39CHAPTER 3
SRS and SBRT Process
Stereotactic radiosurgery (SRS) is a nonsurgical specialized type of external beam radiation treatment in which large doses of highly accurate, precise, and conformal ionizing radiation are delivered to a well-defined target volume in a single procedure using image guidance. The term “stereotactic” refers to a procedure in which a targeted mass is localized with respect to a fixed three-dimensional reference system such as a rigid head frame. The “radiosurgery” is accomplished by directing beams of radiation along any trajectory in this three-dimensional (3D) space toward the localized targeted mass of known 3D coordinates. Lars Leksell, a Swedish neurosurgeon, developed the term SRS in 1951 as a novel concept of a noninvasive method to treat lesions that were not accessible by open surgery techniques. Patients with trigeminal neuralgia (a nerve disorder that causes pain in the face) were the first to be treated using an orthovoltage x-ray machine mounted to a stereotactic frame. In 1968, Leksell developed the first commercially available dedicated radiosurgical device called the Gamma Knife. The Gamma Knife technology made it possible to precisely deliver a single, large dose of highly conformal radiation to any number of intracranial targets using 201 fixed cobalt-60 sources aimed at a focal point while delivering very low doses to the surrounding normal tissue. This provided an alternative treatment for certain neurosurgical procedures that were then associated with significant morbidity (1, 2). In the era before the emergence of computed tomography (CT), treatments were initially limited to patients with acoustic neuromas and arteriovenous malformations (AVMs), which are tangles of expanded blood vessels that disrupt normal blood flow in the brain and that bleed sometimes (2, 3).
For decades, SRS treatment with Gamma Knife as well as with linacs, TomoTherapy, and specialized devices such as CyberKnife has been successfully used in the treatment of brain metastases and many intracranial neoplasms and functional disorders. Radiosurgery can halt tumor cell division, destroy neoplastic blood vessels, induce apoptosis or necrosis, and, when used intracranially, modify the blood–brain barrier around the tumor (4–10). Since the introduction of SRS, thousands of publications have appeared in the literature reporting the benefits of the use of this noninvasive procedure as an efficient and effective means of achieving a high rate of local control and, in some settings, improved survival (11). SRS is now routinely used for the management of single and multiple metastatic, primary, malignant, intracranial, and central nervous system (CNS) tumors as well as benign tumors such as pituitary adenomas, acoustic neuromas, and meningiomas. SRS is also used for the management of AVMs and other neurological conditions such as trigeminal neuralgia and essential tremor.
The success of SRS in the management of intracranial and CNS tumors has led to the development of fractionated SRS, also called stereotactic body radiation therapy (SBRT), with unique technological and clinical considerations. SBRT uses the advantages of stereotactic guidance to deliver hypofractionated, highly conformal, high doses of radiation to extracranial tumors using image guidance in two 40to five sessions. Biologically equivalent doses greater than 100 Gy can be delivered to various extracranial tumors using this technique.
As in intracranial SRS, SBRT also requires a high level of precision and accuracy at every step in the treatment process. Therefore, successful clinical implementation of SBRT requires careful consideration of details of every step in the treatment process and integration of several technologies that provide solutions to challenges posed by the unique characteristics of various extracranial tumors. Important considerations for SBRT treatments include, but are not limited to, the following:
1. Three-dimensional imaging and localization techniques that determine the exact 3D coordinates of the target within the body
2. Integration of modern imaging systems (CT, MRI, PET-CT, etc.) to visualize and precisely delineate the target volume and critical structures
3. For moving targets such as lung and liver tumors, technologies such as four-dimensional (4D) CT for quantification of motion of tumor(s) as well as that of the critical structures that lay close to the tumor
4. Technologies and techniques to manage tumor motion during SBRT treatment to more effectively spare irradiation of healthy tissue; examples of such techniques are gated treatment delivery or tumor tracking
5. An immobilization system that ensures accurate and reproducible patient positioning throughout the duration of treatment
6. Treatment planning systems with the capability of integrating information from various imaging modalities for delineating target and critical structure volumes and calculating accurate highly conformal dose distributions with a sharp dose gradient using different techniques such as intensity-modulated radiation therapy (IMRT) or volumetric-modulated arc therapy (VMAT)
