Fig. 3.1
LB-SBRT immobilization system with abdominal compression by CDR Systems
3.2.2 Image-Guided Localization and Tracking
Immobilization devices can reduce body and organ movement to a certain degree, whilst image-guided localization and tracking, with or without immobilization systems, may reduce the targeting spatial uncertainty to a minimum.
We first discuss the imaging techniques used for locating the tumor in 3D. A variety of technologies are used—some of which involve imaging before the treatment, while others allow monitoring of target during treatment, either continuously or intermittently. These techniques include:
1.
2D MV electronic portal imaging (EPID) [2]. Images are created with the megavoltage beam that is used to treat the patient. Although the imaging quality may be limited, it offers real-time, direct monitoring of the areas being irradiated.
2.
Dual or multiple KV image systems, either room-mounted [3, 4] or built into the treatment machine. The real-time images can be compared with DRRs, which represent the ideal patient position, and be adjusted accordingly. It has a high imaging quality, but the imaging frequency is limited due to the potentially excessive radiation exposure.
3.
CT systems in various forms, including in-the-vault conventional CT [5], MV or KV cone beam CT [6, 7], and the onboard MV fan beam CT in TomoTherapy [8]. CT images are taken right before the treatment and the target position can then be adjusted. KV CT has better image quality, but the MV CT has its advantages. With MV CT it is less susceptible to the artifacts of metal objects such as the fiducials; there is no need for an additional KV source, and its CT values are reliable for accurate calculation of dose distribution.
4.
When the 2D radiography and CT localization systems (items 1–3) are used, implanted metal (gold or stainless steel) markers can be very helpful for improved visualization and localization of soft tissue target. The markers are usually implanted into the target area a few weeks before simulation, allowing time for the stabilization of the markers position. During treatment, the markers’ on-line position can be precisely compared with those in the simulated image. With this information the body can be repositioned or the beam targeting can be adjusted accordingly.
5.
There are several inventions for 3D real-time fiducial localization during treatment with sub-millimeter accuracy. A radiofrequency-based tracking system has been developed by Varian—Calypso Technology (San Francisco, CA) [9, 10]. Small (1.8 × 8.6 mm) electromagnetic transponders (beacons) implanted into the tumor emit a unique resonant frequency signal when excited by an external electromagnetic field. The magnetic array, which is the source of the electromagnetic field, also receives the frequency signal and determines the transponders’ relative positions. A somewhat similar system, the PayPilot® by Micropos Medical (Gorthenburg, Sweden), uses an active radiofrequency emitter, which has a cable connected to outside radiofrequency source. It is suitable for prostate treatment.
Another device, RealTrackTM technology by Navotek (Yokneam, Israel), uses radioactive sources emitting photons that are detected by an array of detectors located outside the patient’s body.
The advantage of these inventions is that they allow a continuous localization without the KV or MV ionization exposure. A potential disadvantage is that it may not be easily identifiable if the fiducials shifted between CT simulation and treatment, thus resulting in mistreatment.
6.
Ultrasound devices are used for localizing soft tissue structures. With these devices the probe’s position can be tracked, for instance, by an infrared camera system, thus the soft tissue found by the probe can be located in reference to the isocenter [11]. Accordingly, the couch position can be adjusted before treatment. Currently, there are several US vendors marketing products for assistance with SBRT: SonArray (Zmed, Ashland, MA), ExacTrac Ultrasound Localization (BrainLAB, Heimstetten, Germany), and the BAT® (B-mode Acquisition and Targeting) ultrasound system (Nomos, Sewickley, PA).
7.
Low-field open Magnetic Resonance Imaging (MRI) -based systems were first developed for real-time monitoring during surgery. Since MRI provides superior soft-tissue contrast without ionizing radiation, it is ideal for real-time volumetric monitoring of soft-tissue targets in SBRT. Prototype of integration of MRI and linac has been developed [12, 13]. In these systems it is necessary to avoid the interference of the MR magnetic field with the trajectory of the electrons in the linac waveguide, and to avoid the interference of the radiofrequency (RF) signals from each system with the operation of others. From that point of view, it seems advantageous in integration of MRI with a cobalt device. The Renaissance System is based on the technique and is currently being developed by ViewRay, Inc. (Oakwood Village, OH).
