5 Arteriovenous malformations (AVMs) are congenital vascular anomalies comprised of an abnormal number of blood vessels that are abnormally constructed. The blood vessels directly shunt blood from arterial input to the venous system without an intervening capillary network to dampen pressure. Both abnormal blood vessel construction and abnormal blood flow lead to a risk of rupture and intracranial hemorrhage. In addition, patients with lobar vascular malformations may suffer from intractable vascular headaches or develop seizure disorders. The annual incidence of AVM recognition is thought to be ~10,000 patients per year in the United States. However, the reliance on magnetic resonance imaging (MRI) has led to an increasing recognition of these vascular anomalies even in patients with minimal symptoms. The decision making relative to management of an AVM must be carefully evaluated based on several risk factors. The options for management include observation, endovascular embolization alone or in preparation for other adjuvant management, craniotomy and surgical removal, and stereotactic radiosurgery (SRS).1 All treatments may be done in one or more stages. In general, the following factors are evaluated when a patient is seen with an AVM: the patient’s age, associated medical condition, history of a prior hemorrhagic event, prior management if any, overall volume and morphology, location of the AVM, initial presenting symptoms (headache, seizures, and local neurologic deficits), the AVM angioarchitecture (e.g., compact vs diffuse nidus), estimation of its surgical risks, presence of a proximal or intranidal aneurysm, and prior surgical experience in training. In making a decision for management strategies, we often employ a decision tree algorithm as shown in Fig. 5.1. Optimal care depends on careful weighing of each of the above factors and the estimated risk of subsequent hemorrhage. The patient’s clinical presentation and location are important issues as well as symptoms in each patient. Age, prior bleeding event, smaller AVM size, deep venous drainage, and high flow rates have been suggested by some as increasing the potential for subsequent bleeding. For larger volume AVM (average diameter 4–5 cm), observation may be the only reasonable strategy in view of the risks of even multimodality management.2 This may be especially true for patients who have never bled previously. Endovascular embolization employing a variety of particulate, glue, or coil methods may be used as an adjunct prior to craniotomy and surgical removal.3,4 It has also been performed in preparation for SRS, although its role prior to radiosurgery has declined with the realization that embolization rarely leads to significant volumetric reduction. Although the flow within the AVM may change after embolization, SRS must include the original volume. In contrast, before surgical removal, embolization may provide major benefit, either by reducing flow or eliminating deep-seated feeders that would otherwise be a significant problem during AVM resection. Recanalization of embolized AVM components over time may require repeat SRS. Surgical removal is an important option for patients with lobar vascular malformations of suitable size, especially at centers of excellence with extensive AVM experience. Incomplete removal requires adjuvant management, perhaps including radiosurgery. Spetzler and Martin, among others, defined the relationship of AVM volume, pattern of venous drainage, and location within critical areas of the brain as important considerations that help to facilitate outcome prediction at the time of surgical resection at centers of excellence. Outcomes after AVM radiosurgery do not correlate with the same predictions of the Spetzler–Martin scale when microsurgery is used.5 Outcomes after radiosurgery may be predicted based on volume, location, age, angioarchitecture, and dose delivered.6 SRS is an excellent management strategy for patients with AVMs < 30 mm in average diameter (for a single procedure). Staged procedures are used for larger vascular malformations or for those that were incompletely obliterated 3 years or more after an initial procedure. Fig. 5.1 Clinical algorithm for choosing management options for patients with intracranial arteriovenous malformations (AVMs). The chief benefit of radiosurgery management is risk reduction; the chief deficit of radiosurgery is the latency interval that is required to achieve complete obliteration of the AVM.7,8 The latency interval is generally 2 to 3 years, but in selected patients it may be longer. AVM radiosurgery has been used for children not suitable for other management