19 Brain metastases are a significant cause of mortality and morbidity, with an annual incidence of ~170,000.1 Most commonly, these lesions originate from primary tumors of the lung, breast, kidney, skin (especially melanomas), and colon. An estimated 20 to 30% of patients with cancer are at risk of developing metastases to the brain,2 with ~40% of patients presenting with a single lesion.3 The prognosis for these patients remains poor despite advances in surgical technique, chemotherapy, and radiation therapy, with those receiving no form of therapy having a median survival of ~1 month. Based on historical studies, it has been determined that medical treatment with steroids alone yields a median survival of 1 to 2 months, whereas the addition of whole brain radiation therapy (WBRT) affords a median survival of 4 to 7 months.4–8 Undoubtedly, brain metastasis is one of the most feared developments for a cancer patient, and hence represents a serious and often difficult therapeutic challenge for both neurosurgeons and oncologists. The treatment of brain metastases historically has included WBRT, a therapeutic modality first reported in the early 1950s.9,10 The Radiation Therapy Oncology Group (RTOG) conducted numerous trials from 1971 to 1993 to investigate various doses of WBRT and their modalities of delivery.11–19 Additionally, the RTOG investigated the use of radiation sensitizers, such as bromodeoxyuridine (a synthetic nucleoside that is the analogue of thymidine) and hyperfractionation, and found no overall improvement in survival. From the outcome of these studies, it was determined that the use of 3000 Gy in 10 fractions was an acceptable regimen. However, although neurologic symptoms improved in the majority of patients, local control rates were low, and neurologic death still occurred in 25 to 54% of patients.11 To offset the poor prognosis in patients with brain metastases, alternative techniques to WBRT were simultaneously sought. Described by Lars Leksell in 1951, stereotactic radiosurgery (SRS) employs the use of a single high dose of radiation directed by stereotaxis to the target with the use of narrow radiation beams to spare the normal surrounding brain tissue.20 Since the initial report of 12 patients treated by a modified linear accelerator (LINAC) in 1987,21 numerous studies have supported the benefit of SRS therapy in newly diagnosed as well as recurrent brain metastases. Today, brain metastases represent the most common indication for SRS. Table 19.1 shows a comparison of studies for surgery versus WBRT versus SRS in categories of study design, average dose, and rates of local control, local recurrence, and median overall survival. The prognostic scale most commonly used for patients with brain metastases is the RTOG recursive partitioning analysis (RPA), as described by Gaspar et al in 1997.22 Based on data obtained from 1200 consecutive patients enrolled in three RTOG trials from 1979 to 1993, the RPA scale identified three major variables predictive of outcome: patient age ≥65 years, functional independence as defined by a Karnof-sky Performance Scale (KPS) score ≥70, and uncontrolled compared with controlled extracranial disease. Patients were then stratified into class 1, 2, or 3 according to these variables. Table 19.2 lists the various classes, their components, and their mean survival time. The single most important predictor of outcome was functional status; patients with KPS scores <70 had the worst prognosis, whereas young, functionally independent patients with controlled extracranial disease had the best prognosis. Corroborating this, a study by Lagerwaard et al reviewed 1292 patients with brain metastases and found the most important factors to be KPS, treatment modality, extracranial disease, and response to steroid treatment.23 Although RPA class is the most significant predictor of survival, aggressive local resection for metastatic foci in addition to WBRT improves survival in selected patients.24–26 Additionally, surgery can establish or confirm the diagnosis while offering immediate and effective palliation of symptomatic mass effect as well as the perceived premise of improved survival (Fig. 19.1). In Europe, Noordijk et al’s study included 63 patients with computed tomography (CT)–confirmed single metastases who were randomized to surgery with WBRT versus WBRT alone.24 Surgery with WBRT improved survival (10 vs 6 months; p = .04) and functional independence (7.5 vs 3.5 months; p = .06) more than WBRT alone. There was no surgical benefit for patients with active extracranial disease. Similarly, Patchell et al’s study at a single center in the United States randomly assigned 48 patients with single brain metastases to two treatment groups