8.
Optical systems use two or more cameras to relay positional information. Various wavelengths are used, including ultraviolet, visible, and infrared light. Light reflectors or emitters attached to the body may be used, supplying continuous external body information. A laser-based optical surface scanning system, C-RAD SentinelTM (Uppsala, Sweden), is able to monitor the patient motion during treatment and assist in repositioning.
With the target’s 3D position determined, either the patient position or the beam position/direction can be adjusted as desired if the target is well-immobilized. For moving targets, such as targets with respiratory motion (lung and upper-abdominal tumors), the next step, image-guided tracking, becomes necessary. Significant effort has been devoted to solving the problem [14]. Various techniques have been developed in the past, including:
Gating techniques using either respiratory gating during free-breathing or breath holding [15–19]. With these techniques, the treatment beam is fixed in space and gated to turn on only when the target, fiducial marker, or other surrogate signal (such as an optical system) moves into the preplanned area. Gating is required in both CT simulation and treatment with either external respiratory signals, such as the Varian Real-time Position Management TM (RPM) system, or using internal fiducial markers tracked by a pair of stereotactic KV X-ray imaging systems [20]. In this approach the treatment beam follows the breath phase but not the absolute position of the target during treatment. As a result of this inherent inaccuracy these techniques still need a substantial safety margin.
Targeting based on four-dimensional (4D) respiration-correlated CT. Ten or more sets of CT data are reconstructed at each respiratory phase. The patient-specific treatment volumes are determined using extreme tumor positions based on the 4D CT. Treatment planning margins from the gross tumor volume to the planning target volume (PTV) can be reduced substantially on a composite gross tumor volume derived from 4D-CT (known as internal target volume—ITV) [21, 22]. This 4D CT method may also combine with the gating technique, thus leading to a smaller margin. The drawback of this method is that the patient may receive substantial dose from the CT and the number of CT slices generated may well above 1,000.
The above two techniques, however, may not be good enough for SBRT in certain locations. Real-time dynamic tumor-tracking methods or respiratory-synchronized techniques offer a much improved solution. Currently this technique has only been realized by SynchronyTM Respiratory Tracking system (Accuray, Sunnyvale, CA). In this system, the treatment plan is based on a static CT scan, usually in the end-expiration phase. The accelerator is robotically controlled, which allows for non-isocentric beam delivery within a solid angle of over 2π. In order to compensate for the time delay between data acquisition and accelerator repositioning, the tumor’s real-time position is predicted by the correlation model of an optical monitor system (with three optical light-emitting markers fitted on a tight vest worn by the patient) and a pair of orthogonal X-ray images which locate the internal gold fiducials implanted inside the tumor. The external marker position is continuously measured using the optical system and the X-ray images are taken every 3–5 beams (or in newer systems according to how much time has elapsed since the last image, a flexible user-defined variable). The patient can breathe freely; the beam is delivered by a linear accelerator mounted on a robotic arm which follows the corresponding model. This model is checked and updated by acquisition of new pairs of X-ray images. With this tracking system, the tumor’s translational movement can be largely compensated, but not for the tumor rotation and body deformation. As a result, a margin of up to 5 mm may be required in selected cases [23]. An improved tracking mode, X-sight Lung by Accuray, allows the dynamic tracking to be achieved based on the lung tumor radiograph images without using fiducials for certain lung cases (about 30 % patients in some centers).
Besides using the robotic controlled linac, the dynamic tumor-tracking can be achieved by a technique currently being developed by Vero, in which a linac is mounted on gimbals that allows tilting of the linac following a moving target. Conceptually, this tracking may also be achieved by dynamic moving MLCs in a conventional linac [24–31] based on the MV EPID or KV imaging. This approach, however, is still in a development stage.