strategies, as well as for older patients who have significant medical risk factors for surgical removal. Radiation to obliterate abnormal blood vessels in the brain is a procedure that was first considered in the late 1960s. Raymond Kjellberg, using the Harvard-affiliated proton facility in Cambridge, Massachusetts, advocated proton Bragg peak stereotactic radiation during the 1970s and early 1980s.9,10 More than 1000 AVM patients were treated, but the dose-planning technique was quite rudimentary. The technology of the Bragg peak proton facility was designed to provide a low exit dose based on the radiophysiological characteristics of this technology. The doses that were actually used in this series of patients were quite low and do not correspond to doses that we now know may be effective in the obliteration of AVMs. Although Kjellberg maintained that Bragg peak radiation stabilized AVM blood vessel walls and reduced the subsequent risk of hemorrhage (in comparison to age-related survivals from a life insurance table), only 20% of patients had complete obliteration of their AVM over time. Fabrikant et al, working at the Lawrence Livermore National Laboratory, began to use helium ion beam to perform multisession AVM irradiation in the 1980s.11 In Stockholm, Lars Leksell and Ladislau Steiner initiated work with the first-generation Leksell Gamma Knife unit.12 The first patient was treated in March 1970, using the original prototype 179 cobalt source photon beam unit designed by Borje Larsson and Lars Leksell. The target definition was based on biplane angiography done during the procedure itself. Patients were observed for a period of time in preparation for a larger experience that began to emerge in Stockholm using the second-generation unit, which was built in 1975. Linear accelerator (linac) technologies have been adapted for SRS. O.O. Betti, working in Paris and Buenos Aires,13 J.L. Barcia-Salorio in Spain,14,15 and Colombo in Vincenza, Italy,16–18 were pioneers in the application of photon radiation using newer generation linacs. In addition, surgeons and radiation oncologists working at the Joint Center in Boston19 and in Gainesville, Florida,20 used modified linacs to treat a large number of vascular malformations. Most centers continue to evaluate SRS as part of an overall management plan that may include embolization, microsurgical removal, or radiosurgery alone or in combination. We will discuss our current viewpoint relative to the role of embolization subsequently. Our first AVM patient was treated in August 1987,21 and we reported our initial experience in 227 patients in 1991.22 Confirming the work of others, we noted that the 2-year success rate in terms of complete obliteration was related to nidus volume and dose. In high-dose cases (AVM < l cc), obliteration rates of 100% were noted, which declined to 85% for volumes of 1 to 4 cc and 58% for AVMs > 4 cc in volume.
Stereotactic Radiosurgery for Arteriovenous Malformations
L. Dade Lunsford, Ajay Niranjan, Hideyuki Kano, John C. Flickinger, and Douglas Kondziolka
History of Radiosurgery for Arteriovenous Malformations
Radiosurgery for Arteriovenous Malformations at the University of Pittsburgh
History
Number of patients | 906 |
Patient age | |
Median | 36 years |
Range | 3–80 years |
Gender | |
Male | 474 (52%) |
Female | 432 (48%) |
Presenting symptoms | |
Hemorrhage | 417 (46%) |
Seizures | 213 (24%) |
Headache | 164 (18%) |
Sensory motor deficit | 74 (8%) |
Incidental | 38 (4%) |
Prior management | |
Embolization | 194 (21%) |
Surgery | 63 (7%) |
Our subsequent 20-year experience in Pittsburgh has now increased to more than 1100 patients who have undergone Gamma Knife radiosurgery for their AVM using one or more radiosurgical procedures. We have more recently analyzed the outcome data of 906 patients who underwent radiosurgery between 1987 and 2004 (Tables 5.1, 5.2, and 5.3). Our median patient age was 36 years, with a range of 3 to 80 years. Typical symptoms at presentation included hemorrhage (46%), seizures (24%), and headache (18%). Eight percent had neurologic deficits (8%). An incidental AVM was detected in 4% of patients. Prior management strategies included surgical removal or clot evaluation in 7%. Twenty-one percent underwent one or more interventional procedures (embolization). The median target volume was 3.4 cc (range 0.065–57.7 cc). The median margin dose was 20 Gy (range 13–32 Gy). A single procedure was performed in 865 (95.5%) of patients, and repeat radiosurgery for incomplete nidus obliteration after 3 years was needed in 113 (12.5%) patients. Prospective volume staged radiosurgery was performed in 41 (4.5%) patients.