and demonstrated that surgery with WBRT improved survival (40 vs 15 weeks; p <.01), local control (80% vs 48%; p <.02), and functional independence (38 vs 8 weeks; p <.005) compared with that of biopsy and WBRT alone.25 A third trial by Mintz et al included 83 patients but did not demonstrate a survival benefit for surgical patients (6.3 vs 5.6 months; p = .24).26 The lack of benefit in this study has been attributed to the influence of RPA class on survival where patients with lower KPS scores were included and the fact that the study consisted of a large percentage of patients with active extracranial disease. We recommend surgical resection of brain metastases >3.5 cm when in a surgically accessible location. Smaller tumors that cause significant mass effect or edema may also benefit from surgery over radiation therapy, particularly when the patient has neurologic deficit. The use of multiple craniotomies for multiple metastases remains controversial, but they may be indicated in good performance patients with large, symptomatic tumors. In general, WBRT follows every surgical resection. The success of aggressive resection for control of a single metastasis has allowed neurosurgeons to use a complementary approach with SRS for control of single and multiple brain metastases. Indeed, in a relatively short time, SRS has emerged as one of the most important noninvasive options in the armamentarium against brain metastases. Brain metastases are generally discrete, pseudo- or semi-spherical in shape, located in the gray-white junction, have a maximum diameter <4 cm, and, unlike primary gliomas, are noninfiltrative. These features lend them the characteristics that allow for accurate target localization, planning, and treatment delivery. Importantly, a single large fraction of radiation appears to have a similar effect irrespective of the tumor type.27–31 Nieder et al reviewed the images of 332 patients with brain metastases to evaluate local control and time to local progression based on dose.32 Total doses of 25 to 60 Gy were administered (single doses 1.8–5.0 Gy). Biologically effective doses were derived for each patient, from which it was determined that higher doses were needed to improve local control. Brain metastases are considered “category IV” targets, which are targets with early-responding tissue (tumor) surrounded by late-responding normal tissue (brain).33 Because the biologically effective dose in early-responding tissue is smaller than an equivalent dose in late-responding tissue, a higher single dose is needed in brain metastases than in benign tumors. SRS allows a high central dose to be given, yet has a rapid drop-off in dose that protects the surrounding brain tissue. As determined from the Noordijk et al24 and Patchell et al25 studies comparing surgery with WBRT versus WBRT alone, local tumor control led to improved survival. These results combined with the potential advantages of SRS led to an increased interest in the use of SRS as an alternative to surgery for local tumor control. An SRS radiation dose is described as energy per mass and is expressed in units of gray delivered to the prescribed isodose line. The prescription isodose line reflects the minimum amount of radiation delivered to every tumor cell within its area and in most cases is the isodose line that most closely approximates the outline of the tumor. This line is most conveniently described as a percentage of the maximum or central dose delivered to the tumor (Fig. 19.2). For example, if 20 Gy is given to the 50% isodose, then the center of the tumor receives 40 Gy. Obviously, the goal of SRS is to include the entire gross tumor volume (GTV) within the prescription isodose volume (PIV) with as little overlap to the normal brain as possible. The ratio PIV/GTV is one description of the treatment plan’s conformality. Ideally, the PIV/GTV ratio is <2, which implies that there is less normal tissue than tumor tissue irradiated in the prescription volume. In addition to being conformal, the treatment plan should ensure that at least 95% of the GTV is covered by the PIV. These relationships can be determined from an examination of the dose–volume histograms (DVHs) that should be generated with each treatment plan. Modern radiosurgery systems can accurately contour the isodose line for small tumors, but if larger tumors are to be treated, this presents a difficulty, as the amount of radiation delivered needs to be increased to allow for equivalent local control. Consequently, the radiation exposure of normal tissue is increased, and therefore treatment of large tumors is performed using the process of hypofractionation, where a lower radiation dosage is delivered over multiple fractions (generally