3.2.3 Simulation and Other Imaging Modalities
CT is a primary imaging modality for SBRT. Since CT provides geometric information with minimal spatial distortion (less than 1 mm in 20 cm as the general QA requirement) and provides the tissue density needed for dose calculation, it is the basis for treatment planning. CT images are helpful in identifying pulmonary nodules, parenchymal diseases, and chest wall involvement for superior sulcus tumors and lung diseases [32, 33]. Contrast-enhanced CT is the most sensitive study for the hepatic system [34]. A typical scan length should extend at least 5–10 cm superior and inferior beyond the treatment area; for non-coplanar systems this length should be extended to 15 cm. Tomographic slice thicknesses of 1–3 mm are required.
MR and 18F-fluorodeocyglucose (18FDG) PET are frequently used. As the gold standard for visualization of brain neoplasms, MR also found increasing applications in prostate, spinal tumors, and chest and solid abdominal tumors. PET (Positron Emission Tomography) is valuable in staging, planning and evaluating treatment response and might predict long-term outcome [35]. PET-CT is now popular for reduced image registration uncertainties and widely used for lung cancer, head-and-neck tumors, colon cancer, etc. Whenever possible, the initial patient setup (along with any immobilization device) during CT simulation should be reproduced during other imaging modalities, which helps to significantly reduce the uncertainty when it is fused with the CT imaging.
3.2.4 Beam Characteristics
Among the photon beam characteristics, the beam penetration power and the beam penumbra are important factors to achieve the following dosimetry goals:
For small narrow beams used in SBRT, the higher the beam energy, the larger the beam penumbra due to lateral electron transport in medium. In a low-density medium, such as lung tissue, this effect becomes more significant. For SBRT lung application a 6 MV photon beam provides a reasonable compromise between beam penetration and penumbra characteristics [36].
A sharp penumbra is required for a rapid dose fall-off. Inherited from SRS, the cone collimators are naturally a good choice (e.g., they are used in CyberKnife). The IrisTM Collimator in CyberKnife, is adjustable (cone size from 5 to 60 mm) with sub-millimeter accuracy. It has a similar beam profile as the fixed cone and increases the dose delivery efficiency. Another alternative is the multi-leaf collimator (MLC). A study compared a 3 mm micro MLC (Brianlab), a 5 mm MLC, and a 10 mm MLC (Varian Millennium) using the Eclipse treatment-planning system. It was found that the narrower leaf-width MLC provided slightly better results than the wider leaf-width MLCs in an overall dosimetric comparison. This advantage decreases as the target volume increases [37]. In practice, the 3 mm MLCs are more demanding in maintenance. Thus, the 5 mm MLC seems favorable in many SBRT applications.
A new development is the photon beam generated without usage of the flattening filter (Varian TrueBeamTM, Varian Medical System, Palo Alto, CA) [38, 39]. When flattening filter-free (FFF) beams are used, out-of-field doses can be reduced significantly. This is mainly due to reduced head scatter and residual electron contamination. FFF beams should therefore lead to reduced peripheral doses and patients may benefit from decreased exposure of normal tissue to scattered doses outside the field. Removal of the flattening filter implies also the possibility to deliver treatments with higher dose rates, up to a factor of 4 at 10 MV, and with a much higher dose per pulse. Besides further improving time efficiency for delivery, this might have subsequent potential radiobiology implications. Whilst interest for FFF beams is increasing in the medical physics field, there are few instances where FFF beams are applied in clinical practice, particularly in SBRT treatments. Initial clinical outcomes for local control are promising. However, aspects related to standardization, dosimetry, treatment planning, and optimization need to be addressed in more detail in order to facilitate the clinical implementation [38, 39].
Tomotherapy is a new concept for beam delivery [8]. A small megavoltage X-ray source was mounted on a ring gantry in a similar fashion to a CT x-ray source, the patient moves through the bore of the gantry simultaneously with gantry rotation. The beam intensity is modulated by temporally modulating multiple independent leaves that open and close across the slit opening. The geometry provided the opportunity to provide CT images of the body in the treatment setup position. The incident electron beam with 6 and 3.5 MeV in the treatment and imaging modes, respectively.
