. Using a single fraction regimen, Gertszten et al. [20] treated 73 lesions (13 meningiomas, 35 schwannomas, 25 neurofibromas) to a mean dose of 17.3 Gy to the 80 % isodose line. At median follow-up of 37 months, they reported 100 % radiographic control rate and 73 % of patients had pain improvement. The investigators at Stanford used both single fraction and multi-fraction schedules to treat 103 tumors (32 meningiomas, 47 schwannomas, 24 neurofibromas) with mean dose of 19.4 Gy over an average of 2 fractions. In this series, at mean follow-up of 33 months there was radiographic control in all but 1 tumor, with 40 % of tumors demonstrating decrease in size while the remaining 59 % were stable. Reporting the results of patients whose pain was improved or stable, the best clinical results were observed in patients with meningiomas (100 %) or schwannomas (89 %) while poorer symptomatic outcomes (67 %) were seen with neurofibromas. Radiation myelopathy as a result of treatment developed in a single patient in the Stanford series and 3 patients in the Pittsburgh series.
7.4 Treatment Techniques
Stereotactic body RT has emerged as a new treatment option and is increasingly being applied to treat spinal disease with high biologic equivalent dose (BED) and a steep dose gradient. Stereotactic radiosurgery of spinal lesions requires exquisite body immobilization, sophisticated contouring, complex treatment planning, and near-real-time image-guidance to ensure accurate dose delivery due to the proximity of the spinal cord. Since even modest positioning errors can result in significantly higher dose to the spinal cord [22], stereotactic radiosurgery of spinal lesions is preferably delivered using systems that allow for intra-fractional target imaging and repositioning during treatment. The CyberKnife (CK) (Accuray, Inc., Sunnyvale), Novalis (Brainlab, Ammerthalstrabe, Germany), and other LINAC-based systems using cone-beam CT are the current SRS systems equipped with in-room real time imaging and capable of adjustments during the delivery of treatment. The CK uses intrafractional stereoscopic X-ray imaging, every 30–60 s, and automatically adjusts positional errors with an accuracy of <0.5 mm whereas Novalis has intrafractional positioning adjustments based on the infrared markers placed on the patient’s surface with an accuracy of 1.36 + 0.11 mm [23]. Although tomotherapy (Accuray, Inc., Madison, WI, USA) is a promising technique with a reported phantom accuracy of 0.6–1.2 mm [24], patient studies of accuracy, however, describe greater errors of approximately 4 mm since the megavoltage CT scan is used only for initial set-up and not for re-positioning. Table 7.2 [8, 22–28] summarizes the accuracy data for the most common radiosurgical systems currently in use.
Table 7.2
The accuracy data for the most common radiosurgical systems currently in use
System | Immobilization | Image-guidance | Error analysis |
---|---|---|---|
Cyberknife [23] (Accuray, Inc) | Head mask, cradle, vacuum bag | Xsight skeletal tracking or fiducial tracking | Phantom- 0.61 ± 0.27 mm |
Patient- 0.49 ± 0.22 mm | |||
Novalis [25] (BrainLAb, Inc.) | Head mask, cradle, vacuum bag | Orthogonal images to set-up | Measure iso dose 2–4 % |
Optical tracking | Patient- 1.36 ± 0.11 mm | ||
TomoTherapy [24] (Accuray, Inc.) | Head mask, vacuum bag | CT | Phantom- ± 0.6–1.2 mm |
Patient- ± 4–4.3 mm | |||
Synergy S [26] (Elekta, Inc.) | BodyFix (Elekta) | Conebeam CT | Patient (w/o image guidance)- 5.2 ± 2.2 mm |
HexaPOD robotic couch | Patient (with image guidance)- 0.9–1.8 mm (translational) 0.8–1.6° (rotational) | ||
Stereotactic body frame or body cast | CT | Patient- varies from 1 to 3.6 mm |
7.5 Patient Selection Criteria
Although there is considerable variability among clinicians regarding patient selection for spinal SRS, the current clinical practice is mainly based on retrospective data and phase I-II trials [5, 7, 9, 11, 13, 14, 22, 29–32]. ASTRO has published very conservative suggestions strongly encouraging that these patients be treated within the confines of clinical trials or at centers who are highly experienced at spinal radiosurgery [33]. The following recommendations (Table 7.3) are generated based upon the range of indications as described in the previous studies [4, 5, 7–9, 11, 13, 14, 19, 20, 32–37] and our clinical experiences at Stanford.