three to five fractions) to prevent radiation toxicity.34 Fig. 19.2 A right temporal metastasis is seen with superimposed isodose curves from a stereotactic radiosurgery treatment plan. The 30, 40, and 50% lines are seen. In this case, the 50% line encompasses the prescription isodose volume. To determine the maximum tolerated doses of single-fraction radiosurgery, the RTOG conducted dose escalation studies that were reported from the RTOG 9005 trial.34 Although the study included patients with primary gliomas and brain metastases, the primary stratification variable was size. From this study it was determined that the maximum tolerated doses were 24, 18, and 15 Gy for tumors ≤20 mm, 21 to 30 mm, and 31 to 40 mm in maximum diameter, respectively. However, for tumors <20 mm, the investigators’ reluctance to escalate to 27 Gy, rather than excessive toxicity, determined the maximum tolerated dose. This study provided the guideline and framework for dosing, although it failed to account for other variables, such as location. For example, the cranial nerves and brainstem are more sensitive to radiation than are other regions of the brain parenchyma; consequently, dose prescription must be reduced as necessary to protect these structures. On the basis of institutional experience at the University of Kentucky and guided by the results from the RTOG 9005 trial, Shehata et al35 conducted a study to determine the optimal SRS dose and influence of WBRT on local tumor control among metastases ≤2 cm in size. Between 1992 and 2001, 160 patients (468 metastases) were treated with SRS (dose range 7–30 Gy; median 20). A total of 240 metastases received planned WBRT (range 6.75–50.4 Gy; median 40.5) versus 228 metastases that did not. The variables tested included histological type, site of metastasis, primary diagnosis, tumor volume, SRS dose, newly diagnosed versus recurrent metastasis, and planned WBRT versus no planned WBRT, with the addition of WBRT alone being the most significant predictor of local tumor control. Overall, patients who received WBRT had superior local tumor control rates (97% vs 87% in those who did not receive WBRT; p = .0001). Additionally, patients receiving WBRT and SRS ≥20 Gy achieved local control rates of 99% compared with 91% control rates when treated with WBRT and SRS <20 Gy (p = .0029), allowing the investigators to conclude that optimal control of brain metastasis ≤2 cm was seen with 20 Gy SRS combined with planned WBRT. Finally, increasing the SRS dose to >20 Gy resulted in no improvement in local tumor control, but rather a higher rate of neurotoxicity. The potential advantages of SRS include elimination of general anesthesia, outpatient delivery, minimal risk for bleeding or infection, lower risk of complications, short convalescence time, and, frequently, lower cost.35–37 Additionally, SRS is not limited by the number or location of lesions, coagulopathic state, or other concomitant comorbidities. For these reasons, SRS has become a viable alternative to surgery, although there have been conflicting results from various studies.38–41 In a multi-institutional retrospective outcome and prognostic analysis from the University of Wisconsin at Madison for resectable single brain metastasis, 122 patients with newly diagnosed and potentially resectable single brain metastases were treated by SRS and WBRT.38 In this study, the local control rate was 86%, with a median survival of 56 weeks and 25% of the deaths being related to tumor progression. Based on these results, the investigators concluded that SRS could be used as an alternative to surgery. Another study from the M D Anderson Cancer Center in 1996 compared retrospectively matched patients treated by SRS (n = 13) with those treated by surgery alone (n = 62).39 The median survival was 7.5 months for the SRS group compared with 16.4 months for the surgery group; the local recurrence rate was 21% for the SRS group versus 8% for the surgery group. Based on these results, it was concluded that surgery should be the preferred modality of therapy for patients with surgically accessible lesions. In contrast to the previously described studies, a retrospective Mayo Clinic review comparing SRS with surgical resection in the treatment of solitary brain metastasis found no difference in 1-year survival rates for either group.40 Further, in a retrospective study by Muacevic et al, the outcome of patients who underwent surgery with radiotherapy were compared with those who underwent Gamma Knife (Elekta Instruments AB, Stockholm, Sweden) SRS alone.41 Only patients with a single lesion of ≤3.5 cm were included. For the surgical and radiotherapy group versus SRS group, 1-year survival rates, 1-year local control rates, and 