Cobalt-60 with MLC is also a suitable beam source. It has been shown that the common assumption that the cobalt penumbra is inferior to linac penumbra for MLC-based IMRT is not supported by comparative analysis [40]. Nearly identical plans can be achieved using cobalt source when compared to 6 MV IMRT.
Proton beams are being investigated for SBRT in certain sites. Dosimeric studies for using proton beam in SBRT have been performed by comparison between photon and proton-based SBRT for liver and lung treatment [41, 42]. It indicates that protons resulted in lower doses to critical organs at risk and a smaller volume of non-targeted normal lung exposed to radiation. However, the clinical significance and relevance of these dosimetric improvements remain unknown at the moment.
3.2.5 Planning
The terminology defined for conventional radiation therapy by ICRU 50 and ICRU 62 [43, 44], such as GTV, CTV, PTV, and ITV, is still used in SBRT. In many cases, especially for the metastatic lung, liver, and paraspinal cases, the GTV and CTV are often identical [45–47].
Due to the inevitable movement in most of the extracranial targets, SBRT is inherently imprecise compared to SRS and SRT, unless adequate immobilization or compensation for the target motions, both inter- and intra-fractional, is achieved. Thus dosimetric benefits of targeting essentially rely on the appropriate utilization of immobilization devices and/or on the techniques for precise organ motion compensation [23, 48–50]. The uncertainty in the CTV is generally accounted for by an internal margin added to the CTV, resulting in the internal target volume (ITV) [44]. The PTV then addresses all other possible geometrical variations, including setup uncertainties, machine tolerances, and intra-treatment variations, by adding all these margins to the CTV. This margin should be determined based on the particular SBRT system, the target site, and other clinical conditions.
The main objectives for high-dose-per-fraction SBRT are the precise and conformal targeting and rapid dose fall off to normal surrounding tissues. These objectives can be spelled out in the following dosimetric considerations during treatment planning:
1.
The shape of the iso-surface defined by the prescribed dose should conform to the outline of the target. Planning systems providing iso-lines in each CT image, and the DVH (dose-volume histogram) are good tools for evaluating the quality of proposed treatment planning. The Conformity Index is a complementary indicator, which attributes a score to a treatment plan. It can be used to compare several treatment plans for the same patient [51].
2.
Rapid fall off of dose from the tumor volume to healthy tissue in all directions, provided the OARs (organs at risk, i.e. serially functioning organs such as the spinal cord or sensitive mucosa) are sufficiently spaced from the target [52]. Otherwise, particular attention has to be paid to the direction of the adjacent critical organs.
These first two objectives can be achieved primarily by using a large number of preferably non-coplanar beams from as many directions as possible in the treatment.
3.