AVM locations | Percentage |
Temporal | 18.50 |
Frontal | 18 |
Parietal | 17.50 |
Thalamus/basal ganglia | 16.0 |
Occipital | 11.50 |
Cerebellar | 6.30 |
Brainstem | 5.50 |
Dural | 2.70 |
Corpus callosal | 2.0 |
Intraventricular | 1.0 |
Pineal | 1.0 |
Spetzler–Martin grade | |
Grade I | 2.1 |
Grade II | 24.4 |
Grade III | 42.4 |
Grade IV | 15.0 |
Grade V | 2.7 |
Grade VI | 13.4 |
Coexistence of aneurysm | 77 (8.5) |
AVM volume | |
Median | 3.4 mL |
Range | 0.065–57.7 mL |
Radiosurgery dose | |
Median | 20 Gy |
Range | 13–32 Gy |
Radiosurgery | |
Single session | 865/906 (95.5%) |
Prospective volume staged | 41/906 (4.5%) |
Repeat radiosurgery | 113/906 (12.5%) |
At a median follow-up of 3 years, complete nidus obliteration was achieved in 78% (confirmed by angiography or MRI). In addition, 21% of patients achieved subtotal obliteration of the nidus. During the follow-up interval, 38 bleeds (4.1%) occurred after the procedure. Seizure control was improved in 51% of patients who presented with seizures. Adverse radiation effects (AREs) resulting in neurologic deficits developed in 24 patients (2.6%) and the detection of new T2 signal increase surrounding the AVM target in 108 patients (12%). We noted long-term complications such as delayed cyst formation and encephalomalacia in 16 patients (1.7%). No patient in this AVM series has developed a radiation-related tumor at the time of this writing.
Stereotactic Radiosurgical Procedure
We perform intracranial radiosurgery using the Leksell Gamma Knife (Elekta Instruments AB, Stockholm, Sweden), beginning with the model unit U, and proceeding to the B, C, 4-C, and in recent experience Perfexion model. The patient’s clinical studies and imaging are reviewed for suitability for SRS. As noted previously, we evaluate the bleeding history, the age of the patient, existing comorbidities, location, and clinical symptomatology. Patients with lobar AVMs were placed prophylactically on anticonvulsants for a period of 2 to 4 weeks around the time of the procedure. This has reduced the risk of a perioperative seizure event from as high as 5% in year 1 of our 20-year experience to a risk of < 1% at the current time. We require that all women within the child-bearing age have a recent negative pregnancy test, or we perform it on the day of the procedure.
Patients are evaluated preoperatively by the neurosurgeon, the radiation oncologist, and the nursing team. On the morning of the procedure, the patients arrive at 6 o’clock and begin conscious sedation using oral lorazepam followed by intravenous (IV) conscious sedation (fentanyl and midazolam) as needed. Scalp anesthetic injection using a combination of Marcaine and Xylocaine is injected at the sites of pin application. At the current time, we prefer to use titanium pins with plastic inserts, to minimize the risk of MRI artifacts. General anesthesia may be required for frame application in imaging in patients younger than 12 years of age.
Neurodiagnostic imaging follows using both IV paramagnetic contrast-enhanced three-dimensional (3D) volumetric MRI scan and a whole-head T2 fast spin echo imaging sequence. Patients subsequently undergo biplane digital subtraction angiography (DSA) as the next step. In patients where MRI scans are not possible (those with undetermined implants, pacemakers, old aneurysm clips, etc.), we use contrast-enhanced stereotactic computed tomography angiography (CTA). The development of axial imaging as part of the imaging paradigm is critical for AVM management. This has allowed us to make superior 3D conformal plans much more carefully in comparison to using two-plane angiography alone.