1-year neurologic death rates were found not to be statistically significant. It is plausible that patient selection bias was the primary contributor to the previously described results, as they were retrospectively conducted studies. Indeed, when the Joint Center for Radiation Therapy (JCRT) attempted to enroll patients in a phase III trial comparing surgery with SRS in the 1990s, only six patients were enrolled because of patient or physician preference.42 Currently, it is generally agreed that for lesions >3.5 cm or for those without histological diagnosis, surgical resection should be considered as the primary modality of therapy rather than SRS. Additionally, surgical resection affords a quicker improvement in neurologic function, minimizes chronic steroid use, and alleviates symptoms secondary to mass effect. Fueled by the encouraging results of studies using SRS for recurrent brain metastases,43–45 the use of SRS with WBRT was investigated for newly diagnosed patients under the hypothesis that the combination would be superior for local and regional control of brain metastases. A multi-institutional retrospective study reported by Sanghavi et al from 10 institutions analyzed the outcome for 502 patients with brain metastases treated by SRS with WBRT.46 The patients were stratified using the RTOG RPA method. The overall median survival period was 10.7 months, with a higher KPS and lower RPA class predicting improved survival. The addition of SRS boosted results in median survival (16.1, 10.3, and 8.7 months for classes 1, 2, and 3, respectively) compared with the median survival (7.1, 4.2, and 2.3 months for classes 1, 2, and 3, respectively; p <.05) of patients treated with WBRT alone. To assess the effectiveness of SRS alone or in combination with WBRT compared with surgery and/or WBRT in prolonging survival and improving the quality of life and functional status of patients with brain metastases, a meta-analysis of randomized, controlled trials and concurrent cohort studies was performed in 2006.47 The review identified three randomized, controlled trials and one cohort study. Among patients with multiple metastases, no difference in survival between those treated with SRS plus WBRT versus those treated with WBRT alone was found. However, in patients with one metastasis, a statistically significant difference, favoring those treated with SRS plus WBRT, was observed. Regarding local tumor control at 24 months, rates were significantly higher in the SRS plus WBRT treatment arm, regardless of the number of metastases. The investigators concluded that adding SRS to WBRT not only improved survival in patients with one brain metastasis, but also improved local tumor control and functional independence. The largest and most influential study conducted to date in patients with brain metastasis treated with SRS plus WBRT (3750 Gy, 15 fractions) versus WBRT alone comes from the RTOG 9508 trial.48 Reported by Andrews et al in 2004, this multi-institutional randomized clinical trial comprised 333 patients from 55 participating RTOG institutions with one to three newly diagnosed brain metastases on magnetic resonance imaging (MRI), each ≤4 cm in diameter. One hundred sixty-seven patients were assigned WBRT and stereotactic radiosurgery, and 164 were allocated WBRT alone. Univariate analysis showed that there was a survival advantage in the WBRT and SRS group for patients with a single brain metastasis (median survival time 6.5 vs 4.9 months; p = .0393) despite the fact that 19% of the patients failed to receive the intended treatment (31 of 164 patients assigned to the SRS group did not actually undergo SRS, and 28 of 167 patients in the WBRT arm received salvage SRS). Patients in the SRS group were also more likely to have a stable or improved KPS score at 6 months follow-up than were patients allocated to WBRT alone (43% vs 27%, respectively; p = .03). By multivariate analysis, survival improved in patients with an RPA class 1 (p <.0001) or a favorable histological status (p = .0121). Steroid use was also lower in the SRS and WBRT arm, as was local control at 1 year (82% vs 71%; p = .01). The risk of developing a local recurrence was 43% greater with WBRT alone (p
Stereotactic Radiosurgery for Brain Metastases
Mayur Jayarao, Lawrence S. Chin, and William F. Regine
Patient Selection and Prognostic Factors
Results of Surgical Resection
Stereotactic Radiosurgery of Brain Metastases
Dose Characteristics and Tumor Size
Stereotactic Radiosurgery versus Surgery
Stereotactic Radiosurgery and Whole Brain Radiation Therapy
Stay updated, free articles. Join our Telegram channel

Full access? Get Clinical Tree
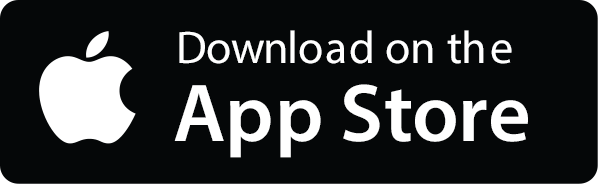
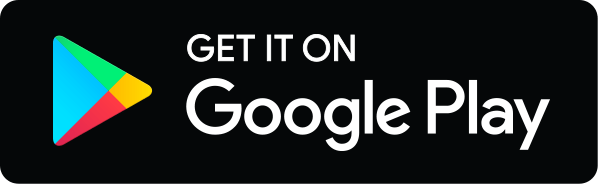