7. Availability of dose calculation algorithms that can account for the effects of tissue heterogeneities
8. Linear accelerators and/or other machines that are designed for the delivery of SBRT treatments
9. Sophisticated image guidance technologies and techniques, such as cone beam CT imaging, stereoscopic x-ray imaging, fluoroscopic verification of tumor motion, gating, and tracking, to confirm the location of a tumor immediately before and during the delivery of radiation
One of the key challenges of SBRT is motion assessment and motion management for tumors and critical structures close to the tumor that move during treatment. Special considerations must be made to account for the effect of internal organ motion (primarily breathing-associated motion but also bowel peristalsis motion) on target positioning and reproducibility. As a first step, it is necessary to quantify the specific motion of a target or critical structure close to the target. Options for motion assessment include real-time fluoroscopy or 4D CT scanning. The gross target volume (GTV) to planning target volume (PTV) expansion should be no greater than 0.5 cm in the axial plane and 0.7 to 1.0 cm in the craniocaudal plane. If tumor motion combined with setup error causes any PTV to be greater than the GTV beyond these limits, then a motion management strategy (or plan to reduce setup error) must be used with validation of success. The patient should be instructed to breathe normally at the time of initial tumor motion assessment. Deep inspiration or expiration breath-hold is not recommended for the initial tumor motion assessment, as such assessment generally overestimates free breathing tumor motion.
Motion assessment involves a query to appreciate the nature of both tumor target and normal tissue displacement that may occur during a typical SBRT treatment session. Dynamic imaging such as fluoroscopy, ultrasound, or 4D CT is typically required for such an assessment. It is not enough to understand how surrogates for targets move (e.g., the diaphragm for a lung tumor). Instead, the actual motion of the target must be reasonably understood. In turn, this assessment may either allow appropriate expansions of targets to encompass this movement (if the expansion would only be minimal) or trigger the use of motion control. Motion management is a logical reaction to excessive motion appreciated from the motion assessment where the natural physiological motion is either modified (e.g., dampened) or countered with an active process (e.g., gating or tracking).
Motion management is highly recommended for SBRT. In instances in which motion management is not possible, larger expansion volumes will be used to adequately cover the motion-related uncertainties. Typical motion management strategies include 414D CT, active breath-hold, gated treatment, and abdominal compression.
Image-guided radiation therapy (IGRT) uses imaging to facilitate accuracy and precision throughout its entire process from target and normal tissue delineation to radiation delivery to adaptation of therapy to anatomical and biological changes over time in individual patients. Here, the terminology IGRT is used to focus on image guidance at the time of radiation delivery to ensure its adherence to the planned treatment. Sophisticated image guidance reduces uncertainties and allows smaller treatment margins for SBRT treatments. Confidently identifying the stereotactic fiducials (e.g., implanted markers, close body tissue surrogates, or even the tumor itself) before and during treatment mostly eliminates interfraction error and reduces intrafraction error, allowing the smaller margins critical to the overall SBRT approach.
SRS/SBRT processes are unique to every facility practicing radiosurgical procedures. Even within a given facility, they are different for different treatment modalities. Figure 3.1 shows an example of a process map or a process tree for a patient undergoing linac-based SRS/SBRT treatment. The trunk of the tree, as shown by the central arrow, depicts the main process of SRS/SBRT treatment, whereas the branches show the subprocesses that feed into the main process. Each of the subprocesses can then be subdivided into many smaller steps that constitute the subprocesses. For the execution of a successful SRS/SBRT treatment, each step in each of these subprocesses will need to be executed successfully. This particular process tree consists of 18 subprocesses. To gain a better understanding of the overall SRS/SBRT process, in the following text we provide a list of various steps that constitute each of the subprocesses. This list is obviously not exhaustive and is given here only as a guide to the reader on how to build a process map for a given process. It should also be mentioned that details of different subprocesses, that is, immobilization, imaging, technology for SRS/SBRT, treatment planning, and so forth, shown in this process map can be found elsewhere in other chapters in this book. The reader is cautioned that this particular process map is applicable to only one of the author’s institutions and the detail is not necessarily applicable to other cancer centers that provide SRS/SBRT services.