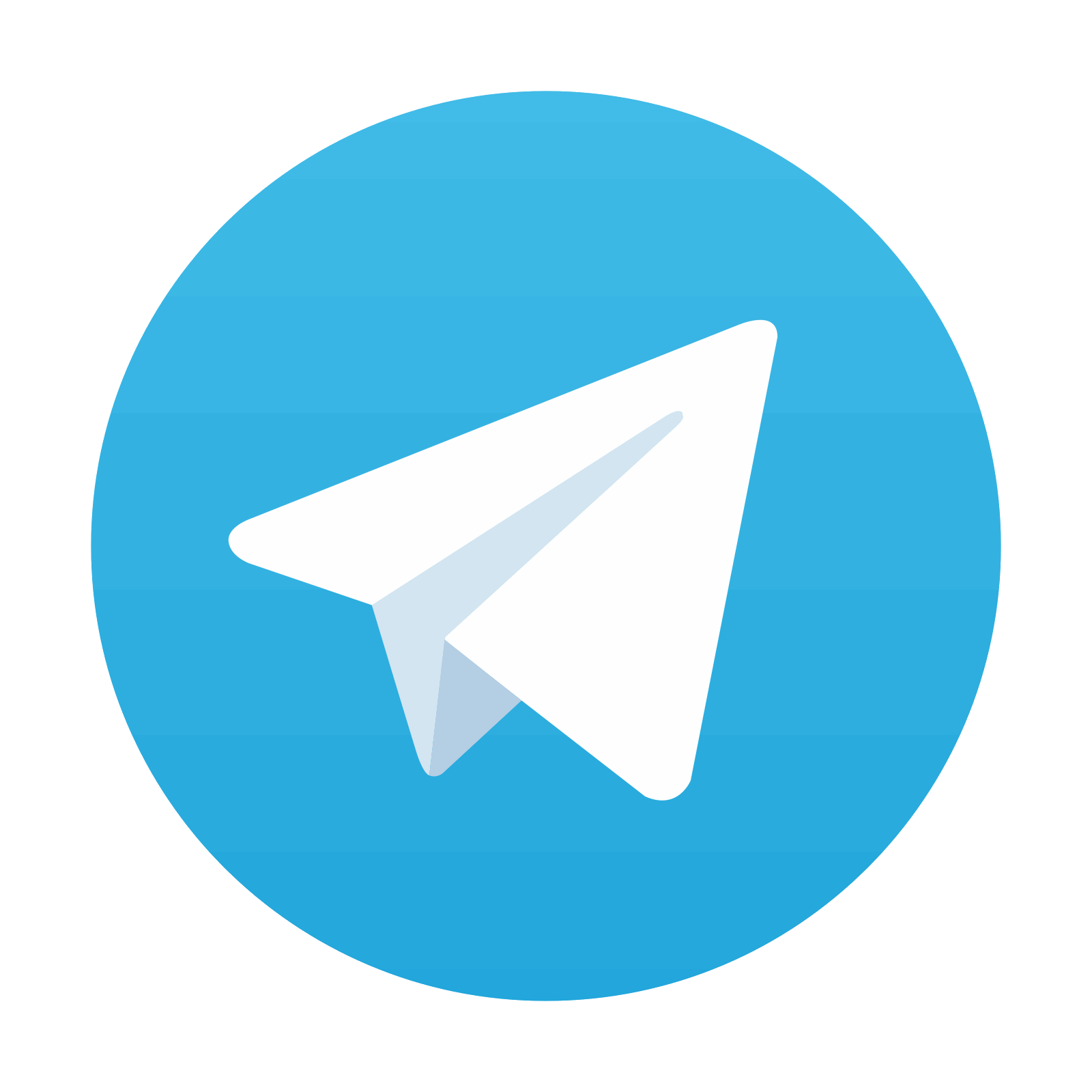
Stay updated, free articles. Join our Telegram channel

Full access? Get Clinical Tree
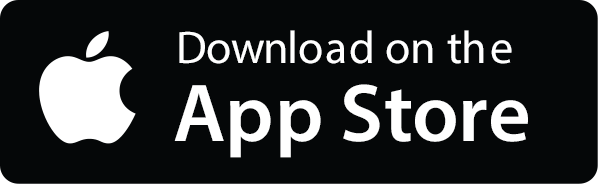
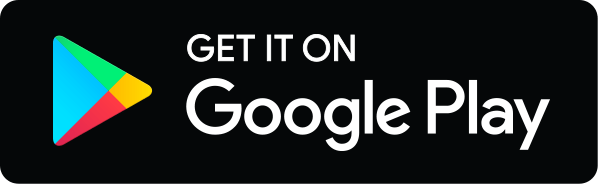