Table 7.3
Recommendations for indications and contraindications for spinal SRS
Indications | Contraindications |
---|---|
Progressive but minimal neurologic deficit | Severe neurologic deficit with significant cord compression |
Post resection local irradiation | Neurologic deficit caused by bony compression |
Disease progression despite surgery and/or irradiation | Spinal instability |
Medically inoperable | Lesion not responsive to radiation |
Inoperable | Maximal tolerable radiation doses delivered to adjacent spinal cord |
Recommended indications for spinal SRS of metastatic tumors include; (1) proven spinal disease on MRI or biopsy, (2) single or multiple spinal metastases (≤2 consecutive vertebral levels or up to 3 noncontiguous spinal sites) without symptomatic spinal cord compression or pathologic fracture requiring surgical stabilization (3) previously irradiated (≥6 months interval between courses), (4) residual tumor after surgery, (5) recurrent tumor after prior surgical resection, or radiosurgical boost for radioresistant (e.g., renal cell, melanoma, sarcoma) tumors. In addition, candidates for spinal SRS should have a good performance status (KPS > 60) and be able to lie supine in comfort on the treatment table for the duration of treatment. Benign intradural extramedullary tumors may benefit from spinal SRS where complete resection is not feasible or for recurrent tumors after surgery [18, 20]. While spinal radiosurgery for intramedullary conditions such as spinal arteriovenous malformations (AVMs) and intramedullary hemangioblastomas have been reported by our institution, radiosurgery should be performed in circumstances when surgery is not an option and only by the most skilled interdisciplinary teams [38].
Contraindications for spinal SRS include; symptomatic spinal cord compression, previous SBRT to the same level, unstable spine, inability to assume a position suitable for accurate treatment, and life expectancy <3 months. Based on limited reports of potential spinal cord toxicity, care may be needed when targeted angiogenic therapy is planned within 2 months of spinal radiosurgery. Although ASTRO guidelines suggests an interval of at least 90 days between EBRT and when reirradiation by radiosurgery is being considered, our clinical experience showed that those most benefit from SRS was seen with patients with at least 12 months interval [39].
7.6 Diagnostic Images
Spinal magnetic resonance imaging (MRI) is the imaging modality of choice. However, additional information may be gained from, PET/CT scan, or CT myelography. MRI of the entire spine is recommended in order to exclude multi-level disease. MRI of the involved spine within 4 weeks prior to SRS is crucial both for defining the extent of disease and delineation purposes.
MRI may be acquired using either 1.5-Tesla (T) or 3-T. While 3 T may improve anatomic visualization in the spine and more precisely differentiate tumor infiltration versus normal bone marrow based on its increased signal-to-noise ratio, shorter scan times, and improved resolution, compared to 1.5, 3 T MRI may also be more challenging. For example, 3 T may be associated with increased susceptibility to metal, degradation of bone interfaces (related to chemical shift), reduced contrast on some T1 images (related to longer relaxation times), and higher likelihood of tissue heating (related to higher specific absorption rate). The most useful MRI sequences include T1- and T2-weighted sagittal and axial images as well as short tau inversion recovery (STIR) images [40]. Fat suppression imaging can help to distinguish tumor from normal bone marrow on T2 STIR images. Typically, pathological lesions are hypointense on T1-weighted images with respect to bone marrow, hyperintense on T2-weighted images, and may have variable levels of enhancement with gadolinium. Gadolinium-enhanced, fat-suppressed T1 imaging is particularly useful for imaging of paravertebral or epidural disease. CT myelography is an invasive procedure requiring injection of contrast into the subarachnoidal space and may be used as an alternative to MRI for the purposes of visualizing the spinal cord. While CT myelography, is used less frequently, it can be particularly helpful in circumstances where MRI is not feasible (e.g. claustrophobia or metal implants). Some groups have also investigated the use of 3D Fast Imaging Employing Steady-state Acquisition (FIESTA) MRI for the purpose of spinal cord delineation [41].
7.7 Simulation Studies
Immobilization is a key factor in the accurate delivery of spinal SRS. Patients should be positioned in a stable, comfortable, and reproducible position using vacuum bag, alpha cradle or stereotactic frame. Considering the long treatment duration, supine positioning is preferred over prone. Rigid plastic head and neck mask are preferred for immobilization, for lesions involving the cervical or upper thoracic spine areas. CT scan is the primary imaging for simulation and is used for target delineation and treatment planning. Preferably, high resolution thin slice CT with slice thickness of <2 mm should be used with images extending 5–10 cm above and below the region of interest. For non-coplanar beam arrangements, it is suggested that imaging extend ±15 cm. Co-registration of the CT images with the MRI (gadolinium contrast T1-weighted and T2-weighted images) and/or CT myelogram is highly recommended to delineate the spinal cord properly [7, 8]. Computed tomography (CT) myelography can be used for contouring spinal cord when MRI is not available [7, 41–43]. The CT-myelogram may also play an important role especially for patients with metal implants or claustrophobic. If both MRI and myelogram are not available, the thecal sac or spinal canal should be delineated rather than spinal cord itself.