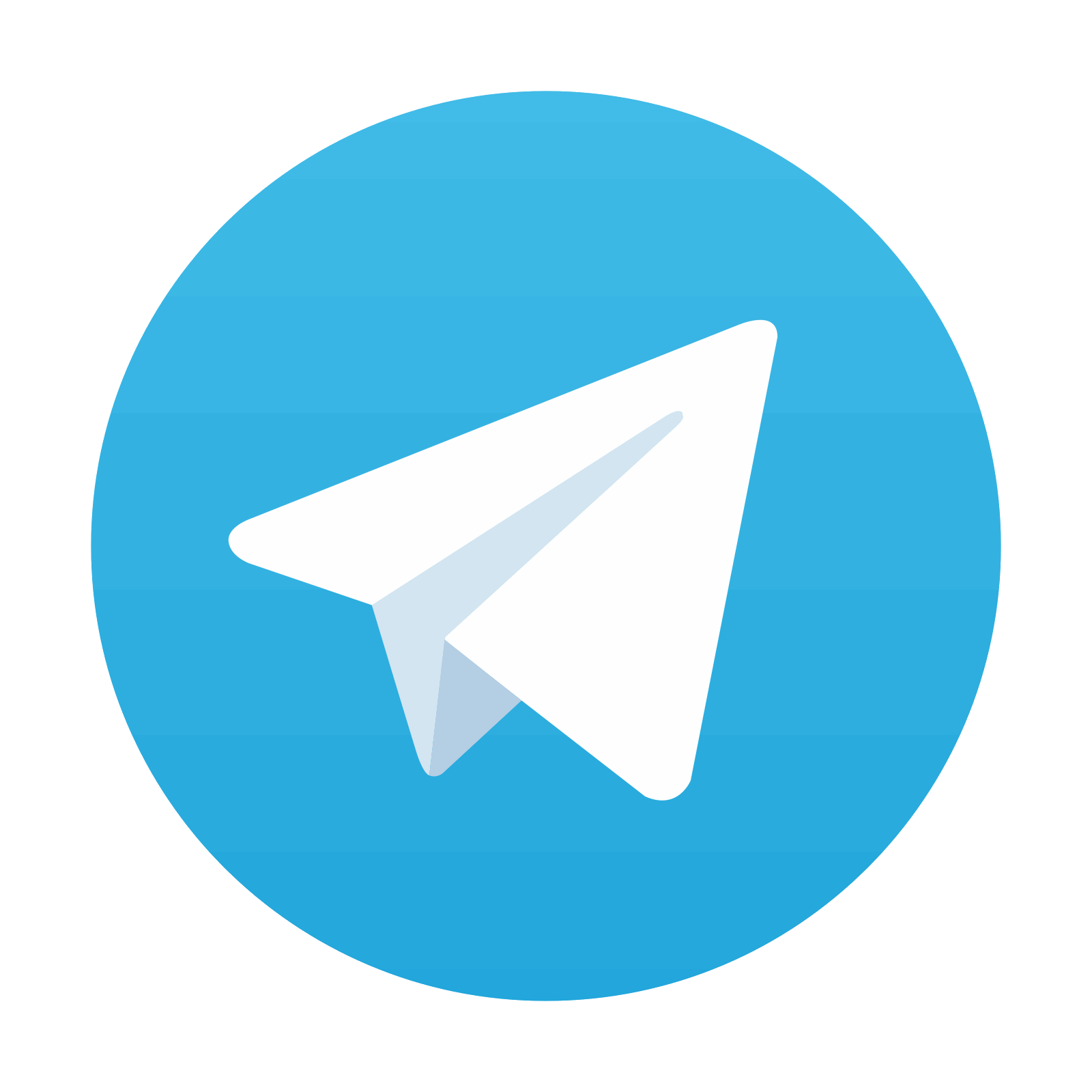
Dose heterogeneity: distribution throughout the volume of the tumor, with the highest dose delivered to the central portion of the tumor, or to a site-specifically defined area. Unlike in conventional radiation therapy where the goal is to deliver uniformly distributed doses to the whole target, in SBRT hot spots (e.g., 130 % of the prescribed dose) within the target volumes are common and generally viewed to be clinically desirable, as long as there is no spillage into normal tissue. It has been hypothesized that hot spots within the central region offer a special advantage in eradicating radio-resistant hypoxic cells that are more likely to be located therein [53].