Subprocesses for the linac-based SRS/SBRT process map in Figure 3.1
1. Start of SRS/SBRT process
• Patient referred to radiation oncology
2. Patient information entered into database
• Demographic/insurance information obtained and entered
• Insurance information verified and authorization obtained
• Previous medical and treatment records obtained and entered into database, if/as appropriate
• Previous and outside imaging studies obtained and entered into database if/as appropriate
FIGURE 3.1 A process map for a linac-based SRS/SBRT process.
3D, three-dimensional; 4D, four-dimensional; DICOM, Digital Imaging Communication in Medicine; SBRT, stereotactic body radiation therapy; SRS, stereotactic radiosurgery; Tx, treatment.
• Patient scheduled for consult (±additional tests/imaging needed for the consult and/or patient selection steps)
3. Consult (may include one or more discussions between the patient and the physician)
• Nursing assessment performed and documented
• History and physical examination completed and documented
• Prior medical records and treatment records (if any), including imaging studies, reviewed by physician
• Patient education performed (including financial counseling when needed)
• Diagnosis/staging completed
• Imaging/lab tests ordered and performed as needed to determine patient eligibility, if not done during previous step
• Results of imaging/lab tests evaluated by physician, entered into database
• Alternative treatment methods considered and discussed with patient
• Consent for treatment obtained
4. Patient selection
• Case reviewed with multidisciplinary team
• Lesion type/size/location/stage confirmed to be appropriate for SRS/SBRT
• Role for concurrent systemic therapy determined
• Patient ability to tolerate treatment/immobilization assessed
• Eligibility for open protocol(s) determined
5. Additional supplemental imaging/procedures required for treatment/treatment planning
• Supplemental imaging ordered and performed (e.g., MRI for fusion)
• Need for internal fiducial determined
• Internal fiducial placement ordered and performed (if applicable)
• Lab work ordered and obtained (e.g., BUN/Cr if intravenous [IV] contrast to be given for imaging)
6. Simulation/imaging for treatment planning ordered