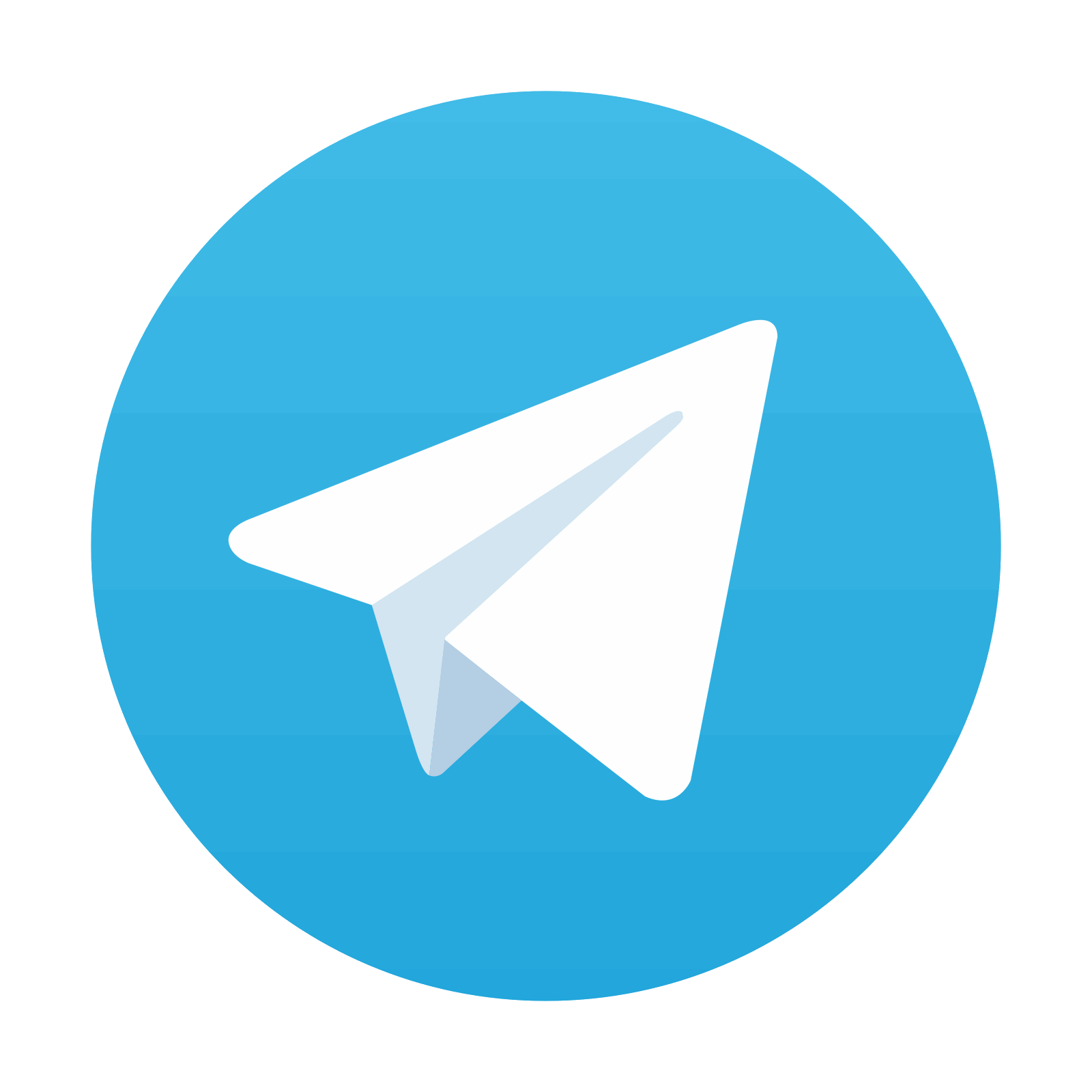
Stay updated, free articles. Join our Telegram channel

Full access? Get Clinical Tree
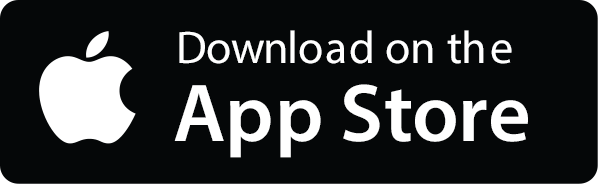
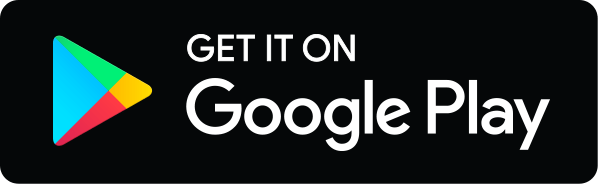