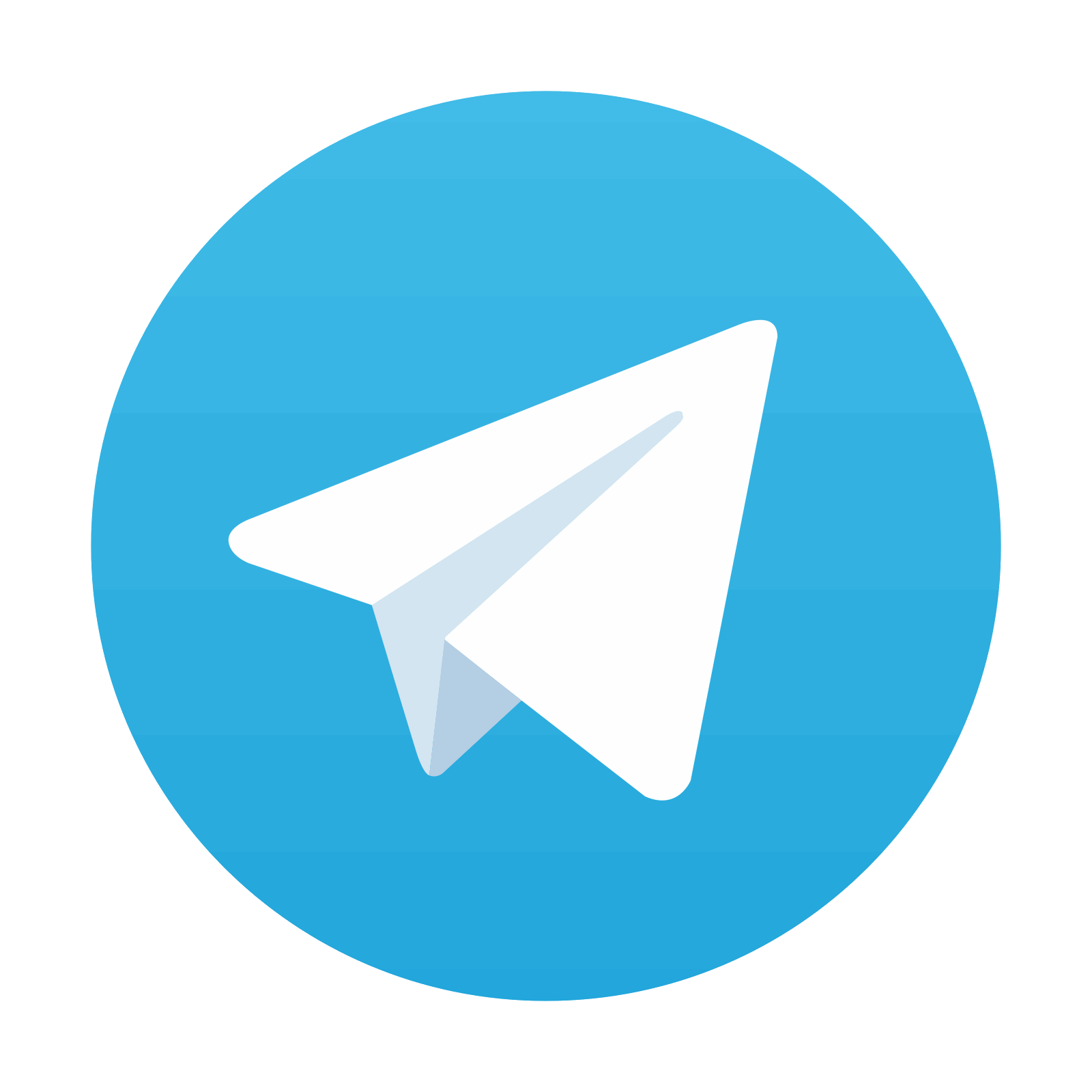
Stay updated, free articles. Join our Telegram channel

Full access? Get Clinical Tree
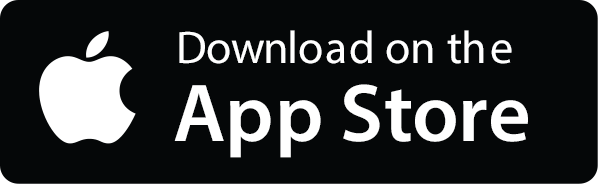
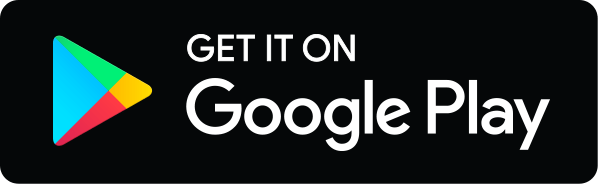