• Simulation orders/imaging request(s) completed, including special instructions
• Patient position specified
• Immobilization specified
• Imaging protocol(s) specified, including use of contrast, slice thickness, and interslice gap
▪ More than one image set/technique/imaging protocol may be needed (e.g., free breathing and abdominal compression CTs; axial, sagittal, and coronal MRIs)
▪ Motion management method specified, if used/needed
▪ Patient scheduled for simulation/imaging
▪ Patient given instructions for simulation/imaging preparation
7. Immobilization and positioning
• Immobilization devices created/configured as needed
▪ Can include special devices such as specialized SBRT body frame, Vac-Lock, thermoplastic mask, and head frame
• Description and settings for immobilization devices entered into database
• Devices prepared/attached as necessary for motion management
▪ Can include abdominal compression with either paddle or inflatable belt, Real-Time Position Management (RPM), Active Breathing Coordinator (ABC), audiovisual feedback, and surface-image guidance
• Description and settings for motion management entered into database
• Setup pictures obtained and entered into database
8. Simulation/imaging for treatment planning
• External fiducials attached if/as needed
• Images obtained using imaging protocols as ordered and with positioning/immobilization/motion management as ordered
• 4D CT image acquisition:
▪ 4D CT images acquired covering regions of interest; images retrospectively sorted into breathing phase bins
▪ If institutional protocol is to use maximum intensity projection (MIP)-based approach, then MIP or other composite image sets created if/as needed
▪ Motion analysis performed for target and critical structures, as appropriate
▪ For gated treatment procedure, gating window determined
▪ Quality assurance (QA) performed on the information derived from the 4D CT image acquisition
• Patient marked/tattooed if needed and associated point on the image set defined
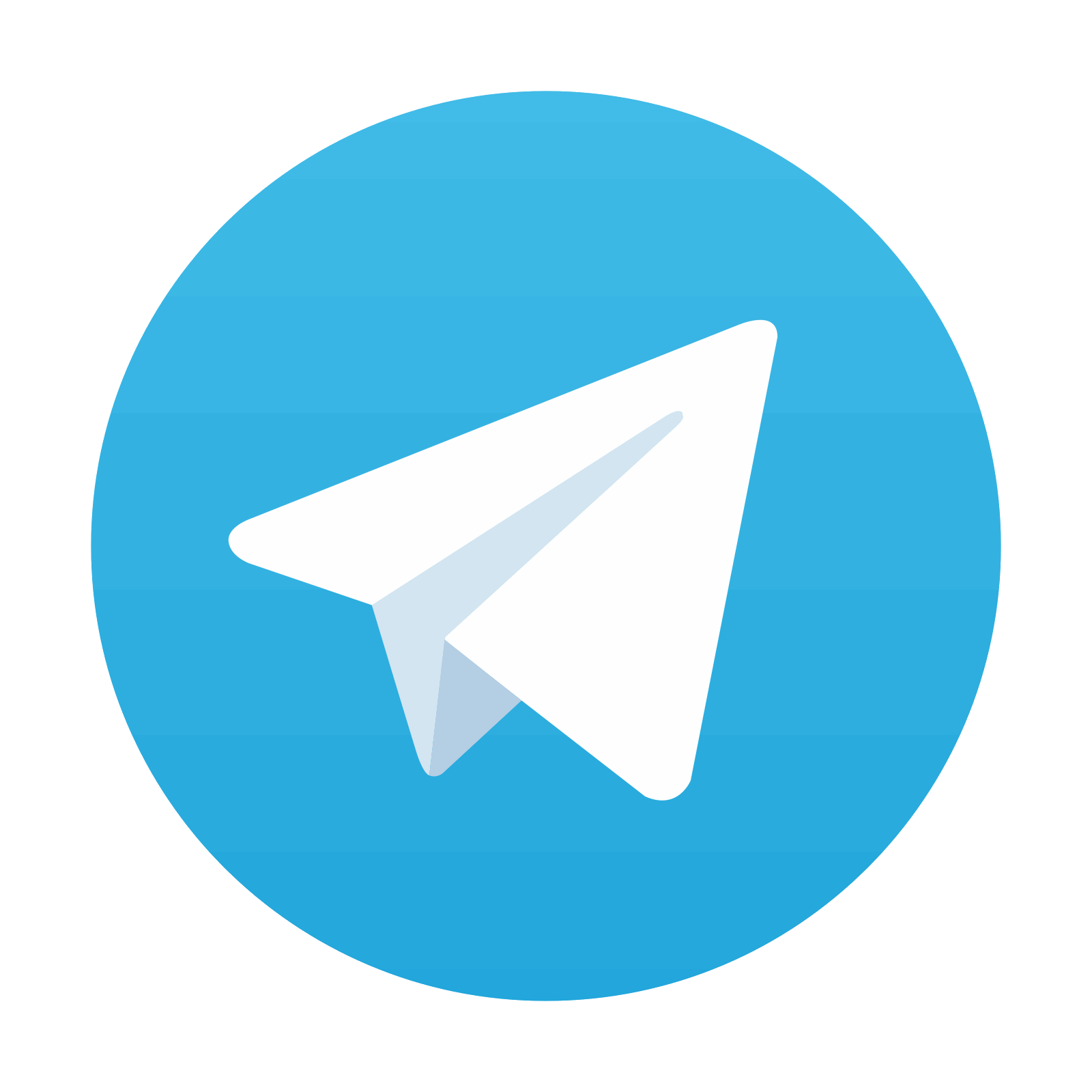
Stay updated, free articles. Join our Telegram channel

Full access? Get Clinical Tree
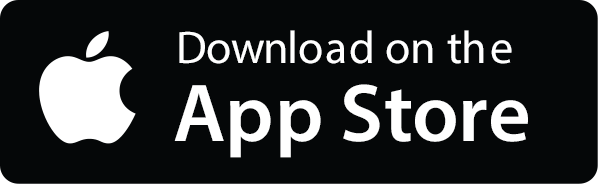
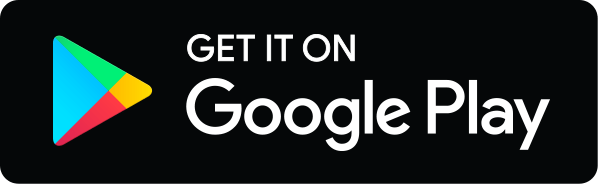
